Abstract
Objectives
To investigate the effect of UDMA %, of a range of filled UDMA:TEGDMA resins, on viscosity, degree of conversion and shear bond strength. Furthermore, to compare between model filled and unfilled UDMA adhesives, and clinically used orthodontic adhesives on these properties.
Methods
Four filled and four unfilled resins with a UDMA to TEGDMA weight ratio 50:50, 60:40, 70:30, 80:20 were formulated, tested and compared to the Bis-GMA control Transbond XT. The properties investigated were: viscosity (rotational viscometry), degree of conversion (DC) (FT-IR) and bond strength (shear bond strength test). One-way ANOVA and Tukey post hoc test was used to statistically analyze the data for viscosity and DC% while the non-parametric Kruskal–Wallis and Mann–Whitney U -test was used for the shear bond strength values.
Results
For SBS a comparable bond strength was obtained between the U80:T20(F) adhesive and the control Transbond XT (27.1 and 30.1 respectively). There was no significant difference between the U70:T30 adhesive and the control. Transbond XT (43.1%) had a significantly lower DC% than all the UDMA based adhesives. Furthermore, there was no significant difference between the DC% means of the various UDMA resins. There was a significant decrease in the viscosity for both filled and unfilled groups, as the TEGDMA concentration was increased.
Significance
The results indicate that adhesives formulated with UDMA and TEGDMA monomers, could produce resins with comparable viscosities to the Bis-GMA control, Transbond XT. Adhesives formulated with high UDMA %, can be used to produce resins with greater viscosity and increased bond strength, potentially without affecting their degree of conversion.
1
Introduction
Orthodontic bonding adhesives (OBAs) are used to bond the attachments on the teeth and maintain them fixed for the duration of the treatment. Bis-GMA is the base monomer used in the majority if not all of the currently used orthodontic adhesives.
Recently, the estrogenic potential of Bis-GMA containing dental resins, including orthodontic adhesives, has come into question . The option to employ Bis-GMA free adhesives should be explored. These must be able to substitute conventional Bis-GMA adhesives used for bonding brackets in orthodontics, without compromising their clinical performance.
A series of key properties need to be evaluated. In particular handling and mechanical properties are considered to be critical in orthodontics. The type of monomers used and their ratios can greatly affect the materials viscosity. Furthermore, the incorporation of fillers in the organic matrix can also further modify the adhesives handling . In contrast to Bis-GMA’s rigid benzoic ring, the flexible ester bond located in UDMA’s spacer group, alters the monomers diffusive potential (displaceability), flexibility and intermolecular forces, which in turn directly affect the materials handling .
A material’s behavior of flow is characterized by its viscosity. This feature is associated with the displacement potential of its monomers, through the adhesive, upon the application of a force. This property can be closely related to the materials handling, in regards to its placement on to the bracket, tacking, clean-up, and bracket drifting and slumping. Previous research has provided data regarding the rheological properties of filled/unfilled Bis-GMA based resin formulations, as well as unfilled UDMA based resin formulation. There seems to be no available data delineating the viscosity of a range filled UDMA:TEGDMA resins. Furthermore, the comparison between model UDMA adhesives and clinically used orthodontic adhesives is also not available.
Also, the adhesives degree of conversion (DC%) can be used as an indirect indicator of mechanical properties (under specific limitations) as well as generalized long term stability ; it has also been closely associated with the adhesive’s formulation and viscosity . The resin’s degree of polymerization can be studied using Fourier transform infrared radiation (FT-IR) spectroscopy .
In terms of mechanical properties, the shear bond strength (SBS) test, with acid-etched enamel, is the one used more often in orthodontics. The orthodontic literature consist of a rather large amount of SBS studies investigating mainly the shear bond strengths of commercial and/or experimental OBA as well as the effect of various potential confounding factors.
When comparing standardized resins that only vary in their monomer ratios, those with higher levels of conversion are expected to exhibit greater strength and stability . This also can improve certain physical and biological properties, such as material plasticization, patient sensitivity and biocompatibility .
The aims of the present study were to compare a series of model adhesives with varied UDMA%, with a commercial one on the following properties: (i) viscosity; (ii) DC and (iii) shear bond strength.
The null hypothesis were (i) that UDMA % will have no affect on viscosity, DC, and SBS and (ii) there will be no difference between the model and commercial adhesives on the properties studied.
