Highlights
- •
No significant differences were found in terms of fatigue resistance between crowns and endocrowns.
- •
Monolithic crowns performed better than the buccal-occlusal veneered counterparts.
- •
Endocrowns fractured predominantly with a mesio-distal wedge-opening fracture (82%).
- •
Partial cusp fractures were observed above all in crowns (70%).
Abstract
Objective
To evaluate the influence of different types of modifications with resin on fatigue resistance and failure behavior of CAD–CAM resin nano ceramic (RNC) restorations for maxillary first premolars.
Methods
Sixty standardized resin composite root dies received CAD–CAM RNC endocrowns (n = 30) and crowns (n = 30) (Lava Ultimate, 3M Espe). Restorations were divided into six groups: full anatomic endocrowns (group A) and crowns (group D), buccal resin veneered endocrowns (group B) and crowns (group E) and buccal resin veneered endocrowns (group C) and crowns (group F) with a central groove resin filling. A nano-hybrid resin composite was used to veneer the restorations (Filtek Supreme, 3M Espe). All specimens were first submitted to thermo-mechanical cyclic loading (1.7 Hz, 49 N, 600 000 cycles, 1500 thermo-cycles) and then submitted to cyclic isometric stepwise loading (5 Hz) until completion of 105 000 cycles or failure after 5000 cycles at 200 N, followed by 20 000 cycles at 400 N, 600 N, 800 N, 1000 N and 1200 N. In case of fracture, fragments were analyzed using SEM and modes of failure were determined. Results were statistically analyzed by Kaplan–Meier life survival analysis and log rank test (p = 0.05).
Results
The differences in survival between groups were not statistically significant, except between groups D and F (p = 0.039). Endocrowns fractured predominantly with a mesio-distal wedge-opening fracture (82%). Partial cusp fractures were observed above all in crowns (70%). Analysis of the fractured specimens revealed that the origin of the fracture was mainly at the occlusal contact points of the stepwise loading.
Significance
Veneering of CAD–CAM RNC restorations has no influence on their fatigue resistance except when monolithic crowns are modified on their occlusal central groove.
1
Introduction
CAD–CAM (computer-aided design/computer-aided machining) technology for dental purposes has highly evolved in the last few years. Hardware has become cheaper, software has become user friendly, fabrication is now faster and the milled workpieces are more accurate in respect of the anatomic form and dimensions as well as of the fit of the margins . Early in-vivo performances of CAD–CAM restorations are also encouraging . As a consequence, a wide range of new tooth-colored ceramic and resin based blocks are now available on the market. Ceramic materials, especially lithium-disilicate reinforced ones, display better mechanical (flexural strength, hardness, thoughness, wear resistance) and optical (translucency, opalescence, gloss) properties than the particulate filled resin materials. Polymer based materials are more interesting with regard to the ease of fabrication and practical clinical features such as the possibility of surface modification for functional or esthetic reasons by safe intra-oral repair, the latter accomplished by surface sandblasting, while toxic hydrofluoric acid is normally needed for ceramic repair . Some authors have also reported an optimal fatigue resistance of restorations made out of resin composite blocks, claiming their stress adsorbing properties . Recently, resin materials with high ceramic fillers content have been introduced in the attempt to obtain intermediate properties between classical particulate-filled resins and ceramics. Lava Ultimate (3M Espe, St. Paul, MN, USA) is one of the firstborns of these “hybrid” materials. It has been introduced by the manufacturer as a resin nano-ceramic (RNC) material as the dimethacrylate resin matrix is charged with silica and zirconia nano-fillers to an extent of approximately 80% by weight. Improved flexural strength and toughness compared to previous resin composite blocks (Paradigm MZ100, 3M Espe) and similar fatigue resistance as well as fracture strength to glass-ceramics have been reported for Lava Ultimate restorations. Some concerns arise about the esthetics of this material, as blocks are monochromatic and an esthetic modification of the raw workpiece after milling may be necessary depending on esthetic needs of the patient. This modification may be accomplished by replacing 1–2 mm of the buccal and/or occlusal part of the CAD–CAM restoration with an esthetic resin composite layer . Restorative hybrid resins are often used for buccal veenering while stained resins with flowable consistency are used to mimic the occlusal fissure shade and anatomy. The adhesive link between the CAD–CAM core and the resin composite which is stratified on it has recently been argued. Lava resin blocks are industrially polymerized and display a high degree of monomer conversion resulting in a low amount of free radicals . A mechanical pre-conditioning of the surface by