Abstract
Objectives
The objective of the present study was to investigate the long-term effect of 0.01% acrolein (ACR) aqueous solution, employed as an additional primer, on the mechanical durability and enzymatic activity of resin-dentine interfaces created with a simplified etch-and-rinse adhesive.
Methods
Dentine surfaces were etched with 35% phosphoric acid for 15 s, rinsed and blot-dried. Specimens were then assigned to: Group 1: dentine pre-treated with 0.01% ACR aqueous solution for 1 min and bonded with Adper Scotchbond 1 XT (SB1XT), a 2-step etch-and-rinse adhesive; Group 2: SB1XT was applied on untreated acid-etched dentine (control). Resin composite build-ups were made using Filtek Z250. Microtensile bond strength was tested by stressing sectioned specimens to failure immediately or after 1 year of storage in artificial saliva at 37 °C. Zymography and in-situ zymography assays were performed for examining dentine matrix metalloproteinase (MMP) activities.
Results
The use of 0.01% ACR as conditioning primer appeared to have contributed better to preservation of bond strength over time without affecting immediate bond strength. Zymography and in-situ zymography showed reduction in MMP activities after the application of ACR.
Conclusion
Dentine collagen cross-linking produced by an ACR-based primer increases the longevity of resin-dentine bonds by reinforcement of the adhesive interface and reduction of dentine MMP activities. Further studies are required to evaluate the potential in vivo and in vivo cytotoxicity of ACR.
Clinical significance
The acrolein-based primer is a potentially useful clinical bonding tool because it demonstrates good collagen cross-linking ability within a clinically-acceptable working time. Although a low ACR concentration was employed in the present study, the cytotoxicity of ACR should be tested prior to clinical use.
1
Introduction
The stability and integrity of collagen fibrils within the hybrid layer is crucial for the maintenance of long-term bonding effectiveness in adhesive dentistry . Type I collagen accounts for approximately 90% of the dentine organic matrix . The collagen fibrillar network forms the organic framework of dentine within which intrafibrillar and extrafibrillar apatite crystallites are deposited. Matrix metalloproteinases (MMPs) are zinc- and calcium-dependent endopeptidases that are trapped within the mineralised dentine matrix during tooth development . These endogenous enzymes are responsible for in vitro and in vivo degradation of the hybrid layer, via hydrolysis of collagen fibrils that are not completely encapsulated by polymerised adhesive resins . Disruption of resin-dentine integrity caused by the degradation of the hybrid layer accounts for the loss of bond strength over time.
Different strategies have been proposed to minimise degradation of the hybrid layer over time. These strategies include reinforcement of the collagen fibrils within the hybrid layer, inhibition/inactivation of endogenous enzymes, or a combination of these two strategies . Based on the premises that native cross-links improve the tensile properties of collagen fibrils and increase the resistance of a collagen matrix against enzymatic degradation , different natural and chemical cross-linking agents have been used experimentally to increase cross-linking of a demineralised collagen matrix prior to adhesive application . Collagen cross-linking agents have the ability to reinforce the collagen fibrils network by inducing intra- and intermolecular cross-links; recently published studies showed that some of these agents are also capable of inactivating dentinal MMPs .
Aldehydes such as glutaraldehyde stabilise collagen fibrils in several connective tissues and improve the mechanical properties of dentine . Acrolein (2-propenal; ACR), the simplest unsaturated aldehyde, has been used for controlling aquatic weeds in irrigation canals, as a burrow fumigant to control rodents, and as a microbiocide to eliminate slime-forming microbes in oil drilling operations, pulp and paper mills . Similar to glutaraldehyde, the electrophilic ACR is a strong cross-linking agent of cellular components such as proteins , forming carbonyl-retaining Michael adducts with protein molecules that may be attacked by adjacent protein nucleophiles to form intermolecular cross-links . Hence, it is anticipated that ACR, when used in a diluted concentration, may have potential use as collagen cross-linker in dentine bonding involving the etch-and-rinse technique. Accordingly, the objective of the present study was to investigate the long-term effect of the use of 0.01 ACR wt% aqueous solution as an additional primer, on the mechanical durability of resin-bonded dentine created with a simplified etch-and-rinse adhesive. Zymography of dentine extracts and in-situ zymography of resin-dentine interfaces were additionally performed to analyse the potential inhibition effect of ACR on dentinal MMPs. The null hypotheses tested were that the use of ACR as a collagen cross-linker: 1) has no effect on bond strength deterioration over time and 2) has no effect on inactivation of endogenous dentine MMPs.