2
Materials and method
Four filled and four unfilled experimental UDMA-based adhesives were prepared and tested. Each varied according to their UDMA:TEGDMA percentage weight ratio (U 80 :T 20 , U 70 :T 30 , U 60 :T 40 , U 50 :T 50 ) and consisted of a 57% weight filler loading (Optimized Methacrylsilane treated 0.8 μm powder; 1351-900 PST/3, Quarzwerke, Germany), 0.47% weight CQ and 0.45% weight of DMAEMA. Transbond XT (3 M Unitek) was used as the Bis-GMA control. Both monomers were supplied by Röhm, GmbH, Germany and CQ and DMAEMA by Sigma–Aldrich, UK. Transbond XT Primer consists of BisGMA and TEGDMA and Transbond XT Adhesive of BisGMA, Silane, Silica, hexa-fluoro-phosphate and N-dimethyl benzocaine (according to manufacturer’s information).
2.1
Viscosity
A Bohlin rheometer (Bohlin Instruments Ltd, UK; Bohlin Software: GEMINI 150) was used to conduct a steady shear sweep test (shear ramp parameter) in order to measure the adhesives viscosity. The adhesive (approx 3 ml) was first placed on the lower plate and the upper plate was then lowered, until a 1 mm gap was formed and any excess adhesive was extruded from its circumference. The plate was then manually rotated as to have an even spread of the adhesive. Any excess adhesive and any air bubbles present around the circumference of the plate were removed, prior to initiating active testing. A plate of 20 mm in diameter was used for Transbond XT with a gap size of 300 μm, while a plate of 40 mm with a gap size of 100 μm was used for all the rest of the adhesives; the base plate was heated to 25 °C. This difference in plate size and gap width was due to the highly viscous nature of Transbond XT, resulting in the slippage of the rotating upper plate, during testing.
The specimens were placed under increasing torque frequencies (synonymous with increasing shear rates) while the resulting amount of shear stress developing in the resin was measured. The shear stress to shear strain values obtained enabled the calculation of the materials instantaneous viscosity (Eq. (1) ).
where η = viscosity, τ = shear stress, and γ = shear strain rate (i.e. velocity gradient).
Descriptive statistics of the data (i.e. mean, standard deviation and standard error) were calculated for the viscosity readings. Levene’s test statistic was used to assess for homogeneity of variances and a one-way ANOVA was used to compare means, per group, together with Tukey HSD post hoc test statistic at p < 0.05.
2.2
DC%
The DC was measured using an FTIR spectrometer (Avatar 360, Nicolet Analytical Instruments, UK) equipped with a single reflection horizontal ATR accessory (MIRacle ATR, PIKE Technologies, 6125 Cottonwood Drive, Madison). The FTIR spectrometer was operating under the following conditions: 2000–500 cm −1 wavelength, 4 cm −1 resolution, and 32 scans.
The active peak used in all the adhesive samples was the aliphatic C C (peak 1637 cm −1 ). The internal standard varied between the Bis-GMA and UDMA samples. In Transbond XT the aromatic (C⋯C) bond was used (peak 1608 cm −1 ), while in the UDMA based adhesives, the secondary amide bond (NH-II) was used (peak 1527 cm −1 ). The degree of conversion was then calculated by evaluating the proportional change of the polymerizing absorption peak area (i.e. aliphatic C C) area in relation to the internal standard (Eq. (2) ).
where A 1637 = area of active aliphatic C C bond, and A 1527 = area of the internal standard, secondary NH-II bond. The DC% corresponds to a UDMA-based resin. An internal standard was used for all materials. In the case of BisGMA being present, the peak at 1608 cm −1 was used.
Descriptive statistics were used to assess the mean degree of conversion and standard deviation. Levene’s test statistic was used to assess for homogeneity of variances and a one-way ANOVA was used to compare means, per group, together with Tukey HSD post hoc test statistic at p < 0.05.
2.3
SBS
Lower mandibular bovine teeth (crowns) were collected, cleaned of debris, soaked for 24 h in 0.5% (wt/vol) hypochlorite solution and stored in water (frequently refreshed) . The buccal surfaces of the teeth were flattened using carbide abrasive paper (P600) and teeth with any resulting dentin exposure were excluded. The teeth ( n = 70) were then equally divided into the 5 experimental groups according to their size.
24 h prior to testing the crowns were embedded in brass cylinder holders with dental die stone. Their exposed buccal surface gently re-sanded (P600), washed and re-examined prior to bonding. Circular lingual buttons (Item 224-112, TP Orthodontic Inc, UK) were used as the attachment device.