high-pressure air abrasion or silica coating has proved to have a positive impact on micromechanical retention and adhesive strength . Likewise, the use of a silane and a bonding resin as chemical intermediate agents over the conditioned CAD–CAM restoration surface seems today mandatory to reach an adequate bond strength with the veneering resin composite . The objective of the modification is to improve the esthetic aspect of the CAD–CAM restoration without compromising its mechanical properties. So far, the effect of such a structural modification on mechanical performances of CAD–CAM resin restorations has not yet been investigated. In fact, one of the major advantages of CAD–CAM workpieces in comparison to the classical lab-made ones is that they are milled from resin blocks which are fabricated under standardized and controlled high-pressure/high-temperature polymerization conditions. The resin composite produced is highly homogeneous and its mechanical properties are superior to the chair-side photopolymerized resin counterparts . Replacing a part of the CAD–CAM resin restoration with a chair-side restorative resin composite could jeopardize the mechanical integrity of the milled restoration. Moreover, it is reasonable to suppose that this procedure could have a potential higher negative impact on crown restorations than for endocrowns as their thickness is limited by the presence of a core inside. The aim of this paper was to test in-vitro the fatigue behavior and the fracture mode of veneered CAD–CAM RNC crowns and endocrowns for upper premolars. Buccal and occlusal modifications of the restorations after cutting-back were tested. It was hypothesized that (a) buccal resin veneering of CAD–CAM RNC monolithic restorations has a negative impact on their mechanical performances and (b) this impact is higher if an occlusal composite resin filling of the central groove is introduced.
2
Materials and methods
2.1
Endocrowns/crowns fabrication
An extracted maxillary first premolar tooth was chosen as a master model. An optical impression of the crown (Bluecam, Cerec) was used to fabricate the crown anatomy of the CAD/CAM restorations using the software Cerec 4.0 in Biogeneric Copy Design mode. Then the premolar tooth model was cut at the CEJ and prepared for an endocrown restoration. An optical impression of the cavity was taken and the full anatomic CAD/CAM endocrown restorations were milled (n = 10, full anatomic endocrowns, control group A) ( Fig. 1 a). Endocrowns were 7.4-mm thick at the mesio-distal sulcus, including the 2.8 mm of the endo-core. Then, new endocrown restorations were fabricated with the same procedure but digitally cut-back with the Cerec software (6 × 5 mm, 1-mm deep) on the buccal side (n = 10, buccal veneered endocrowns, group B) and equally reduced on the buccal side but with a 1 × 3 mm, 1-mm deep occlusal groove (n = 10, buccal veneered endocrowns with an occlusal central groove, group C). Thereafter, a 2.8-mm high resin composite core was built up on the premolar root model and prepared for a crown restoration, without touching the outer limits (chamfer) of the former preparation for the endocrown. An optical impression of this core was taken ( Fig. 1 b) and the full anatomic CAD/CAM crown restorations were milled with the Cerec machine basing on the same Biogeneric Copy of group A (n = 10, full anatomic crowns, control group D). Crowns had standard dimensions and a thickness of 1.8 mm at the mesio-distal sulcus. New CAD/CAM crown restorations were then fabricated with the same procedure but with 6 × 5 mm, 1-mm deep buccal reduction (n = 10, buccal veneered crowns, group E) and with equal buccal reduction and above an occlusal 1 × 3 mm, 1-mm deep groove (n = 10, buccal veneered crowns with an occlusal central groove, group F) ( Fig. 1 c).
2.2
Root dies fabrication
At the beginning, a trasparent silicon mold (Memosil 2, Heraeus Kulzer, Hanau, Germany) was created from the premolar tooth model and cut horizontally 1 mm over the CEJ. For each specimen, a nano-hybrid resin composite (Clearfil Majesty Posterior, Kuraray, Okayama, Japan) with an elastic modulus similar to that of human dentin (E-modulus 22 GPa) was inserted in the silicon mold to fabricate the root dies. Then, each restoration was isolated with glycerine, seated over the resin inside the silicone mold in order to stamp on this unpolymerized resin the shape of its inner-marginal area. The resin die simulating the root was then polymerized by using a LED lamp (Bluephase, Ivoclar-Vivadent, Schaan, Liechtenstein) through the transparent silicon mold. Root dies for endocrowns had 2.8-mm deep central pit corresponding to the endo-core of the restoration, surrounded by a chamfer preparation of around 1,2 mm. The core of the root dies for crown preparation was 2.8-mm high with the same chamfer preparation. All root dies were vertically fixed on a metallic holder. The base was embedded with self curing PMMA resin (Technovit 4071, Heraeus Kulzer, Hanau, Germany) till 1 mm under the artificial CEJ to complete the root stabilization.
