Abstract
Objectives
This study aimed to evaluate the dentin wall carious lesion development of different composite-dentin interfaces in the presence of two adhesive bonding materials in the gaps, using a microcosm biofilm model.
Methods
Dentin samples were prepared (10.4 mm 2 ) and restored with a composite resin using two adhesive systems (etch-and-rinse and self-etch techniques). Different conditions with respect to composite-dentin interfaces were produced with a 200 μm gap: failed bonded without ageing or after mechanical ageing, or non-bonded with or without the presence of adhesive material on the dentin wall. For cariogenic challenge, specimens were subjected to a biofilm microcosm model for 14 days to create caries-like wall lesions. Before and after caries development, transverse wavelength-independent microradiography images were taken, and lesion depth and mineral loss were measured. Data were analysed with linear regression models ( p < 0.05).
Results
The composite-dentin interface conditions significant influenced the caries development: lesion development was reduced by the presence of the adhesive material on dentin wall, while lesion development was increased by the mechanical ageing ( p = 0.019). There was no difference between the adhesive materials ( p values > 0.05).
Conclusion
Different composite-dentin interfaces influence wall lesion development in gaps, with the interfaces submitted to ageing showing less carious protection than those interfaces with the presence of adhesive covering the dentin.
Clinical significance
The presence of adhesive bonding material in the gaps plays a role on the wall caries lesion development.
1
Introduction
The most common reasons for posterior restoration failure are caries and fracture , with secondary caries as the main cause of composite restoration failure in high caries-risk patients . Secondary caries refers to a carious lesion affecting the margins of an existing restoration , and it is described to consist of an outer and an inner/wall lesion . The outer lesion is caused by the primary caries attack on the tooth surface, while the wall lesion is the result of bacteria, fluids and acids entering the gap between restoration and cavity wall .
Considering secondary caries, wall lesions seem to be a clinical reality. It was shown that inner wall lesions of secondary caries could develop without the presence of outer lesions . Several clinical and in vitro studies have indicated that the width of marginal gaps is related to the presence of secondary caries development . However, one in situ study did not find such a relationship . It was suggested that a minimum gap size for wall lesion development is required . Interfacial gaps larger than 68 μm seem to be susceptible to demineralization, and may thus lead to caries development . Thus, marginal integrity may be an important factor in restoration survival and, therefore, indirectly impact the longevity of affected teeth and health expenditure.
Despite all available evidence on secondary caries and composite restoration, the influence of gaps has been studied with a very limited model in laboratory studies. Until now, almost all secondary caries studies with gaps have created artificial gaps without the presence of bonding in the interfacial gap. However, recently, the presence of adhesive systems in gaps between composite and dentin has been reported to play a role in caries development .
In a clinical situation, the restoration interface with a gap is unlikely to contain an adhesive simply present, on either attached to the tooth tissue or to the composite surface. It is more likely that this gap has been created through failure of a previously bonded interface, usually after some degree of degradation and fatigue by ageing, and the caries susceptibility of such an aged failed bonded interface is unknown. A previous study showed that such failed bonded interfaces present less caries development than untreated dentin . In that study, caries demineralization was modeled with a lactic acid gel. More complex caries models, such as biofilm models, should be used to study caries development in failed bonded interfaces in order to increase clinical relevance. Microcosm biofilms formed in microplates have demonstrated complex community dynamics similar to natural dental biofilm . Oral biofilms are polymicrobial complexes that under certain environmental conditions may play a critical role in the progression of dental caries, since metabolic products not only demineralize the underlying dental tissues, but also degrade the composite and adhesive of a restoration .
Therefore, the aim of this in vitro study was to evaluate the dentin carious wall lesion development in failed bonded composite-dentin interfaces, in the presence of two adhesive bonding materials in the gaps, using a microcosm biofilm model.
2
Materials and methods
2.1
Study design
This was an in vitro study evaluating different composite-dentin interface conditions (failed bond broken after no ageing or mechanical ageing, or non-bonded with or without adhesive on dentin) and adhesive materials (etch-and-rinse and self-etch techniques). A microcosm biofilm model was used to create caries-like lesions, during 14 days. Outcome variables were dentin wall lesion depth and mineral loss.