2
Materials and methods
2.1
Microtensile bond strength
Thirty-six extracted non-carious sound human third molars were collected after the patients’ informed consents were obtained under a protocol approved by the institutional review board of the University of Bologna, Italy. Tooth crowns were removed with a low-speed diamond saw under water irrigation (Micromet, Remet; Bologna, Italy) to expose coronal dentine that was devoid of occlusal enamel. A standardised smear layer was created on the middle/deep coronal dentine with 180-grit wet silicon carbide paper. The exposed dentine surfaces were etched with 35% phosphoric acid for 15 s (etching gel, 3 M ESPE; St Paul, MN, USA), rinsed with water, gently air-dried and kept moist until the adhesive was applied using the wet-bonding technique. The teeth were divided and randomly assigned to 2 treatment groups (n = 18). In group 1 (experimental), the acid-etched dentine was pre-treated with 0.01 ACR wt% aqueous solution (MilliporeSigma, St, Louis, MO, USA) for 1 min, gently air-dried and bonded with Adper Scotchbond 1XT (3 M ESPE) in accordance with the manufacturer’s instructions. The composition of the adhesive is shown in Table 1 . In group 2 (control), Adper Scotchbond 1XT was applied directly on the etched dentine in accordance with manufacturer’s instructions. In both groups, the adhesive was light-cured (Curing Light 2500; 3 M ESPE) for 20 s after solvent evaporation. Four 1-mm-thick layers of a microhybrid resin composite (Filtek Z250, 3 M ESPE) were incrementally placed over the bonded dentine surface and individually polymerised for 20 s to obtain a final 4 mm-thick composite build-up for microtensile bond strength (μTBS) testing.
Composition | |
---|---|
Etching : | 35% H 3 PO 4 |
Adhesive : | dimethacrylates, 2-hydroxyethyl methacrylate, polyalkenoic acid copolymer, 5 nm silane-treated colloidal silica, ethanol, water, photoinitiator |
Resin-dentine sticks were created with a cross-sectional area of approximately 1 mm × 1 mm from each bonded tooth using the low-speed saw under water irrigation, in accordance with the protocol for the non-trimming μTBS technique. The dimension of each stick was measured with a pair of digital callipers (±0.01 mm), and the bonded area was calculated for subsequent conversion of μTBS values into units of stress (MPa). Sticks from each tooth were randomly assigned to two storage groups. For time 0 (T0), the sticks were stored in artificial saliva for 24 h at 37 °C; for time 1 year (T1yr), the sticks were stored for 1 year in artificial saliva at 37 °C.
After storage, the bonded beams were stressed to failure using a simplified universal testing machine (Bisco Inc., Schaumburg, IL, USA) at a crosshead speed of 1 mm/min. Each specimen was observed under a stereomicroscope (Stemi 2000-C; Carl Zeiss Jena GmbH, Göttingen, Germany) at 50× magnification to determine the mode of failure, which was classified as adhesive (A), cohesive in composite (CC) or cohesive in dentine (CD).
Analysis was performed using the tooth as the statistical unit; bond strength data from each tooth were averaged to obtain the mean bond strength for that tooth. The acquired data (n = 18) were evaluated for compliance with the normality (Shapiro-Wilk test) and equality of variance (modified Levine test) assumptions required for parametric statistical analysis. Because these assumptions were not violated, the data were analysed with a two-factor analysis of variance, to examine the effects of “with/without ACR” and “storage time” on μTBS. Post-hoc pairwise comparisons were conducted using the Holm-Sidak method. For all analyses, statistical significance were set at α = 0.05.
2.2
Zymography of dentine extracts
Zymography was performed using the method employed by Mazzoni et al. . Mineralised dentin powder was obtained from additional eight human third molars by freezing the dentine in liquid nitrogen and triturating it using a Retsch mill (Model MM400, Retsch GmbH, Haan, Germany). Aliquots of mineralised dentine powder were divided in 2 groups. For group 1, the dentine powder was demineralised with 37% phosphoric acid to simulate the etching procedure used in the application of an etch-and-rinse adhesive. For group 2, the dentine powder was demineralised in the same manner as group 1 and then treated with 0.01 wt% ACR solution at 4 °C for 30 min. For the control, after demineralisation with 37% phosphoric acid, the dentine powder was incubated with 2 mM of 1,10-phenanthroline at 4 °C for 30 min.
