Abstract
Objectives
To assess the influence of material/technique selection (direct vs. CAD/CAM inlays) for large MOD composite adhesive restorations and its effect on the crack propensity and in vitro accelerated fatigue resistance.
Methods
A standardized MOD slot-type tooth preparation was applied to 32 extracted maxillary molars (5 mm depth and 5 mm bucco-palatal width) including immediately sealed dentin for the inlay group. Fifteen teeth were restored with direct composite resin restoration (Miris2) and 17 teeth received milled inlays using Paradigm MZ100 block in the CEREC machine. All inlays were adhesively luted with a light curing composite resin (Filtek Z100). Enamel shrinkage-induced cracks were tracked with photography and transillumination. Cyclic isometric chewing (5 Hz) was simulated, starting with a load of 200 N (5000 cycles), followed by stages of 400, 600, 800, 1000, 1200 and 1400 N at a maximum of 30,000 cycles each. Samples were loaded until fracture or to a maximum of 185,000 cycles.
Results
Teeth restored with the direct technique fractured at an average load of 1213 N and two of them withstood all loading cycles (survival = 13%); with inlays, the survival rate was 100%. Most failures with Miris2 occurred above the CEJ and were re-restorable (67%), but generated more shrinkage-induced cracks (47% of the specimen vs. 7% for inlays).
Significance
CAD/CAM MZ100 inlays increased the accelerated fatigue resistance and decreased the crack propensity of large MOD restorations when compared to direct restorations. While both restorative techniques yielded excellent fatigue results at physiological masticatory loads, CAD/CAM inlays seem more indicated for high-load patients.
1
Introduction
The longevity of dental restorations is influenced by multiple parameters such as material properties, patient’s conduct and dentist’s skills . Polymerization shrinkage stress of composite resin restorations is one of the major problems related to direct techniques, especially in large and high C-factor defects . Contraction stress challenges the dentin–resin hybrid layer and may result in gap formation and/or decreased dentin bond strength . On the other hand, when using strong adhesives and achieving total bonding, the composite material will shrink and cause cuspal movements, deformation and cracking of the surrounding tooth structure .
Composite resin restorative materials are increasingly popular. Advances in filler technology and in formulation of the resin matrix have resulted in significantly improved mechanical properties and reduced polymerization shrinkage . However, to optimally prevent the side-effects of polymerization shrinkage, it is recommended to use stabilized and post-polymerized luted restorations . Composite resin inlays/onlays can be fabricated using laboratory indirect techniques. A more recent trend is the use of chairside semi-direct techniques including direct and extraoral inlays and computer-aided design/computer-assisted manufactured restorations (CAD/CAM). Wassel et al. did not find clinical advantage of semi-direct post-polymerized inlay technique over direct incremental placement using the same material, after 5 years, in matched pairs of restorations. An extend review about clinical evaluations of restorative techniques demonstrated that indirect composite resin restorations had a higher mean annual failure rate (2.9%) compared with direct composite restorations (2.2%). Opdam et al. , in a 12-year survival retrospective study, found high survival rates (85%) for large three-, four- and five-surface direct composite resin restorations. In spite of the above-mentioned, indirect restorations are considered the gold standard to restore large defects , especially in consideration of shrinkage-induced crack in enamel and dentin. Stress resulting from polymerization shrinkage induces cuspal flexure but is less of a concern in luted restorations because it is restricted to a thin cement layer, accounting for a superior marginal quality . Significant additional advantages of inlays are the facilitated anatomic form, marginal adaptation, and appropriate proximal contact, contour and occlusion, especially in case of large class II preparations since the restoration is fabricated in a removable die . CAD/CAM restorations are predictable alternatives with high success rate, color stability, excellent marginal adaptation and a clinically acceptable wear . In this particularly innovative approach, the dentist is able to deliver chair-side luted porcelain restorations from an optical impression of the tooth preparation in a single appointment, avoiding the costs of dental technicians and impression materials . Lately, composite resins blocks also became available for CAD/CAM restorations, opening the range of material options . Industrial manufacturing allows the use of postcuring methods, which can improve the mechanical properties of the direct composite resin versions . Furthermore the composite resin CAD/CAM blocks present acceptable wear properties and because it is a less brittle material than porcelain, it can be used in thinner layer, allowing more conservative preparation designs and more resistant restorations . The combination of CAD/CAM technique, Paradigm MZ100 resin blocks for Cerec and immediate dentin sealing (IDS) has proven to be a conservative and biomimetic solution under fatigue loading .