2.2
Selection and preparation of teeth
Thirty-five freshly extracted sound human molars were selected, cleaned and stored in water. Flat midcoronal dentin surfaces were exposed by enamel removal using #150 grit SiC paper (Siawat Abrasives, Frauenfeld, Switzerland), under running water. Complete removal of enamel was confirmed by stereomicroscopic examination. Subsequently, the dentin surfaces were polished using #600 grit SiC paper (Siawat Abrasives, Frauenfeld, Switzerland).
Two bonding materials, a two-step self-etch adhesive (Clearfil™ SE-Bond – CSE, Kuraray Medical Inc., Tokyo, Japan) and an universal adhesive system applied with etch-and-rinse technique (Scotchbond™ Universal – SU, 3 M ESPE, St. Paul, MN, USA), were applied on the dentin surface according to the manufacturers’ instructions. The bonding materials were light-cured for 10 s using a LED curing device, with an intensity of ≈900 mW/cm 2 (Fusion™ S7 Curing Light, DentLight Inc., Richardon, USA). The restorations were made with a composite resin (Clearfil™ AP-X, Kuraray Medical Inc., Tokyo, Japan). Each composite increment was light-cured for 20 s.
Fig. 1 illustrates schematically the steps of the study. One trained operator performed all adhesive and restorative procedures. The samples were prepared in different ways using the two bonding materials and the composite resin, in order to produce different composite-dentin interfaces. All groups were made to include an interfacial gap, resulting in the following seven experimental groups:
Failed bonded, no ageing (CSE/SU)
Failed bonded, after mechanical ageing (CSE/SU)
Non-bonded, with adhesive material on the dentin (CSE/SU)
Non-bonded, no adhesive material on the dentin.
2.2.1
Failed bonded samples
Twenty teeth were used to produce failed bonded samples ( Fig. 1 a). The prepared teeth were embedded in acrylic resin (16 mm high), leaving the prepared occlusal dentin surface free. These samples were optimally bonded with one of the two adhesive materials (n = 10 teeth), and composite resin restorations were built up to ±4 mm in height. After the restorative procedures, the samples remained stored in distilled water for 24 h, at 37 °C. Subsequently, the samples were randomly divided according to the ageing conditions (n = 5 teeth): no ageing (control – 24 h in distilled water) or mechanical loading ageing. Mechanical loading was performed using a Rub&Roll loading device (30 N of force, 0.4 Hz) for three weeks, resulting in 750.000 mechanical cycles. The Rub&Roll device used for applying mechanical loading is described in detail elsewhere . The mechanical loading was performed at room temperature in water, which was weekly changed.
The ageing was performed before sectioning on whole restored teeth. All restored teeth were sectioned into rectangular composite-dentin samples with an approximate cross-sectional adhesive area of 10.4 mm 2 (3.2 × 3.2 × 8 mm), using a low speed diamond saw under continuous water-cooling. This resulted in the generation of two composite-dentin blocks for each tooth (n = 10 samples per group).
The composite-dentin blocks of the non-aged and the mechanically aged groups were subsequently broken in order to create failed bonded interfaces ( Fig. 1 b): The composite-dentin samples (3.2 × 3.2 × 8 mm) were fixed onto polystyrene bars (3.2 × 3.2 × 25 mm) (Stripstyrene, Item 32 N o . 176, 0.100 × 0.125″, Evergreen scale models, Kirkland, WA 98034), with the adhesive interface placed in the middle of the bar. Subsequently, the samples were subjected to 3-point flexural loading to promote the fracture of the composite-dentin interface, using an Universal Testing Machine (Materials Testing Machine LS1, Lloyd Materials Testing, Hampshire, UK) at 1 kN, and 1 mm/min cross speed. The stylus was positioned on the bar exactly above the interface, thus promoting the fracture of composite-dentin interface. The polystyrene bar remained intact during this procedure, and the dentin and composite blocks, now with an interfacial gap, remained attached to the bar. The composite blocks were removed from the bar, after fracturing, and repositioned onto the polystyrene bars with a plastic matrix of 200 μm between it and the dentin, in order to create a fixed interfacial gap of 200 μm. Afterwards, the plastic matrix was removed.
