Abstract
Objectives
The aim of this study was to investigate whether Streptococcus mutans and Enterococcus faecalis develop resistance to the cationic biocides chlorhexidine (CHX), cetylpyridinium chloride (CPC), and 12-methacryloyloxydodecylpyridinium bromide (MDPB).
Methods
The minimum inhibitory concentrations (MICs) of CHX, CPC, and MDPB were assessed after repeated exposure of S . mutans and E . faecalis to these biocides. Cell-surface hydrophobicity and protein expression profiles of bacterial cells were examined to elucidate possible resistance mechanisms.
Results
The MIC of CHX against E. faecalis showed constant increases up to 10 passages. No changes in the MICs of CPC and MDPB against E. faecalis were observed. The MICs of CHX, CPC, and MDPB against S. mutans did not increase. The surface hydrophobicity of E. faecalis significantly increased with increasing exposure to CHX and CPC. However, changes in protein expression profiles were only found in CHX-adapted E. faecalis , as evidenced by the emergence of a novel, approximately 19-kDa band following sodium dodecyl sulfate-polyacrylamide gel electrophoresis.
Conclusions
While E. faecalis and S. mutans did not exhibit increased resistance to CPC or MDPB, repeated exposure of E. faecalis to CHX led to resistance. It is likely that the acquisition of resistance is related to an altered protein composition.
Clinical Significance
Alkyl pyridinium compounds, such as CPC and MDPB, could have a lower risk to cause adaptation of E. faecalis , which is advantageous compared with CHX.
1
Introduction
Cationic biocides have been used as disinfectants in a variety of fields, such as the chemical, pharmaceutical, and food industries, as well as in hospitals, homes, and the environment. Among them, chlorhexidine (CHX) and a quaternary ammonium compound (QAC), cetylpyridinium chloride (CPC), are widely used in dentistry to prevent caries and periodontal disease, or as endodontic treatments , because they have a broad antimicrobial spectrum. The antibacterial monomer 12-methacryloyloxydodecylpyridinium bromide (MDPB), which is synthesized by combining a QAC with a methacryloyl group , is also used in a commercial adhesive to exhibit disinfecting effects of tooth substrate containing caries-related bacteria .
Although the strong killing effects of biocides are clinically beneficial, their frequent use could lead to the development of bacterial drug resistance . Regarding non-oral bacteria such as Escherichia coli , a few studies have reported adaptation to CHX and CPC. However, no reports have investigated the possibility that oral bacteria can acquire resistance to cationic biocides.
The aim of this study was to investigate if Streptococcus mutans and Enterococcus faecalis develop resistance to CHX, CPC, and MDPB by measuring their minimum inhibitory concentrations (MICs) after repeated exposure to these biocides. Cell-surface hydrophobicity and protein expression profiles of bacterial cells were also examined to elucidate possible resistance mechanisms.
2
Materials and methods
2.1
Bacterial strains and cationic biocides used
S. mutans UA159 and E. faecalis SS497 were used in this study. Inocula from stock cultures were stored in Microbank vials ® (PRO-LAB Diagnostics, Richmond Hill, ON, Canada) at −80 °C and cultivated in Brain Heart Infusion (BHI; Becton Dickinson, Sparks, MD, USA) broth supplemented with 0.5% yeast extract (Becton Dickinson) for 12 h at 37 °C. Two antimicrobials, CPC and CHX diacetate, were provided by Sigma–Aldrich (St. Louis, MO, USA). MDPB was prepared as described elsewhere .
2.2
MIC measurements
The MICs of the three cationic biocides against S. mutans and E. faecalis were repeatedly measured by modified microdilution methods . 500 μL of an overnight culture of S. mutans or E. faecalis was added to 10 mL of BHI broth to give a concentration of approximately 1 × 10 7 colony-forming units (CFU)/mL. 100 μL of a bacterial suspension was placed into the wells of a 96-well microplate, and 100 μL of a CHX, CPC, or MDPB solution at 1.0–16.0 μg/mL (at increments of 1.0 μg/mL), which was prepared in BHI broth supplemented with 0.5% yeast extract, was added. The addition of BHI broth alone was used as the control. After anaerobic incubation at 37 °C for 24 h, the turbidity of the suspension was visually examined and the MIC was determined. The MIC value was defined as the lowest concentration at which bacterial growth was not observed with showing the same turbidity as the control.