After the aforementioned treatments, the dentine powder aliquots were suspended in extraction buffer (50 mM Tris-HCl, pH 6, containing 5 mM CaCl 2 , 100 mM NaCl, 0.1% Triton X-100, 0.1% non-ionic detergent P-40, 0.1 mM ZnCl 2 and 0.02% NaN 3 ) overnight at 4 °C. The powders were subsequently sonicated for 10 min (at ≈ 30 pulses) and centrifuged for 20 min at 4 °C (20,800X G); the supernatants were retrieved and re-centrifuged. The protein content in the supernatants was concentrated using Vivaspin centrifugal concentrator (10,000 KDa cut-off; Vivaspin Sartorius Stedim Biotech, Goettingen, Germany) for 30 min at 25 °C (15,000× G for 3 times). Total protein concentration of the dentine extracts was determined by Bradford assay. Dentine protein aliquots (60 μg) were diluted with Laemmli sample buffer in a 4:1 ratio. Electrophoresis was performed under non-reducing conditions using 10% sodium dodecyl sulphate-polyacrylamide gel (SDS-PAGE) containing 1 mg/mL fluorescent dye-labelled gelatine. Pre-stained low-range molecular weight SDS-PAGE standards (Bio-Rad, Hercules, CA, USA) were used as reference markers. After electrophoresis, the gels were washed for 1 h in 2% Triton X-100, and incubated in zymography activation buffer (50 mmol/L Tris-HCl, 5 mmol/L CaCl 2 , pH 7.4) for 48 h. Proteolytic activity was evaluated and registered with a long-wave ultraviolet light scanner (ChemiDoc Universal Hood, Bio-Rad). Gelatinase activities in the specimens were analysed in duplicate using gelatine zymography. Densitometric evaluation of bands obtained from zymography was performed using the ImageJ software (National Institutes of Health, Bethesda, MD, USA).
2.3
In-situ zymography of resin-dentine interfaces
One millimetre-thick slabs of middle/deep dentine were obtained from extracted human third molars using the low-speed Micromet saw with water-cooling. A standardised smear layer was created on each dentine surface using 280-grit silicon-carbide paper. One surface of each slab was treated with ACR primer and Adper Scotchbond 1XT adhesive as described for μTBS testing. This was followed by a 1-mm build-up with flowable composite (Filtek 250 flow; 3 M ESPE); the composite was polymerised for 40 s using a light-emitting diode light-curing unit (Curing Light 2500; 3 M ESPE). After completion of those procedures, the bonded assemblies were sectioned vertically into 1-mm-thick slabs to expose the adhesive-dentine interface. Each slab was glued to a microscope slide and polished to the thickness of approximately 50 μm. In-situ zymography was performed according to the protocol reported by Mazzoni et al. , using self-quenched fluorescein-conjugated gelatine as the MMP substrate (E- 12055; Molecular Probes, Eugene, OR, USA). Briefly, the fluorescent gelatine mixture was placed over the polished slab on top of the microscopic slide, covered with a coverslip and incubated in a humidified chamber at 37 °C for 12 h. During incubation, the assemblies were prevented from direct contact with water, and were protected from exposure to light. After incubation, the microscopic slides were examined using a confocal laser scanning microscope (excitation wavelength, 488 nm; emission wavelength, 530 nm; Model A1-R; Nikon, Tokyo, Japan). For each assembly, a series of 1 μm-thick two-dimensional images were made to show the hydrolysis of the quenched fluorescein-conjugated gelatine substrate, as indicator of endogenous gelatinolytic enzyme activity. Each image series was subsequently merged into three-dimensional images, providing additional information on the changes in the gelatinolytic activity throughout the depth of the sample. Enzymatic activity was quantified on the images as the integrated density of the fluorescence signals using ImageJ software (National Institutes of Health, Bethesda, MD, USA).
The integrated density of the fluorescence signals was quantified as indicator of the dentine enzymatic activities of the tested groups. Because the data were not normally distributed even after nonlinear transformation, they were analysed using the Mann-Whitney U test, with α = 0.05.