This research assessed the accelerated fatigue strength and shrinkage-induced enamel crack propensity of large Class II mesial-occlusal-distal (MOD) CAD/CAM composite inlays compared to direct composite restoration in same cavity size and shape. The null hypotheses were that (1) no significant difference would be found in accelerated fatigue resistance and mode of failure among the restorative techniques used, and (2) there would be no difference in enamel crack propensity (induced by shrinkage stress) between two groups.
2
Materials and methods
Upon approval by Ethics Committee of the Federal University of Santa Catarina, Brazil and Institutional Review Board of University of Southern California, 32 extracted sound human third maxillary molars with similar size and shape were carefully selected from a large collection of teeth, scaled, pumiced and stored in 0.1% thymol solution. Each tooth was mounted in a special positioning device using acrylic resin (Palapress, Heraeus Kulzer GmbH, Dormagen, Germany), embedding the root up to 3 mm below the cementoenamel junction (CEJ). For the purpose of “enamel crack tracking” during the experiment, each surface of each tooth was photographed at baseline under standardized conditions at ×1.5 magnification (Nikon D50 and Sigma 105 mm macro lens) using a macro ring-flash (Sigma EM-140 DG). A second set of images was generated using transillumination (Microlux, Addent, Danbury, CT, USA) in order to detect existing cracks and for detection of new cracks following the subsequent procedures.
2.1
Specimen preparation
Standard preparations simulated a large MOD defect ( Fig. 1 ) using tapered diamond burs (313.029 and 314.021, Brasseler, Savannah, GA) and a high-speed electric handpiece under continuous water cooling, followed by photographic enamel crack tracking. Teeth were then randomly distributed in two groups: MZ100 ( n = 17) – Indirect restorations (CEREC inlay with Paradigm MZ100, 3M-ESPE, St. Paul, MN, USA), and M2 ( n = 15) – Direct microhybrid composite resin restoration (Miris 2, Coltène-Whaledent, Altstätten, Switzerland).
For M2 restorations only, a 0.5–1 mm 45° bevel at the cervical and proximal angles was created with a flame shape fine diamond bur (274, 011904U0, Brassler). For MZ100 Group, immediate dentin sealing (IDS) was applied to the freshly cut dentin with a three-step etch-and-rinse dentin bonding agent (Optibond FL, Kerr, Orange, CA, USA) following a previously published protocol and according to manufacturer’s recommendations. The adhesive was light polymerized for 20 s at 1000 mW/cm 2 (Valo, Ultradent, South Jordan, UT, USA) followed by an additional 10 s light polymerization under an air-blocking barrier (K-Y Jelly, Johnson & Johnson, Montreal, Canada).