From the bacterial suspensions that demonstrated growth under the highest concentration of each biocide, a 500-μL portion was inoculated into 10 mL of fresh BHI broth to obtain a bacterial concentration of approximately 1 × 10 7 CFU/mL, and the MIC was determined as described previously. MIC determinations were repeatedly performed for 10 passages. For all of the biocides, the tests were conducted in five replicates for each bacterium. Strains that were passaged five and 10 times, which showed growth under the highest concentrations of CHX or CPC, were stored at −80 °C for further experiments.
2.3
Determination of cell-surface hydrophobicity
Cell-surface hydrophobicity of E. faecalis after five or 10 exposures to CHX and CPC was measured by microbial adherence to n -hexadecane, as reported previously . Cells were washed twice and resuspended in phosphate-urea-magnesium sulfate buffer (100 mM sodium phosphate buffer, pH 7.1, 30 mM urea, 0.8 mM MgSO 4 ) to an initial optical density (OD) at 550 nm of 0.55–0.60. 4 mL of the cell suspension was mixed vigorously with 0.5 mL of n -hexadecane for 30 s, and left at room temperature for 10 min. The OD of the lower aqueous phase was then measured at 550 nm. Hydrophobicity was expressed as the percentage of adherence to the hydrocarbon, which was calculated as follows:
where I = the OD of the initial cell suspension, and A = the OD of the aqueous phase.
Hydrophobicities were recorded as the mean of three replicates for each bacterial suspension. Statistical significance was analyzed using analysis of variance (ANOVA) and a Student–Newman–Keuls’ post-hoc test with a significance level of 0.05.
2.4
Analysis of protein expression profiles
Late exponential phase cells grown in BHI broth containing CHX or CPC for five or 10 passages were harvested by centrifugation (2380 × g , 10 min). 500 μL of BugBuster Master Mix ® (Merck, Darmstadt, Germany) was used to resuspend the cell pellet. The cells were disrupted by sonication for 30 s. After rotation for 2 h at 4 °C, the mixture was centrifuged at 16,000 × g for 20 min at 4 °C to remove debris and unbroken cells. The concentrations of proteins in the supernatant were measured using a modified Bradford method , and the concentration of the extracted proteins was adjusted to 155 μg/mL. 50 μL of NuPAGE SDS Sample Buffer ® and 20 μL of a NuPAGE Sample Reducing Agent ® (Invitrogen, Carlsbad, CA, USA) were added to 150 μL of the supernatant. The mixture was incubated at 70 °C for 10 min and then cooled to room temperature. The protein fractions were analyzed using sodium dodecyl sulfate-polyacrylamide gel electrophoresis as described previously ; the running gel contained 12% (w/v) acrylamide (NuPAGE ® 12% Bis–Tris Gel, Invitrogen). Electrophoresis was conducted at 250 V for 1 h, and the gel was stained with 2.5% Coomassie brilliant blue R 250 (Sigma–Aldrich). The protein expression profiles were visually examined, and the gray values of protein bands in images (8-bit, 256 gray scales) were analyzed using ImageJ software (National Institutes of Health, Bethesda, MD, USA) to compare the darkness of protein bands .
2
Materials and methods
2.1
Bacterial strains and cationic biocides used
S. mutans UA159 and E. faecalis SS497 were used in this study. Inocula from stock cultures were stored in Microbank vials ® (PRO-LAB Diagnostics, Richmond Hill, ON, Canada) at −80 °C and cultivated in Brain Heart Infusion (BHI; Becton Dickinson, Sparks, MD, USA) broth supplemented with 0.5% yeast extract (Becton Dickinson) for 12 h at 37 °C. Two antimicrobials, CPC and CHX diacetate, were provided by Sigma–Aldrich (St. Louis, MO, USA). MDPB was prepared as described elsewhere .