2.3
Luting of the endocrowns/crowns
The intaglio surface of the CAD/CAM restorations as well as the root dies cavities were sandblasted with 27 μm aluminum-oxide powder at about 0.2 MPa pressure for 5 s (MicroEtcher CD-Intraoral Sandblaster, Danville Materials, San Ramon, CA 94583, USA), rinsed and dried. The conditioned surfaces were then treated with an adhesive system (Scotchbond Universal Adhesive, 3M ESPE, Seefeld, Germany) without curing. A dual-curing resin composite (RelyX Ultimate, A2 shade, 3M ESPE, Seefeld, Germany) was used as luting cement. The restoration was seated in place first with manual pressure and then with the assistance of a specific ultra-sonic device (Cementation tip, EMS, Nyon, Switzerland). After removal of the excess cement, each restoration surface was light-cured for 60 s (buccal, occlusal and palatal) and margins were polished with discs of decreasing grain size, from coarse (80 μm) to superfine (20 μm) (SoFlex, 3M ESPE, Seefeld, Germany).
2.4
Veneering of the endocrowns/crowns
The grinded buccal and occlusal surfaces of specimens of groups B, C, E and F were submitted to airborne-particle abrasion with 27 μm aluminum oxide particles at about 0.2 MPa pressure for 5 s, rinsed and dried. The adhesive system (Scotchbond Universal Adhesive) was applied on all sandblasted surfaces and light-cured. A nanofilled resin composite (Filtek Supreme XTE Universal Restorative, A3B, 3M ESPE, Seefeld, Germany) was veneered on restorations’ buccal cavity in one increment and photo-polymerized for 30 s. It was then polished with discs of decreasing grain size. The occlusal grooves of groups C and F were filled with a flowable resin composite (Filtek Supreme XTE Flowable A3, 3M ESPE, Seefeld, Germany) and photo-polymerized for 30 s ( Fig. 2 and Table 1 ).
Brand name (manufacturer) | Chemical composition a | E-modulus (GPa) | Fracture toughness K IC (MPa√m) |
---|---|---|---|
Lava Ultimate (3M ESPE) | Highly cross-linked resin matrix reinforced by 80 wt% of silane treated nano zirconia–silica particles agglomerated to clusters (0,6–10 μm) and individual silane bonded nano silica or zirconia particles (<20 nm) | 12,77 a | 2,02 a |
RelyX Ultimate (3M ESPE) | Adhesive resin cement consisting of methacrylate monomers, radiopaque, silanated fillers, initiator components, stabilizers, rheological additives, fluorescence dye dark cure activator for Scotchbond Universal adhesive | 7,7 a | 1,95 b |
Scotchbond Universal Adhesive (3M ESPE) | MDP, HEMA | Not furnished | Not furnished |
Vitrebond™ Copolymer | |||
Filler, ethanol, water, initiators, silane | |||
Filtek Supreme XTE Universal (3M ESPE) | Bis-GMA, UDMA, TEGDMA, and bis-EMA resins, non-aggregated 20 nm silica filler, non-aggregated 4–11 nm zirconia filler, and aggregated zirconia/silica cluster filler (comprised of 20 nm silica and 4–11 nm zirconia particles) | 11,3 a | 1,84 a |
Filtek Supreme XTE Flowable (3M ESPE) | Bis-GMA, TEGDMA and Procrylat resins. Fillers of ytterbium trifluoride (particles 0.1–5.0 μm), a non-aggregated surface-modified 20 nm and 75 nm silica filler, a surface-modified aggregated zirconia/silica cluster filler (comprised of 20 nm silica and 4–11 nm zirconia particles) clusters (0.6–10 μm) | 6,8 a | 1,67 a |
Clearfil Majesty Posterior (Kuraray) | Light-cure, nano-superfilled, radiopaque restorative posterior composite resin composed of nano and micro inorganic filler, silanated glass ceramic filler (average: 1.5 μm), surface treated alumina micro filler (average: 20 nm) | 22 a | 1,06 c |
Bis-GMA, TEGDMA, dl -camphorquinone, accelerators, pigments |
2.5
Thermo-mechanical fatigue loading
After 24 h the stress test was carried out with an established thermo-mechanical fatigue method, in a chewing simulator for Thermal Cycling and Mechanical Loading (TCML). All specimens were subjected to 600 000 cycles with 49 N axial occusal loading force applied with a ball of 3-mm diameter on the buccal cusp at a 1.7 Hz frequency following a one-half sine wave curve. By having the specimen holder mounted on a hard rubber disc, a sliding movement of the tooth is produced between the first contact on an inclined plane of the buccal cusp (mesial or distal) and the central fossa ( Fig. 3 a). A total of 1500 thermo-cycles (5 °C to 50 °C to 5 °C) were performed simultaneously.