The load at fracture for each sample was recorded and the bond strength (σ) in MPa was obtained with the formula σ = F/A, where F = load for specimen rupture (in Newton) and A = bonded area (mm 2 ). To determine the area, the formula to calculate A (10.4 mm 2 ) = width (3.2 mm) × height (3.2 mm) was employed.
The broken samples were observed in stereomicroscope (40 x magnification) and baseline microradiographs (see later) were assessed to categorize the type of fracture. Only samples with adhesive fractures were included in the cariogenic challenge (microcosm model) test for further analysis. Samples showing mixed or cohesive failures were discarded, as the focus of this study was evaluating the behaviour of the adhesively failed restoration interface with respect to caries development.
2.2.2
Non-bonded samples
Fifteen teeth were used to produce non-bonded samples with artificial gap interfaces ( Fig. 1 c). Adhesive material was applied on the dentin wall using one of the two adhesive systems (adhesive on dentin) or no adhesive material was applied at all (no adhesive) (n = 5 teeth per group).
Dentin blocks of 3.2 × 3.2 × 4 mm dimensions (n = 10 samples per group) were prepared using a low speed diamond saw under continuous water-cooling and were fixed onto polystyrene bars (3.2 × 3.2 × 25 mm). For the groups with the adhesive on dentin, the adhesive systems were applied on the dentin surface according the manufacturers’ instructions. For the others no adhesive system was applied.
The polystyrene bars mounted dentin samples were secured in a vice, and a plastic matrix of 200 μm thickness was placed to create composite-dentin gaps. The dentin blocks mounted on the polystyrene bars with the matrix in position were restored with resin composite material (Clearfil™AP-X, Kuraray) parallel to the dentin wall, creating composite resin blocks (3.2 × 3.2 × 4 mm) using a mould. Afterwards, the plastic matrix was removed.
2.3
Cariogenic challenge
All samples, fixed on the polystyrene bars, were kept moist in deionized distilled water, and subsequently submitted to sterilization: the samples were placed at 3 cm from the radiation source and sterilized with gamma irradiation from a cobalt-60 source with particle energies of 1.25 MeV and submitted to 533.53 Gy/min, with a total dose of 4.08 KGy (Theratronics, Eldorado 78, Best Theratronic LTDA, Ottawa, Canada).
Subsequently, the samples were submitted to cariogenic challenge using a microcosm biofilm model previously described elsewhere . Fresh stimulated saliva (±20 mL; Parafilm “M” ® , American National CanTM, Chicago, IL, USA) was collected from a healthy donor (female, 24 age) in the morning. The donor had abstained from oral hygiene procedures for 24 h prior to saliva collection.
The sterilized samples were transferred aseptically into sterile wells (24-well tissue culture plate; TPP – Techno Plastic Products, Trasadingen, SU) and 0.4 mL of homogenized saliva was dripped in each gap of the samples. After 1 h incubation at 37 °C, the saliva was gently removed out from the wells and 1.8 mL of previously prepared DMM + s was added in each well. After 6 h the growth medium was replaced for DMM without sucrose. Defined medium enriched with mucin (DMM) medium was used as artificial saliva . For the cariogenic challenge, sucrose was added to the medium at a concentration of 1% (DDM + s). The caries following cycling regimen was performed for 14 days: the samples received DMM +s during 6 h (final pH of DMM +s medium = 4.7 ± 0.10), were washed with distilled water and subsequently received DMM without sucrose for 18 h (final pH of DMM medium = 7.4 ± 0.05). The medium was replaced daily . Biofilms were formed independently on the samples under anaerobic condition (80% N 2 , 10% CO 2 and 10% H 2 ) and controlled temperature (37 °C). Analysis of the biofilm acidity was carried out daily using pH measurements (Quimis 50w; Quimis Scientific Instruments, Diadema, SP, Brazil) and V621 electrode (Analion, Ribeirão Preto, SP, Brazil).