The bonding procedure used, followed the manufacturer’s suggested protocol. The exposed enamel surface was etched for 15 s with 40% phosphoric acid gel (Henry-Schein) and then rinsed for 30 s under pressurized tap water and dried (air dry). A thin layer of Transbond-XT Primer (3 M, Unitek) was applied onto the tooth’s surface for all the adhesives and a small amount of the OBA was placed on the base of the button. A metallic button was then tacked onto the surface of the tooth, gently compressed and any excess flash was removed. The adhesive was then light cured for 40 s using a high intensity soft-start LED light curing unit (Celalux, VOCO) of at least 1500 mW/cm 2 (measured with a high intensity radiometer; Demetron Research Corp.), at 90° angle directly above the button. The bonded specimens were then stored in a dry environment (23 °C), for 24 h.
The shear bond strength test was conducted using a Zwick/Roell Z020 Universal testing machine (Leominster, UK) and the Zwick/Roell TestXpert computer software program.
The testing machine was used with a 500 N loading cell and a crosshead speed used was 0.5 mm/min . The bracket’s ligature groove was used as the loading point. The specimens were loaded until the bracket-adhesive-enamel complex failed.
Shapiro–Wilk’s test and Levene’s test were used to assess for normality and homogeneity, and subsequently the Kruskal–Wallis and Mann–Whitney rank test statistics were applied, with a confidence level of 95% ( p < 0.05).
The bond strength (MPa) was calculated based on the following equation (Eq. (3) ):
where σ = stress, A = nominal surface area of attachment.
2
Materials and method
Four filled and four unfilled experimental UDMA-based adhesives were prepared and tested. Each varied according to their UDMA:TEGDMA percentage weight ratio (U 80 :T 20 , U 70 :T 30 , U 60 :T 40 , U 50 :T 50 ) and consisted of a 57% weight filler loading (Optimized Methacrylsilane treated 0.8 μm powder; 1351-900 PST/3, Quarzwerke, Germany), 0.47% weight CQ and 0.45% weight of DMAEMA. Transbond XT (3 M Unitek) was used as the Bis-GMA control. Both monomers were supplied by Röhm, GmbH, Germany and CQ and DMAEMA by Sigma–Aldrich, UK. Transbond XT Primer consists of BisGMA and TEGDMA and Transbond XT Adhesive of BisGMA, Silane, Silica, hexa-fluoro-phosphate and N-dimethyl benzocaine (according to manufacturer’s information).
2.1
Viscosity
A Bohlin rheometer (Bohlin Instruments Ltd, UK; Bohlin Software: GEMINI 150) was used to conduct a steady shear sweep test (shear ramp parameter) in order to measure the adhesives viscosity. The adhesive (approx 3 ml) was first placed on the lower plate and the upper plate was then lowered, until a 1 mm gap was formed and any excess adhesive was extruded from its circumference. The plate was then manually rotated as to have an even spread of the adhesive. Any excess adhesive and any air bubbles present around the circumference of the plate were removed, prior to initiating active testing. A plate of 20 mm in diameter was used for Transbond XT with a gap size of 300 μm, while a plate of 40 mm with a gap size of 100 μm was used for all the rest of the adhesives; the base plate was heated to 25 °C. This difference in plate size and gap width was due to the highly viscous nature of Transbond XT, resulting in the slippage of the rotating upper plate, during testing.
The specimens were placed under increasing torque frequencies (synonymous with increasing shear rates) while the resulting amount of shear stress developing in the resin was measured. The shear stress to shear strain values obtained enabled the calculation of the materials instantaneous viscosity (Eq. (1) ).
where η = viscosity, τ = shear stress, and γ = shear strain rate (i.e. velocity gradient).
Descriptive statistics of the data (i.e. mean, standard deviation and standard error) were calculated for the viscosity readings. Levene’s test statistic was used to assess for homogeneity of variances and a one-way ANOVA was used to compare means, per group, together with Tukey HSD post hoc test statistic at p < 0.05.
2.2
DC%
The DC was measured using an FTIR spectrometer (Avatar 360, Nicolet Analytical Instruments, UK) equipped with a single reflection horizontal ATR accessory (MIRacle ATR, PIKE Technologies, 6125 Cottonwood Drive, Madison). The FTIR spectrometer was operating under the following conditions: 2000–500 cm −1 wavelength, 4 cm −1 resolution, and 32 scans.