2.6
Stepwise fatigue loading
After the TCML test all specimens were subjected to a cyclic loading test with a MTS Mini Bionix 858.02 servohydraulic testing system (Mini Bionix II, MTS, Eden Prairie, MN, USA) according to a stepwise loading method. The system was equipped with a load cell with a range of 0–2500 N. The chewing cycle was simulated by an isometric contraction. The loading member was a stainless steel ball of 3-mm diameter. Fatigue testing was carried out with unidirectional axial force. Because of the standardized anatomy, all restorations were adjusted in the same position with the loading sphere contacting both buccal and palatal cusps, halfway of the slope ( Fig. 3 b). The load varied sinusoidally between a nominal peak value F and 10% of this value (R = 0.1). The loading frequency was 5 Hz. The first 5000 cycles was a warm-upload at 200 N, followed by stages at 400, 600, 800, 1000 and 1200 N of a maximum of 20 000 cycles each. Specimens were loaded until fracture or to a maximum of 105 000 cycles and the number of endured cycles was registered. The integrity of the specimens was monitored throughout the test with a peak detector (Peak/Valley detector, MTS) which recognizes the difference between current loading and prescribed loading curve. The deviation was usually connected with excessive wear, accidental movements and first micro fractures inside the restoration.
2.7
Fractography
After fracture all the specimens were visually examined in order to establish which fragments were suitable for fractographic analysis. The first examination of the broken specimens was performed using a stereomicroscope (SZX9, Olympus optical Co., Ltd., Tokyo, Japan). Characteristic features like compression curl, hackle and arrest lines were identified. Different magnifications (ranging from 6.3× to 50×) were used depending on the size of the characteristic marks detected. Angled illumination was used to better view the fracture surface. All recognizable features were photographed and documented. Scanning Electron Microscopy (SEM) (Digital SEM XL20, Philips, Amsterdam, Netherlands) was then used for a more detailed analysis of the fractured surfaces. In order to remove all of the impurities, all fragments were cleaned in an ultrasonic 10% sodium hypochlorite bath for 3 min, rinsed with water, dried and then fixed on the support for the microscope. The specimens were gold coated prior to the analysis with the SEM. Magnifications up to 2000× were used to obtain higher definition of identified crack features in selected areas of interest. The overall direction of crack propagation and failure origin(s) were systematically mapped for all specimens.
The modes of fracture were analyzed by optical stereo microscopy and classified as (1) cuspal fracture or (2) split vertical fracture. Classification was based on an agreement between three examiners.
2
Materials and methods
2.1
Endocrowns/crowns fabrication
An extracted maxillary first premolar tooth was chosen as a master model. An optical impression of the crown (Bluecam, Cerec) was used to fabricate the crown anatomy of the CAD/CAM restorations using the software Cerec 4.0 in Biogeneric Copy Design mode. Then the premolar tooth model was cut at the CEJ and prepared for an endocrown restoration. An optical impression of the cavity was taken and the full anatomic CAD/CAM endocrown restorations were milled (n = 10, full anatomic endocrowns, control group A) ( Fig. 1 a). Endocrowns were 7.4-mm thick at the mesio-distal sulcus, including the 2.8 mm of the endo-core. Then, new endocrown restorations were fabricated with the same procedure but digitally cut-back with the Cerec software (6 × 5 mm, 1-mm deep) on the buccal side (n = 10, buccal veneered endocrowns, group B) and equally reduced on the buccal side but with a 1 × 3 mm, 1-mm deep occlusal groove (n = 10, buccal veneered endocrowns with an occlusal central groove, group C). Thereafter, a 2.8-mm high resin composite core was built up on the premolar root model and prepared for a crown restoration, without touching the outer limits (chamfer) of the former preparation for the endocrown. An optical impression of this core was taken ( Fig. 1 b) and the full anatomic CAD/CAM crown restorations were milled with the Cerec machine basing on the same Biogeneric Copy of group A (n = 10, full anatomic crowns, control group D). Crowns had standard dimensions and a thickness of 1.8 mm at the mesio-distal sulcus. New CAD/CAM crown restorations were then fabricated with the same procedure but with 6 × 5 mm, 1-mm deep buccal reduction (n = 10, buccal veneered crowns, group E) and with equal buccal reduction and above an occlusal 1 × 3 mm, 1-mm deep groove (n = 10, buccal veneered crowns with an occlusal central groove, group F) ( Fig. 1 c).