2.4
Film processing and image measurements
Caries wall lesion development in the dentin at the interfaces was evaluated using Transversal Wavelength Independent Microradiography (T-WIM). Microradiographs were made at baseline (T0) and after the microcosm cariogenic challenge model (T14). The microradiography settings were 60 kV, 30 mA and an exposure time of 8 s . A step wedge with the same absorption coefficient as the tooth material (94% Al/6% Zn alloy) was used for proper quantitative measurement of lesion depth (LD, μm) and mineral loss (ML, μm.vol%). After exposure, the films were developed (10 min), fixed (7 min), rinsed, and dried. A digital image of each sample was recorded with a light microscope (Leica Microsystems, Wetzlar, Germany) with a magnification of 10× and a CMOS camera (Canon EOS 50D, Tokyo, Japan).
Lesion depth and mineral loss for T-WIM were measured using thresholds of 8% (tissue edge) and 43.2% (sound) mineral for dentin. Each sample was measured with a software program (T-WIM calculation programme, version 5.25, J.de Vries, Groningen, NL) at one location: 200 μm distance from the entrance of the gap. Baseline measurements (T0) were subtracted from measurements after 14 days (T14) and subtracted values were used in the statistical analysis.
2.5
Statistical analysis
The correlation between mineral loss and lesion depth was subjected to Pearson’s Correlation analysis. The effect of interface conditions and adhesive materials on both lesion severity outcomes (mineral loss – ML, μm.vol%; and lesion depth – LD, μm) and the effect of ageing and adhesive materials on bond strength values (MPa) were analyzed using linear regression models. All tests were conducted using the statistical software package SPSS (Statistical Package for Social Sciences, version 22.0, Chicago, IL, USA) and the statistical significance was set at p < 0.05.
2
Materials and methods
2.1
Study design
This was an in vitro study evaluating different composite-dentin interface conditions (failed bond broken after no ageing or mechanical ageing, or non-bonded with or without adhesive on dentin) and adhesive materials (etch-and-rinse and self-etch techniques). A microcosm biofilm model was used to create caries-like lesions, during 14 days. Outcome variables were dentin wall lesion depth and mineral loss.
2.2
Selection and preparation of teeth
Thirty-five freshly extracted sound human molars were selected, cleaned and stored in water. Flat midcoronal dentin surfaces were exposed by enamel removal using #150 grit SiC paper (Siawat Abrasives, Frauenfeld, Switzerland), under running water. Complete removal of enamel was confirmed by stereomicroscopic examination. Subsequently, the dentin surfaces were polished using #600 grit SiC paper (Siawat Abrasives, Frauenfeld, Switzerland).
Two bonding materials, a two-step self-etch adhesive (Clearfil™ SE-Bond – CSE, Kuraray Medical Inc., Tokyo, Japan) and an universal adhesive system applied with etch-and-rinse technique (Scotchbond™ Universal – SU, 3 M ESPE, St. Paul, MN, USA), were applied on the dentin surface according to the manufacturers’ instructions. The bonding materials were light-cured for 10 s using a LED curing device, with an intensity of ≈900 mW/cm 2 (Fusion™ S7 Curing Light, DentLight Inc., Richardon, USA). The restorations were made with a composite resin (Clearfil™ AP-X, Kuraray Medical Inc., Tokyo, Japan). Each composite increment was light-cured for 20 s.
Fig. 1 illustrates schematically the steps of the study. One trained operator performed all adhesive and restorative procedures. The samples were prepared in different ways using the two bonding materials and the composite resin, in order to produce different composite-dentin interfaces. All groups were made to include an interfacial gap, resulting in the following seven experimental groups:
Failed bonded, no ageing (CSE/SU)
Failed bonded, after mechanical ageing (CSE/SU)
Non-bonded, with adhesive material on the dentin (CSE/SU)
Non-bonded, no adhesive material on the dentin.