2
Materials and methods
2.1
Microtensile bond strength
Thirty-six extracted non-carious sound human third molars were collected after the patients’ informed consents were obtained under a protocol approved by the institutional review board of the University of Bologna, Italy. Tooth crowns were removed with a low-speed diamond saw under water irrigation (Micromet, Remet; Bologna, Italy) to expose coronal dentine that was devoid of occlusal enamel. A standardised smear layer was created on the middle/deep coronal dentine with 180-grit wet silicon carbide paper. The exposed dentine surfaces were etched with 35% phosphoric acid for 15 s (etching gel, 3 M ESPE; St Paul, MN, USA), rinsed with water, gently air-dried and kept moist until the adhesive was applied using the wet-bonding technique. The teeth were divided and randomly assigned to 2 treatment groups (n = 18). In group 1 (experimental), the acid-etched dentine was pre-treated with 0.01 ACR wt% aqueous solution (MilliporeSigma, St, Louis, MO, USA) for 1 min, gently air-dried and bonded with Adper Scotchbond 1XT (3 M ESPE) in accordance with the manufacturer’s instructions. The composition of the adhesive is shown in Table 1 . In group 2 (control), Adper Scotchbond 1XT was applied directly on the etched dentine in accordance with manufacturer’s instructions. In both groups, the adhesive was light-cured (Curing Light 2500; 3 M ESPE) for 20 s after solvent evaporation. Four 1-mm-thick layers of a microhybrid resin composite (Filtek Z250, 3 M ESPE) were incrementally placed over the bonded dentine surface and individually polymerised for 20 s to obtain a final 4 mm-thick composite build-up for microtensile bond strength (μTBS) testing.
Composition | |
---|---|
Etching : | 35% H 3 PO 4 |
Adhesive : | dimethacrylates, 2-hydroxyethyl methacrylate, polyalkenoic acid copolymer, 5 nm silane-treated colloidal silica, ethanol, water, photoinitiator |
Resin-dentine sticks were created with a cross-sectional area of approximately 1 mm × 1 mm from each bonded tooth using the low-speed saw under water irrigation, in accordance with the protocol for the non-trimming μTBS technique. The dimension of each stick was measured with a pair of digital callipers (±0.01 mm), and the bonded area was calculated for subsequent conversion of μTBS values into units of stress (MPa). Sticks from each tooth were randomly assigned to two storage groups. For time 0 (T0), the sticks were stored in artificial saliva for 24 h at 37 °C; for time 1 year (T1yr), the sticks were stored for 1 year in artificial saliva at 37 °C.
After storage, the bonded beams were stressed to failure using a simplified universal testing machine (Bisco Inc., Schaumburg, IL, USA) at a crosshead speed of 1 mm/min. Each specimen was observed under a stereomicroscope (Stemi 2000-C; Carl Zeiss Jena GmbH, Göttingen, Germany) at 50× magnification to determine the mode of failure, which was classified as adhesive (A), cohesive in composite (CC) or cohesive in dentine (CD).
Analysis was performed using the tooth as the statistical unit; bond strength data from each tooth were averaged to obtain the mean bond strength for that tooth. The acquired data (n = 18) were evaluated for compliance with the normality (Shapiro-Wilk test) and equality of variance (modified Levine test) assumptions required for parametric statistical analysis. Because these assumptions were not violated, the data were analysed with a two-factor analysis of variance, to examine the effects of “with/without ACR” and “storage time” on μTBS. Post-hoc pairwise comparisons were conducted using the Holm-Sidak method. For all analyses, statistical significance were set at α = 0.05.
2.2
Zymography of dentine extracts
Zymography was performed using the method employed by Mazzoni et al. . Mineralised dentin powder was obtained from additional eight human third molars by freezing the dentine in liquid nitrogen and triturating it using a Retsch mill (Model MM400, Retsch GmbH, Haan, Germany). Aliquots of mineralised dentine powder were divided in 2 groups. For group 1, the dentine powder was demineralised with 37% phosphoric acid to simulate the etching procedure used in the application of an etch-and-rinse adhesive. For group 2, the dentine powder was demineralised in the same manner as group 1 and then treated with 0.01 wt% ACR solution at 4 °C for 30 min. For the control, after demineralisation with 37% phosphoric acid, the dentine powder was incubated with 2 mM of 1,10-phenanthroline at 4 °C for 30 min.