2.2
MIC measurements
The MICs of the three cationic biocides against S. mutans and E. faecalis were repeatedly measured by modified microdilution methods . 500 μL of an overnight culture of S. mutans or E. faecalis was added to 10 mL of BHI broth to give a concentration of approximately 1 × 10 7 colony-forming units (CFU)/mL. 100 μL of a bacterial suspension was placed into the wells of a 96-well microplate, and 100 μL of a CHX, CPC, or MDPB solution at 1.0–16.0 μg/mL (at increments of 1.0 μg/mL), which was prepared in BHI broth supplemented with 0.5% yeast extract, was added. The addition of BHI broth alone was used as the control. After anaerobic incubation at 37 °C for 24 h, the turbidity of the suspension was visually examined and the MIC was determined. The MIC value was defined as the lowest concentration at which bacterial growth was not observed with showing the same turbidity as the control.
From the bacterial suspensions that demonstrated growth under the highest concentration of each biocide, a 500-μL portion was inoculated into 10 mL of fresh BHI broth to obtain a bacterial concentration of approximately 1 × 10 7 CFU/mL, and the MIC was determined as described previously. MIC determinations were repeatedly performed for 10 passages. For all of the biocides, the tests were conducted in five replicates for each bacterium. Strains that were passaged five and 10 times, which showed growth under the highest concentrations of CHX or CPC, were stored at −80 °C for further experiments.
2.3
Determination of cell-surface hydrophobicity
Cell-surface hydrophobicity of E. faecalis after five or 10 exposures to CHX and CPC was measured by microbial adherence to n -hexadecane, as reported previously . Cells were washed twice and resuspended in phosphate-urea-magnesium sulfate buffer (100 mM sodium phosphate buffer, pH 7.1, 30 mM urea, 0.8 mM MgSO 4 ) to an initial optical density (OD) at 550 nm of 0.55–0.60. 4 mL of the cell suspension was mixed vigorously with 0.5 mL of n -hexadecane for 30 s, and left at room temperature for 10 min. The OD of the lower aqueous phase was then measured at 550 nm. Hydrophobicity was expressed as the percentage of adherence to the hydrocarbon, which was calculated as follows:
where I = the OD of the initial cell suspension, and A = the OD of the aqueous phase.
Hydrophobicities were recorded as the mean of three replicates for each bacterial suspension. Statistical significance was analyzed using analysis of variance (ANOVA) and a Student–Newman–Keuls’ post-hoc test with a significance level of 0.05.
2.4
Analysis of protein expression profiles
Late exponential phase cells grown in BHI broth containing CHX or CPC for five or 10 passages were harvested by centrifugation (2380 × g , 10 min). 500 μL of BugBuster Master Mix ® (Merck, Darmstadt, Germany) was used to resuspend the cell pellet. The cells were disrupted by sonication for 30 s. After rotation for 2 h at 4 °C, the mixture was centrifuged at 16,000 × g for 20 min at 4 °C to remove debris and unbroken cells. The concentrations of proteins in the supernatant were measured using a modified Bradford method , and the concentration of the extracted proteins was adjusted to 155 μg/mL. 50 μL of NuPAGE SDS Sample Buffer ® and 20 μL of a NuPAGE Sample Reducing Agent ® (Invitrogen, Carlsbad, CA, USA) were added to 150 μL of the supernatant. The mixture was incubated at 70 °C for 10 min and then cooled to room temperature. The protein fractions were analyzed using sodium dodecyl sulfate-polyacrylamide gel electrophoresis as described previously ; the running gel contained 12% (w/v) acrylamide (NuPAGE ® 12% Bis–Tris Gel, Invitrogen). Electrophoresis was conducted at 250 V for 1 h, and the gel was stained with 2.5% Coomassie brilliant blue R 250 (Sigma–Aldrich). The protein expression profiles were visually examined, and the gray values of protein bands in images (8-bit, 256 gray scales) were analyzed using ImageJ software (National Institutes of Health, Bethesda, MD, USA) to compare the darkness of protein bands .