2.2.1
Failed bonded samples
Twenty teeth were used to produce failed bonded samples ( Fig. 1 a). The prepared teeth were embedded in acrylic resin (16 mm high), leaving the prepared occlusal dentin surface free. These samples were optimally bonded with one of the two adhesive materials (n = 10 teeth), and composite resin restorations were built up to ±4 mm in height. After the restorative procedures, the samples remained stored in distilled water for 24 h, at 37 °C. Subsequently, the samples were randomly divided according to the ageing conditions (n = 5 teeth): no ageing (control – 24 h in distilled water) or mechanical loading ageing. Mechanical loading was performed using a Rub&Roll loading device (30 N of force, 0.4 Hz) for three weeks, resulting in 750.000 mechanical cycles. The Rub&Roll device used for applying mechanical loading is described in detail elsewhere . The mechanical loading was performed at room temperature in water, which was weekly changed.
The ageing was performed before sectioning on whole restored teeth. All restored teeth were sectioned into rectangular composite-dentin samples with an approximate cross-sectional adhesive area of 10.4 mm 2 (3.2 × 3.2 × 8 mm), using a low speed diamond saw under continuous water-cooling. This resulted in the generation of two composite-dentin blocks for each tooth (n = 10 samples per group).
The composite-dentin blocks of the non-aged and the mechanically aged groups were subsequently broken in order to create failed bonded interfaces ( Fig. 1 b): The composite-dentin samples (3.2 × 3.2 × 8 mm) were fixed onto polystyrene bars (3.2 × 3.2 × 25 mm) (Stripstyrene, Item 32 N o . 176, 0.100 × 0.125″, Evergreen scale models, Kirkland, WA 98034), with the adhesive interface placed in the middle of the bar. Subsequently, the samples were subjected to 3-point flexural loading to promote the fracture of the composite-dentin interface, using an Universal Testing Machine (Materials Testing Machine LS1, Lloyd Materials Testing, Hampshire, UK) at 1 kN, and 1 mm/min cross speed. The stylus was positioned on the bar exactly above the interface, thus promoting the fracture of composite-dentin interface. The polystyrene bar remained intact during this procedure, and the dentin and composite blocks, now with an interfacial gap, remained attached to the bar. The composite blocks were removed from the bar, after fracturing, and repositioned onto the polystyrene bars with a plastic matrix of 200 μm between it and the dentin, in order to create a fixed interfacial gap of 200 μm. Afterwards, the plastic matrix was removed.
The load at fracture for each sample was recorded and the bond strength (σ) in MPa was obtained with the formula σ = F/A, where F = load for specimen rupture (in Newton) and A = bonded area (mm 2 ). To determine the area, the formula to calculate A (10.4 mm 2 ) = width (3.2 mm) × height (3.2 mm) was employed.
The broken samples were observed in stereomicroscope (40 x magnification) and baseline microradiographs (see later) were assessed to categorize the type of fracture. Only samples with adhesive fractures were included in the cariogenic challenge (microcosm model) test for further analysis. Samples showing mixed or cohesive failures were discarded, as the focus of this study was evaluating the behaviour of the adhesively failed restoration interface with respect to caries development.
2.2.2
Non-bonded samples
Fifteen teeth were used to produce non-bonded samples with artificial gap interfaces ( Fig. 1 c). Adhesive material was applied on the dentin wall using one of the two adhesive systems (adhesive on dentin) or no adhesive material was applied at all (no adhesive) (n = 5 teeth per group).
Dentin blocks of 3.2 × 3.2 × 4 mm dimensions (n = 10 samples per group) were prepared using a low speed diamond saw under continuous water-cooling and were fixed onto polystyrene bars (3.2 × 3.2 × 25 mm). For the groups with the adhesive on dentin, the adhesive systems were applied on the dentin surface according the manufacturers’ instructions. For the others no adhesive system was applied.
The polystyrene bars mounted dentin samples were secured in a vice, and a plastic matrix of 200 μm thickness was placed to create composite-dentin gaps. The dentin blocks mounted on the polystyrene bars with the matrix in position were restored with resin composite material (Clearfil™AP-X, Kuraray) parallel to the dentin wall, creating composite resin blocks (3.2 × 3.2 × 4 mm) using a mould. Afterwards, the plastic matrix was removed.
2.3
Cariogenic challenge
All samples, fixed on the polystyrene bars, were kept moist in deionized distilled water, and subsequently submitted to sterilization: the samples were placed at 3 cm from the radiation source and sterilized with gamma irradiation from a cobalt-60 source with particle energies of 1.25 MeV and submitted to 533.53 Gy/min, with a total dose of 4.08 KGy (Theratronics, Eldorado 78, Best Theratronic LTDA, Ottawa, Canada).