After the aforementioned treatments, the dentine powder aliquots were suspended in extraction buffer (50 mM Tris-HCl, pH 6, containing 5 mM CaCl 2 , 100 mM NaCl, 0.1% Triton X-100, 0.1% non-ionic detergent P-40, 0.1 mM ZnCl 2 and 0.02% NaN 3 ) overnight at 4 °C. The powders were subsequently sonicated for 10 min (at ≈ 30 pulses) and centrifuged for 20 min at 4 °C (20,800X G); the supernatants were retrieved and re-centrifuged. The protein content in the supernatants was concentrated using Vivaspin centrifugal concentrator (10,000 KDa cut-off; Vivaspin Sartorius Stedim Biotech, Goettingen, Germany) for 30 min at 25 °C (15,000× G for 3 times). Total protein concentration of the dentine extracts was determined by Bradford assay. Dentine protein aliquots (60 μg) were diluted with Laemmli sample buffer in a 4:1 ratio. Electrophoresis was performed under non-reducing conditions using 10% sodium dodecyl sulphate-polyacrylamide gel (SDS-PAGE) containing 1 mg/mL fluorescent dye-labelled gelatine. Pre-stained low-range molecular weight SDS-PAGE standards (Bio-Rad, Hercules, CA, USA) were used as reference markers. After electrophoresis, the gels were washed for 1 h in 2% Triton X-100, and incubated in zymography activation buffer (50 mmol/L Tris-HCl, 5 mmol/L CaCl 2 , pH 7.4) for 48 h. Proteolytic activity was evaluated and registered with a long-wave ultraviolet light scanner (ChemiDoc Universal Hood, Bio-Rad). Gelatinase activities in the specimens were analysed in duplicate using gelatine zymography. Densitometric evaluation of bands obtained from zymography was performed using the ImageJ software (National Institutes of Health, Bethesda, MD, USA).
2.3
In-situ zymography of resin-dentine interfaces
One millimetre-thick slabs of middle/deep dentine were obtained from extracted human third molars using the low-speed Micromet saw with water-cooling. A standardised smear layer was created on each dentine surface using 280-grit silicon-carbide paper. One surface of each slab was treated with ACR primer and Adper Scotchbond 1XT adhesive as described for μTBS testing. This was followed by a 1-mm build-up with flowable composite (Filtek 250 flow; 3 M ESPE); the composite was polymerised for 40 s using a light-emitting diode light-curing unit (Curing Light 2500; 3 M ESPE). After completion of those procedures, the bonded assemblies were sectioned vertically into 1-mm-thick slabs to expose the adhesive-dentine interface. Each slab was glued to a microscope slide and polished to the thickness of approximately 50 μm. In-situ zymography was performed according to the protocol reported by Mazzoni et al. , using self-quenched fluorescein-conjugated gelatine as the MMP substrate (E- 12055; Molecular Probes, Eugene, OR, USA). Briefly, the fluorescent gelatine mixture was placed over the polished slab on top of the microscopic slide, covered with a coverslip and incubated in a humidified chamber at 37 °C for 12 h. During incubation, the assemblies were prevented from direct contact with water, and were protected from exposure to light. After incubation, the microscopic slides were examined using a confocal laser scanning microscope (excitation wavelength, 488 nm; emission wavelength, 530 nm; Model A1-R; Nikon, Tokyo, Japan). For each assembly, a series of 1 μm-thick two-dimensional images were made to show the hydrolysis of the quenched fluorescein-conjugated gelatine substrate, as indicator of endogenous gelatinolytic enzyme activity. Each image series was subsequently merged into three-dimensional images, providing additional information on the changes in the gelatinolytic activity throughout the depth of the sample. Enzymatic activity was quantified on the images as the integrated density of the fluorescence signals using ImageJ software (National Institutes of Health, Bethesda, MD, USA).
The integrated density of the fluorescence signals was quantified as indicator of the dentine enzymatic activities of the tested groups. Because the data were not normally distributed even after nonlinear transformation, they were analysed using the Mann-Whitney U test, with α = 0.05.