2.2
Restorative procedures
Inlays were generated with the Cerec 3 CAD/CAM System (v. 3.03, Sirona Dental Systems, GmbH, Bensheim, Germany) with an average thickness of 3.5 mm at the central groove. To standardize form and anatomy, the original design of the restoration was not edited, only the position tools were used to ensure correct thickness. Restorations were milled using Paradigm MZ100 blocks (3M-ESPE, size 14) and mechanically polished using a commercial polishing kit (Kit 4477 Q-Polishing System, Komet, Lemgo, Germany). Internal surface conditioning of milled restorations included airborne-particle abrasion (RONDOflex plus 360, KaVo Dental, Charlotte, NC, USA) with 27 μm aluminum oxide at 0.2 MPa, followed by cleaning using 35% phosphoric acid (Ultradent, South Jordan, UT, USA) with a gentle brushing motion for 1 min. After rinsing for 30 s, the inlays were immersed in distilled water in an ultrasonic bath for 2.5 min, air-dried and silanated (Silane, Ultradent) and dried at 100 °C for 1 min (DI500 oven; Coltène Whaledent AG, Alstätten, Switzerland). Tooth preparations were treated by airborne-particle abrasion with 27-μm aluminum oxide at 0.2 MPa, etching for 30 s with 35% phosphoric acid and abundant rinsing and drying. Adhesive resin (Optibond FL, bottle 2; Kerr) was applied to both fitting surfaces (tooth and inlay) and left unpolymerized until the luting material (Filtek Z100, 3M-ESPE), preheated for 5 min at 68 °C in Calset (Addent, Danbury, CT, USA), was inserted into the preparation and followed by the complete seating of the inlay. After careful elimination of composite resin excesses, each surface was light polymerized for a total of 60 s (20 s per surface, repeated 3 times) and another 10 s under an air-blocking barrier. The margins were finished and polished mechanically using tungsten carbide burs and composite resin polishers with diamond grit (kit 4477, Q-Polishing System Komet, Lemgo, Germany). Two teeth of this group (one right and one left third maxillary molar), were not used for testing but served as a guide to copy the cusp inclination and occlusal anatomy while layering restorations in M2 Group.
For direct composite restorations in M2 Group, the same previous three-step total-etch bonding agent was used (OptiBond, FL, USA). The natural layering technique (enamel and dentin shades) in seven increments was applied. First, proximal walls were raised with a 2-mm thick dentin shade (Miris S2) increment and followed by a 2-mm thick enamel shade (Miris NR) increment for the marginal ridge. The remaining class I defect was filled with two 1.5-mm horizontal increments of the same dentin shade and one increment of the same enamel. Special attention was used to strictly emulate the cuspal inclination and occlusal anatomy of the reference CAD/CAM inlays. Each increment was polymerized for 20 s at 1000 mW/cm 2 and final light polymerization was performed under an air-blocking barrier (KY Jelly, Johnson & Johnson) for 10 s. Finishing procedures were the same as for the MZ100 Group.
2.3
Fatigue testing
Restored specimens were kept in distilled water at ambient temperature for 1 week following adhesive procedures. Each tooth surface was then subjected again to enamel crack tracking (transillumination and photography). An artificial mouth using closed-loop servohydraulics (Mini Bionix II; MTS Systems, Eden Prairie, MN, USA) was used to simulate the masticatory forces with an antagonist 7 mm-diameter composite resin sphere (Filtek Z100, 3M-ESPE) post-polymerized at 100 °C for 5 min . These composite resin spheres contacted simultaneously and equally the mesiobucal, distobucal and lingual cusps (tripod contact) with isometric chewing under a 5 Hz of frequency. The load chamber was filled with distilled water until complete immersion of specimens and, the first 5000 cycles was a warm-up load of 200 N, followed by stages of 400, 600, 800, 1000, 1200 and 1400 N at a maximum of 30,000 cycles each ( Fig. 2 ). Specimens were loaded until fracture or to a maximum of 185,000 cycles and the number of endured cycles was registered. Under optical microscope and with a two-examiner agreement, the distinction between restorable or non-restorable fractures was made ( Fig. 3 ). A restorable fracture is usually above the cementum–enamel junction, meaning that even in case of major coronal substance loss, the tooth can be re-restored. A non-restorable fracture involves a large portion of the tooth and extends below the cementoenamel junction.