The active peak used in all the adhesive samples was the aliphatic C C (peak 1637 cm −1 ). The internal standard varied between the Bis-GMA and UDMA samples. In Transbond XT the aromatic (C⋯C) bond was used (peak 1608 cm −1 ), while in the UDMA based adhesives, the secondary amide bond (NH-II) was used (peak 1527 cm −1 ). The degree of conversion was then calculated by evaluating the proportional change of the polymerizing absorption peak area (i.e. aliphatic C C) area in relation to the internal standard (Eq. (2) ).
where A 1637 = area of active aliphatic C C bond, and A 1527 = area of the internal standard, secondary NH-II bond. The DC% corresponds to a UDMA-based resin. An internal standard was used for all materials. In the case of BisGMA being present, the peak at 1608 cm −1 was used.
Descriptive statistics were used to assess the mean degree of conversion and standard deviation. Levene’s test statistic was used to assess for homogeneity of variances and a one-way ANOVA was used to compare means, per group, together with Tukey HSD post hoc test statistic at p < 0.05.
2.3
SBS
Lower mandibular bovine teeth (crowns) were collected, cleaned of debris, soaked for 24 h in 0.5% (wt/vol) hypochlorite solution and stored in water (frequently refreshed) . The buccal surfaces of the teeth were flattened using carbide abrasive paper (P600) and teeth with any resulting dentin exposure were excluded. The teeth ( n = 70) were then equally divided into the 5 experimental groups according to their size.
24 h prior to testing the crowns were embedded in brass cylinder holders with dental die stone. Their exposed buccal surface gently re-sanded (P600), washed and re-examined prior to bonding. Circular lingual buttons (Item 224-112, TP Orthodontic Inc, UK) were used as the attachment device.
The bonding procedure used, followed the manufacturer’s suggested protocol. The exposed enamel surface was etched for 15 s with 40% phosphoric acid gel (Henry-Schein) and then rinsed for 30 s under pressurized tap water and dried (air dry). A thin layer of Transbond-XT Primer (3 M, Unitek) was applied onto the tooth’s surface for all the adhesives and a small amount of the OBA was placed on the base of the button. A metallic button was then tacked onto the surface of the tooth, gently compressed and any excess flash was removed. The adhesive was then light cured for 40 s using a high intensity soft-start LED light curing unit (Celalux, VOCO) of at least 1500 mW/cm 2 (measured with a high intensity radiometer; Demetron Research Corp.), at 90° angle directly above the button. The bonded specimens were then stored in a dry environment (23 °C), for 24 h.
The shear bond strength test was conducted using a Zwick/Roell Z020 Universal testing machine (Leominster, UK) and the Zwick/Roell TestXpert computer software program.
The testing machine was used with a 500 N loading cell and a crosshead speed used was 0.5 mm/min . The bracket’s ligature groove was used as the loading point. The specimens were loaded until the bracket-adhesive-enamel complex failed.
Shapiro–Wilk’s test and Levene’s test were used to assess for normality and homogeneity, and subsequently the Kruskal–Wallis and Mann–Whitney rank test statistics were applied, with a confidence level of 95% ( p < 0.05).
The bond strength (MPa) was calculated based on the following equation (Eq. (3) ):
where σ = stress, A = nominal surface area of attachment.
3
Results
3.1
Viscosity
The type of flow observed for both the filled and unfilled experimental UDMA resins was Newtonian. There was no significant change in the viscosity upon the application of differing shear rates. This was also observed for Transbond Primer, the unfilled Bis-GMA control.
Conversely, Transbond XT’s viscosity initially rose as the shear rate increased. It then peaked (6.67 × 10 3 Pa s, at a shear rate of 0.0693 1 s −1 ) and then progressively declined as the shear rate continued to increase.
The viscosities obtained for the unfilled UDMA resins ranged between 5.91 and 61.48 Pa s × 10 −2 , with a standard deviation ranging between 0.08 and 1.38 (Pa s × 10 −2 ). Transbond Primer had a viscosity of 0.179 Pa s (SD 0.0025). The values obtained for the filled resins were much greater, ranging between 1.38 and 16.33 Pa s, with a standard deviation of 0.10–0.17 Pa s ( Table 1 ).
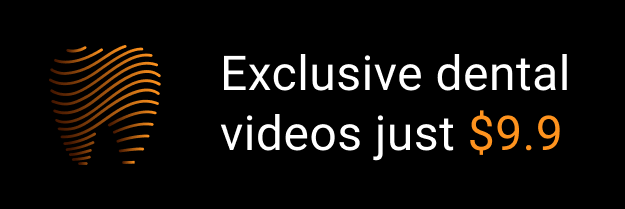