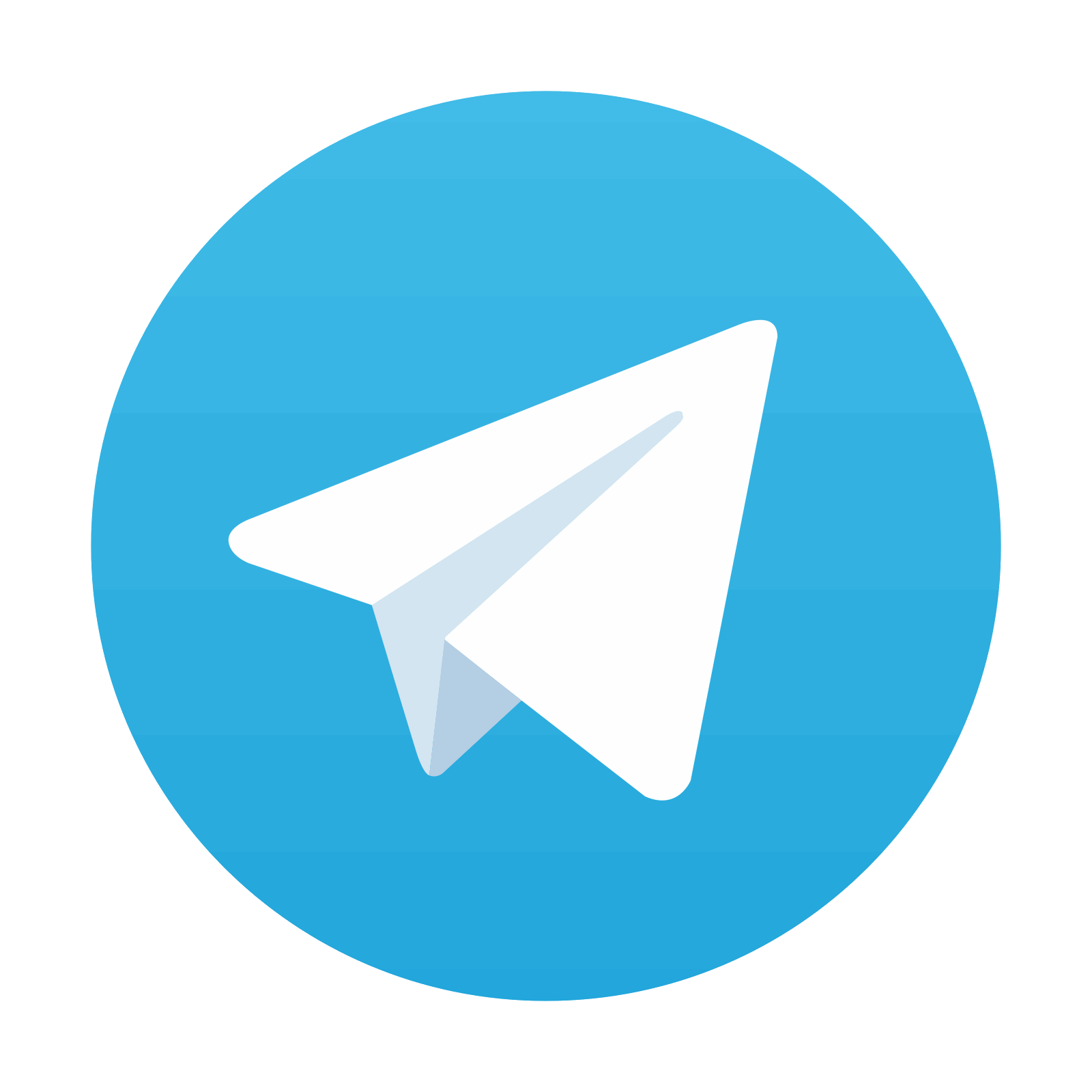
Stay updated, free dental videos. Join our Telegram channel

VIDEdental - Online dental courses
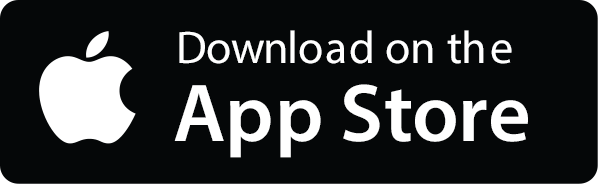
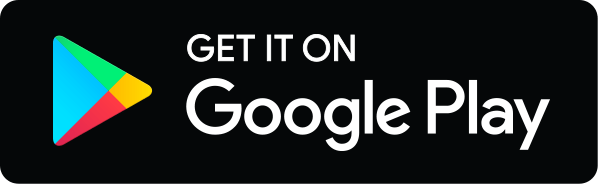