2.4
Enamel crack detection and tracking
Specimens were evaluated multiple times during the experiment in order to detect new enamel cracks at ×1.5 magnification in standardized conditions and with transillumination (Nikon D50 and Sigma 105 mm macro lens using a macro ring-flash Sigma EM-140 DG or Microlux, Addent) before and after tooth preparation, 1 week after restoration, and at the end of the fatigue test. In case of doubt, the sample was evaluated in a two-examiner agreement and analyzed under optical microscope at 10:1 magnification (Leica MZ 125, Leica Microsystems, Wetzlar, Germany). Special attention was taken to differ between pre-existed cracks from those created by polymerization shrinkage. Since many different sizes of cracks were observed, a classification with three categories was created: (a) no cracks visible, (b) visible cracks smaller than 3 mm, and (c) visible cracks larger than 3 mm ( Fig. 4 ).
2.5
Statistical analysis
The fatigue resistance of the two groups was compared using the life table survival analysis. At each time interval (defined by each load step), the number of specimens beginning the interval intact and the number of fractured specimens during the interval were counted, providing the survival probability (%) at each load step. The influence of the restorative technique and material on the fatigue resistance was observed comparing the survival curves using the log rank test at a significance level of .05.
2
Materials and methods
Upon approval by Ethics Committee of the Federal University of Santa Catarina, Brazil and Institutional Review Board of University of Southern California, 32 extracted sound human third maxillary molars with similar size and shape were carefully selected from a large collection of teeth, scaled, pumiced and stored in 0.1% thymol solution. Each tooth was mounted in a special positioning device using acrylic resin (Palapress, Heraeus Kulzer GmbH, Dormagen, Germany), embedding the root up to 3 mm below the cementoenamel junction (CEJ). For the purpose of “enamel crack tracking” during the experiment, each surface of each tooth was photographed at baseline under standardized conditions at ×1.5 magnification (Nikon D50 and Sigma 105 mm macro lens) using a macro ring-flash (Sigma EM-140 DG). A second set of images was generated using transillumination (Microlux, Addent, Danbury, CT, USA) in order to detect existing cracks and for detection of new cracks following the subsequent procedures.
2.1
Specimen preparation
Standard preparations simulated a large MOD defect ( Fig. 1 ) using tapered diamond burs (313.029 and 314.021, Brasseler, Savannah, GA) and a high-speed electric handpiece under continuous water cooling, followed by photographic enamel crack tracking. Teeth were then randomly distributed in two groups: MZ100 ( n = 17) – Indirect restorations (CEREC inlay with Paradigm MZ100, 3M-ESPE, St. Paul, MN, USA), and M2 ( n = 15) – Direct microhybrid composite resin restoration (Miris 2, Coltène-Whaledent, Altstätten, Switzerland).
For M2 restorations only, a 0.5–1 mm 45° bevel at the cervical and proximal angles was created with a flame shape fine diamond bur (274, 011904U0, Brassler). For MZ100 Group, immediate dentin sealing (IDS) was applied to the freshly cut dentin with a three-step etch-and-rinse dentin bonding agent (Optibond FL, Kerr, Orange, CA, USA) following a previously published protocol and according to manufacturer’s recommendations. The adhesive was light polymerized for 20 s at 1000 mW/cm 2 (Valo, Ultradent, South Jordan, UT, USA) followed by an additional 10 s light polymerization under an air-blocking barrier (K-Y Jelly, Johnson & Johnson, Montreal, Canada).