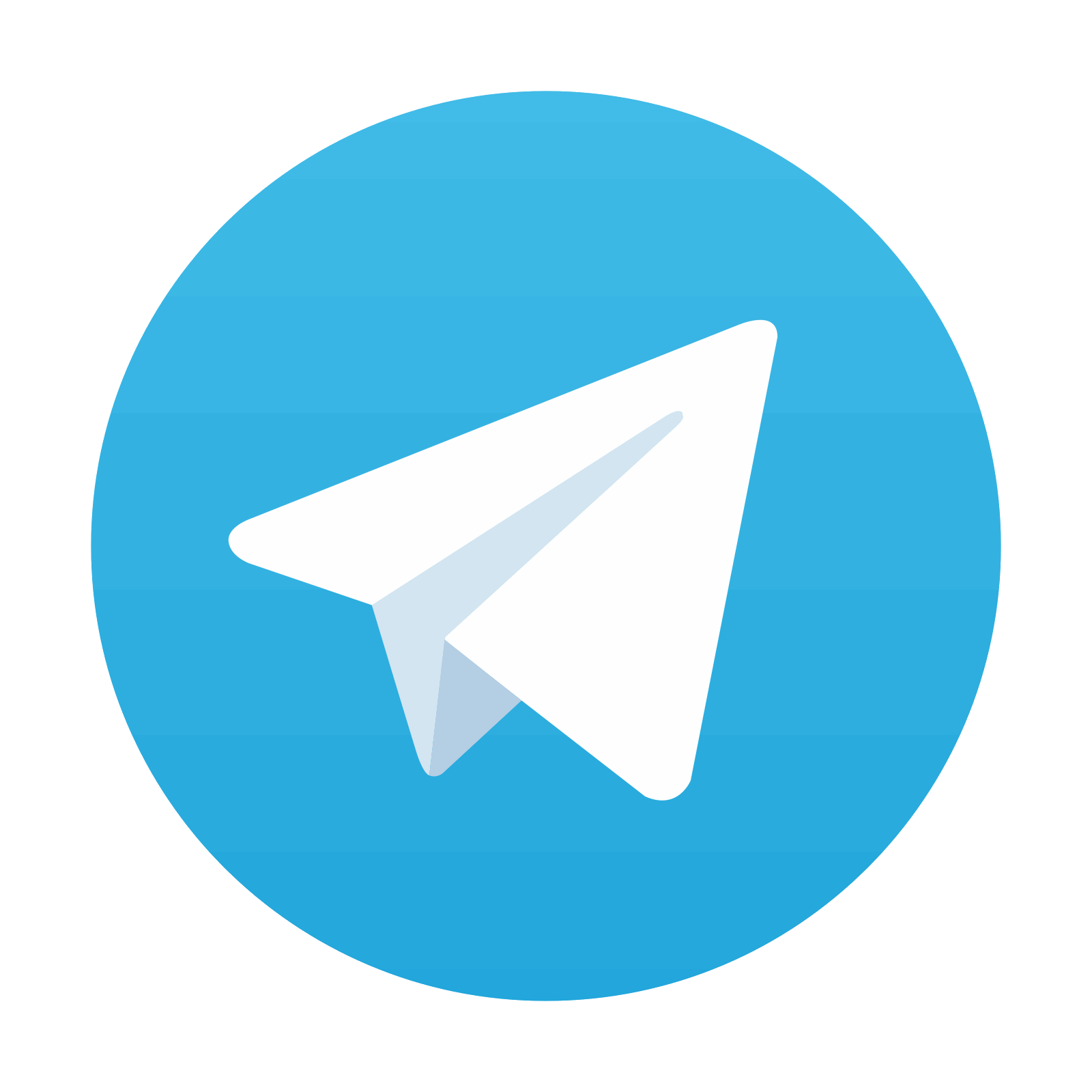
Stay updated, free dental videos. Join our Telegram channel

VIDEdental - Online dental courses
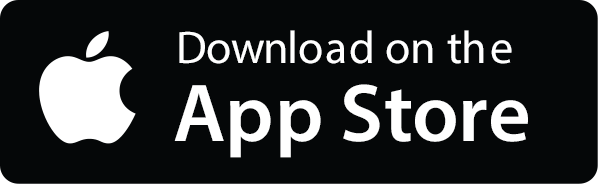
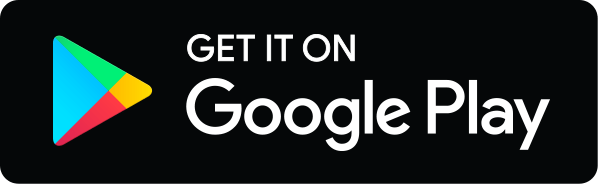