2.2
Root dies fabrication
At the beginning, a trasparent silicon mold (Memosil 2, Heraeus Kulzer, Hanau, Germany) was created from the premolar tooth model and cut horizontally 1 mm over the CEJ. For each specimen, a nano-hybrid resin composite (Clearfil Majesty Posterior, Kuraray, Okayama, Japan) with an elastic modulus similar to that of human dentin (E-modulus 22 GPa) was inserted in the silicon mold to fabricate the root dies. Then, each restoration was isolated with glycerine, seated over the resin inside the silicone mold in order to stamp on this unpolymerized resin the shape of its inner-marginal area. The resin die simulating the root was then polymerized by using a LED lamp (Bluephase, Ivoclar-Vivadent, Schaan, Liechtenstein) through the transparent silicon mold. Root dies for endocrowns had 2.8-mm deep central pit corresponding to the endo-core of the restoration, surrounded by a chamfer preparation of around 1,2 mm. The core of the root dies for crown preparation was 2.8-mm high with the same chamfer preparation. All root dies were vertically fixed on a metallic holder. The base was embedded with self curing PMMA resin (Technovit 4071, Heraeus Kulzer, Hanau, Germany) till 1 mm under the artificial CEJ to complete the root stabilization.
2.3
Luting of the endocrowns/crowns
The intaglio surface of the CAD/CAM restorations as well as the root dies cavities were sandblasted with 27 μm aluminum-oxide powder at about 0.2 MPa pressure for 5 s (MicroEtcher CD-Intraoral Sandblaster, Danville Materials, San Ramon, CA 94583, USA), rinsed and dried. The conditioned surfaces were then treated with an adhesive system (Scotchbond Universal Adhesive, 3M ESPE, Seefeld, Germany) without curing. A dual-curing resin composite (RelyX Ultimate, A2 shade, 3M ESPE, Seefeld, Germany) was used as luting cement. The restoration was seated in place first with manual pressure and then with the assistance of a specific ultra-sonic device (Cementation tip, EMS, Nyon, Switzerland). After removal of the excess cement, each restoration surface was light-cured for 60 s (buccal, occlusal and palatal) and margins were polished with discs of decreasing grain size, from coarse (80 μm) to superfine (20 μm) (SoFlex, 3M ESPE, Seefeld, Germany).
2.4
Veneering of the endocrowns/crowns
The grinded buccal and occlusal surfaces of specimens of groups B, C, E and F were submitted to airborne-particle abrasion with 27 μm aluminum oxide particles at about 0.2 MPa pressure for 5 s, rinsed and dried. The adhesive system (Scotchbond Universal Adhesive) was applied on all sandblasted surfaces and light-cured. A nanofilled resin composite (Filtek Supreme XTE Universal Restorative, A3B, 3M ESPE, Seefeld, Germany) was veneered on restorations’ buccal cavity in one increment and photo-polymerized for 30 s. It was then polished with discs of decreasing grain size. The occlusal grooves of groups C and F were filled with a flowable resin composite (Filtek Supreme XTE Flowable A3, 3M ESPE, Seefeld, Germany) and photo-polymerized for 30 s ( Fig. 2 and Table 1 ).
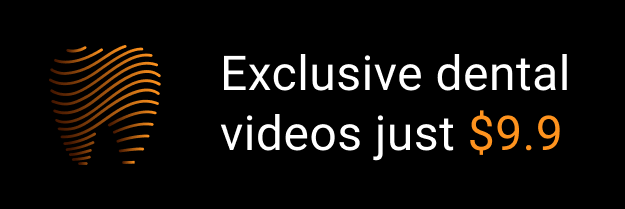