2.2
Restorative procedures
Inlays were generated with the Cerec 3 CAD/CAM System (v. 3.03, Sirona Dental Systems, GmbH, Bensheim, Germany) with an average thickness of 3.5 mm at the central groove. To standardize form and anatomy, the original design of the restoration was not edited, only the position tools were used to ensure correct thickness. Restorations were milled using Paradigm MZ100 blocks (3M-ESPE, size 14) and mechanically polished using a commercial polishing kit (Kit 4477 Q-Polishing System, Komet, Lemgo, Germany). Internal surface conditioning of milled restorations included airborne-particle abrasion (RONDOflex plus 360, KaVo Dental, Charlotte, NC, USA) with 27 μm aluminum oxide at 0.2 MPa, followed by cleaning using 35% phosphoric acid (Ultradent, South Jordan, UT, USA) with a gentle brushing motion for 1 min. After rinsing for 30 s, the inlays were immersed in distilled water in an ultrasonic bath for 2.5 min, air-dried and silanated (Silane, Ultradent) and dried at 100 °C for 1 min (DI500 oven; Coltène Whaledent AG, Alstätten, Switzerland). Tooth preparations were treated by airborne-particle abrasion with 27-μm aluminum oxide at 0.2 MPa, etching for 30 s with 35% phosphoric acid and abundant rinsing and drying. Adhesive resin (Optibond FL, bottle 2; Kerr) was applied to both fitting surfaces (tooth and inlay) and left unpolymerized until the luting material (Filtek Z100, 3M-ESPE), preheated for 5 min at 68 °C in Calset (Addent, Danbury, CT, USA), was inserted into the preparation and followed by the complete seating of the inlay. After careful elimination of composite resin excesses, each surface was light polymerized for a total of 60 s (20 s per surface, repeated 3 times) and another 10 s under an air-blocking barrier. The margins were finished and polished mechanically using tungsten carbide burs and composite resin polishers with diamond grit (kit 4477, Q-Polishing System Komet, Lemgo, Germany). Two teeth of this group (one right and one left third maxillary molar), were not used for testing but served as a guide to copy the cusp inclination and occlusal anatomy while layering restorations in M2 Group.
For direct composite restorations in M2 Group, the same previous three-step total-etch bonding agent was used (OptiBond, FL, USA). The natural layering technique (enamel and dentin shades) in seven increments was applied. First, proximal walls were raised with a 2-mm thick dentin shade (Miris S2) increment and followed by a 2-mm thick enamel shade (Miris NR) increment for the marginal ridge. The remaining class I defect was filled with two 1.5-mm horizontal increments of the same dentin shade and one increment of the same enamel. Special attention was used to strictly emulate the cuspal inclination and occlusal anatomy of the reference CAD/CAM inlays. Each increment was polymerized for 20 s at 1000 mW/cm 2 and final light polymerization was performed under an air-blocking barrier (KY Jelly, Johnson & Johnson) for 10 s. Finishing procedures were the same as for the MZ100 Group.
2.3
Fatigue testing
Restored specimens were kept in distilled water at ambient temperature for 1 week following adhesive procedures. Each tooth surface was then subjected again to enamel crack tracking (transillumination and photography). An artificial mouth using closed-loop servohydraulics (Mini Bionix II; MTS Systems, Eden Prairie, MN, USA) was used to simulate the masticatory forces with an antagonist 7 mm-diameter composite resin sphere (Filtek Z100, 3M-ESPE) post-polymerized at 100 °C for 5 min . These composite resin spheres contacted simultaneously and equally the mesiobucal, distobucal and lingual cusps (tripod contact) with isometric chewing under a 5 Hz of frequency. The load chamber was filled with distilled water until complete immersion of specimens and, the first 5000 cycles was a warm-up load of 200 N, followed by stages of 400, 600, 800, 1000, 1200 and 1400 N at a maximum of 30,000 cycles each ( Fig. 2 ). Specimens were loaded until fracture or to a maximum of 185,000 cycles and the number of endured cycles was registered. Under optical microscope and with a two-examiner agreement, the distinction between restorable or non-restorable fractures was made ( Fig. 3 ). A restorable fracture is usually above the cementum–enamel junction, meaning that even in case of major coronal substance loss, the tooth can be re-restored. A non-restorable fracture involves a large portion of the tooth and extends below the cementoenamel junction.
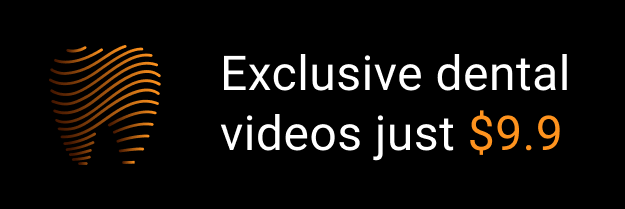