Subsequently, the samples were submitted to cariogenic challenge using a microcosm biofilm model previously described elsewhere . Fresh stimulated saliva (±20 mL; Parafilm “M” ® , American National CanTM, Chicago, IL, USA) was collected from a healthy donor (female, 24 age) in the morning. The donor had abstained from oral hygiene procedures for 24 h prior to saliva collection.
The sterilized samples were transferred aseptically into sterile wells (24-well tissue culture plate; TPP – Techno Plastic Products, Trasadingen, SU) and 0.4 mL of homogenized saliva was dripped in each gap of the samples. After 1 h incubation at 37 °C, the saliva was gently removed out from the wells and 1.8 mL of previously prepared DMM + s was added in each well. After 6 h the growth medium was replaced for DMM without sucrose. Defined medium enriched with mucin (DMM) medium was used as artificial saliva . For the cariogenic challenge, sucrose was added to the medium at a concentration of 1% (DDM + s). The caries following cycling regimen was performed for 14 days: the samples received DMM +s during 6 h (final pH of DMM +s medium = 4.7 ± 0.10), were washed with distilled water and subsequently received DMM without sucrose for 18 h (final pH of DMM medium = 7.4 ± 0.05). The medium was replaced daily . Biofilms were formed independently on the samples under anaerobic condition (80% N 2 , 10% CO 2 and 10% H 2 ) and controlled temperature (37 °C). Analysis of the biofilm acidity was carried out daily using pH measurements (Quimis 50w; Quimis Scientific Instruments, Diadema, SP, Brazil) and V621 electrode (Analion, Ribeirão Preto, SP, Brazil).
2.4
Film processing and image measurements
Caries wall lesion development in the dentin at the interfaces was evaluated using Transversal Wavelength Independent Microradiography (T-WIM). Microradiographs were made at baseline (T0) and after the microcosm cariogenic challenge model (T14). The microradiography settings were 60 kV, 30 mA and an exposure time of 8 s . A step wedge with the same absorption coefficient as the tooth material (94% Al/6% Zn alloy) was used for proper quantitative measurement of lesion depth (LD, μm) and mineral loss (ML, μm.vol%). After exposure, the films were developed (10 min), fixed (7 min), rinsed, and dried. A digital image of each sample was recorded with a light microscope (Leica Microsystems, Wetzlar, Germany) with a magnification of 10× and a CMOS camera (Canon EOS 50D, Tokyo, Japan).
Lesion depth and mineral loss for T-WIM were measured using thresholds of 8% (tissue edge) and 43.2% (sound) mineral for dentin. Each sample was measured with a software program (T-WIM calculation programme, version 5.25, J.de Vries, Groningen, NL) at one location: 200 μm distance from the entrance of the gap. Baseline measurements (T0) were subtracted from measurements after 14 days (T14) and subtracted values were used in the statistical analysis.
2.5
Statistical analysis
The correlation between mineral loss and lesion depth was subjected to Pearson’s Correlation analysis. The effect of interface conditions and adhesive materials on both lesion severity outcomes (mineral loss – ML, μm.vol%; and lesion depth – LD, μm) and the effect of ageing and adhesive materials on bond strength values (MPa) were analyzed using linear regression models. All tests were conducted using the statistical software package SPSS (Statistical Package for Social Sciences, version 22.0, Chicago, IL, USA) and the statistical significance was set at p < 0.05.
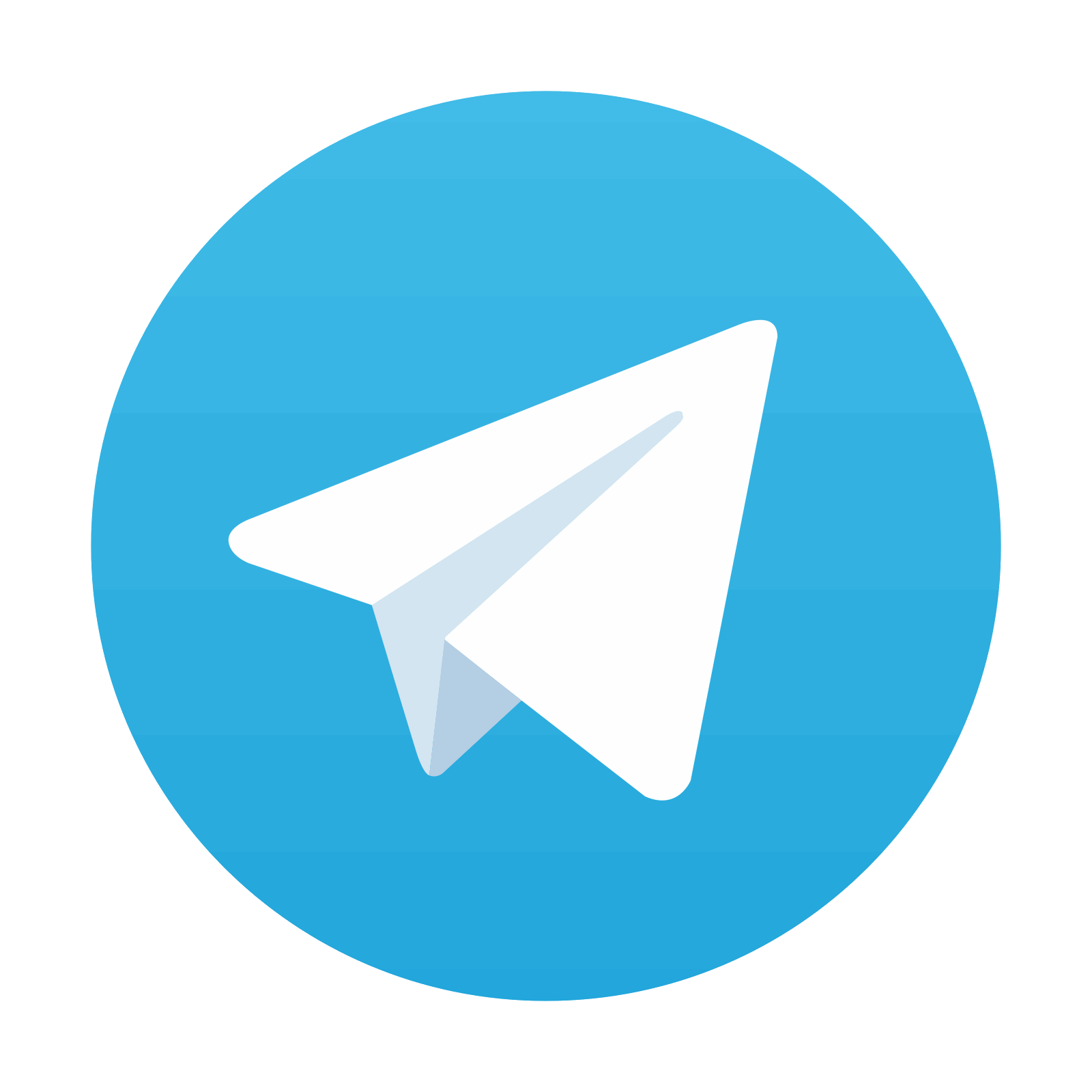
Stay updated, free dental videos. Join our Telegram channel

VIDEdental - Online dental courses
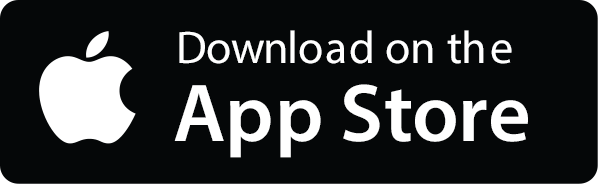
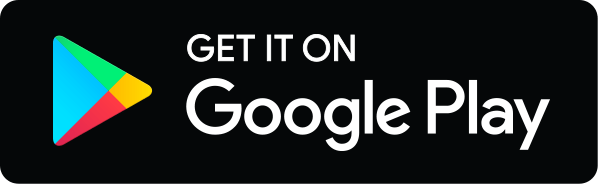