Enzymes
Publisher Summary
Living organisms continuously transform energy and materials and are able to do so only because of the innumerable enzymes that they contain. Enzymes act as catalysts whose function is to make or break covalent bonds that otherwise could only be created or destroyed under conditions of temperature and pH that are incompatible with life. Catalysts act by reducing the stability of bonds and lowering the amount of energy required to break them. This energy is known as the activation energy; the greater it is, the less reactive the compound or system. A catalyst lowers the activation energy by combining with the substrate and forming an unstable intermediate whose rapid decomposition provides an alternative pathway with a lower energy barrier. Whether the activation energy is high or low, the overall change in free energy in the reaction is the same. Enzymes are proteins, and while they resemble inorganic catalysts in reducing the activation energy and in remaining unchanged at the end of the reaction, they are much more specific, more efficient, and more readily inactivated. They achieve their effects by providing a surface on which their substrates are specifically adsorbed and orientated. As a result of the formation of this enzyme-substrate complex, a substrate that is to be broken down becomes strained just at the point where fission is to take place. In condensation reactions, instead of being dependent on random collisions, the reactants are attracted and held by the enzyme in exactly the position needed for the reaction to occur.
Catalysts act by reducing the stability of bonds and consequently lowering the amount of energy required to break them. This energy is known as the activation energy and the greater it is the less reactive the compound or system. A catalyst lowers the activation energy by combining with the substrate and forming an unstable intermediate whose rapid decomposition provides an alternative pathway with a lower energy barrier. Whether the activation energy is high or low the overall change in free energy in the reaction will be the same (Figure 6.1).
Classification of enzymes
Enzymes are classified into six main groups according to the type of reaction that they catalyse.
1. Oxidoreductases catalyse oxidoreduction reactions, and include dehydrogenases, oxidases, oxygenases, peroxidase and catalase.
2. Transferases catalyse the transfer of groups such as amino groups and phosphate.
3. Hydrolases hydrolyse glycosides and esters as well as peptide and other amide bonds, e.g. amylase, maltase, dextranase, lipase and trypsin.
4. Lyases either remove groups from their substrates leaving double bonds or alternatively add groups to existing double bonds, e.g. aldolase, fumarase.
5. Isomerases catalyse various intramolecular rearrangements such as the conversion of an aldose to a ketose sugar, or alteration of the position of a phosphate group, e.g. phosphohexoisomerase, phosphoglucomutase.
6. Ligases or synthetases catalyse the joining together of two molecules in a reaction that is coupled with the hydrolysis of a nucleoside triphosphate such as ATP (page 210), e.g. acetyl-CoA carboxylase, glycogen synthetase, succinic thiokinase.
Coenzymes
A considerable number of enzymes require the presence of one or more small organic molecules which participate in the overall reaction. These molecules are usually termed coenzymes, but since they react in stoichiometric proportions with the substrate(s), they are more accurately regarded as cosubstrates. The function of such molecules is to link reactions. They do this by acting as carriers of particular groups such as phosphate or acyl groups, or of reducing equivalents, e.g. electrons or hydrogen atoms (Table 6.1).
Table 6.1
Full name | Abbreviation | Group transferred | Corresponding vitamin |
I. Hydrogen-transferring coenzymes | |||
a. Nicotinamide–adenine dinucleotide | NAD | H+ + 2e | Nicotinamide |
b. Nicotinamide–adenine dinucleotide phosphate | NADP | H+ + 2e | Nicotinamide |
c. Flavin mononucleotide | FMN | 2H | Riboflavin |
d. Flavin adenine dinucleotide | FAD | 2H | Riboflavin |
e. Ubiquinone | CoQ | 2H | ? |
f. Lipoic acid | 2H + acyl | — | |
II. Group-transferring coenzymes | |||
a. Pyridoxal phosphate | PALP | Amino | Pyridoxine (B6) |
b. Tetrahydrofolate | CoF | Hydroxymethyl (formyl) | Folic acid |
c. Biotin | — | Carboxyl (CO2) | Biotin |
d. Cobalamin | B12 | Carboxyl | Cobalamin (B12) |
e. Coenzyme A | CoA | Acetyl and other acyl groups | Pantothenic acid |
f. Thiamine pyrophosphate | TPP | Acetaldehyde | Thiamine |
< ?xml:namespace prefix = "mml" />
The pyridoxal phosphate returns to its original form at the end of the reaction. In the case of other coenzymes, a second enzyme reaction must occur before the coenzyme is reconverted to its original form. For example, with NAD (page 214), which is a coenzyme for many dehydrogenase enzymes, the oxidized form of the coenzyme is reduced in the first enzyme reaction to NADH which must then be reoxidized by a second enzyme-catalysed reaction before it is able to participate again in the first type of reaction.

Pyridoxine, the parent substance of pyridoxal phosphate, is also known as vitamin B6 (page 165). Many coenzymes contain a derivative of one or other of the B vitamins as an essential part of their structure. Coenzymes may react with a number of different enzymes which are specific for different substrates but which catalyse the same general type of reaction.
Enzyme kinetics
Progress curves
If the progress of an enzyme reaction is plotted against time it is found that the velocity is initially high but soon begins to decrease so that a curve such as A in Figure 6.2 is obtained. If lower concentrations of enzyme are used similar curves (B) and (C) are obtained but the time taken to reach the same final concentration of product is increased.

and the forward and backward reactions are occurring at an equal rate. The slowing may also result from other changes in conditions occurring as a result of the reaction, such as an alteration in pH. Since enzyme studies are almost always comparative, it is possible to design the experiments so as to ensure that these factors apply equally in all cases. This is usually achieved by measuring the initial velocity (Vi) of the reaction, i.e. the slope of the curve in the earliest stages when the substrate concentration [S] is virtually unchanged, insignificant amounts of product have been formed, the original pH is maintained and the enzyme is not denatured, i.e. conditions approximate to those operating in an open system in a steady state.
The effect of temperature
Most enzyme reactions only occur at temperatures between 0 and 60°C. At the lower end of the range, enzyme reactions behave like ordinary chemical reactions and the rate increases as the temperature rises, the velocity being approximately doubled for every 10°C rise as a result of the increased kinetic energy of the reacting molecules. A typical curve relating the activity of an enzyme measured over a constant time, e.g. 5 or 10 minutes, with the temperature is shown in Figure 6.3 from which it may be seen that there is a definite optimum temperature which is usually related to the temperature of the environment of the cell from which the enzyme was derived. In mammals the optimum is about 37°C while, curiously, for many plant and bacterial enzymes it is higher than this. The rapid decrease in activity seen at temperatures above the optimum is due to heat denaturation, which occurs progressively as the temperature rises.
The effect of pH on enzyme activity
In view of the importance of pH in determining the ionization of proteins, it is not surprising that enzymes are extremely sensitive to it. Changes in their charge distribution may affect activity by altering either the overall conformation of the protein or the reactivity of amino acid side chain groupings involved in the active site. Each enzyme has a characteristic optimum pH although, if an enzyme acts on two different substrates, the optimum pH values may differ slightly. Pepsin is unusual in acting at very low pH values and has an optimum pH varying from 1.5 to 2.5 according to the protein being digested. Most enzymes have an optimum pH between 5 and 9 (Figure 6.4
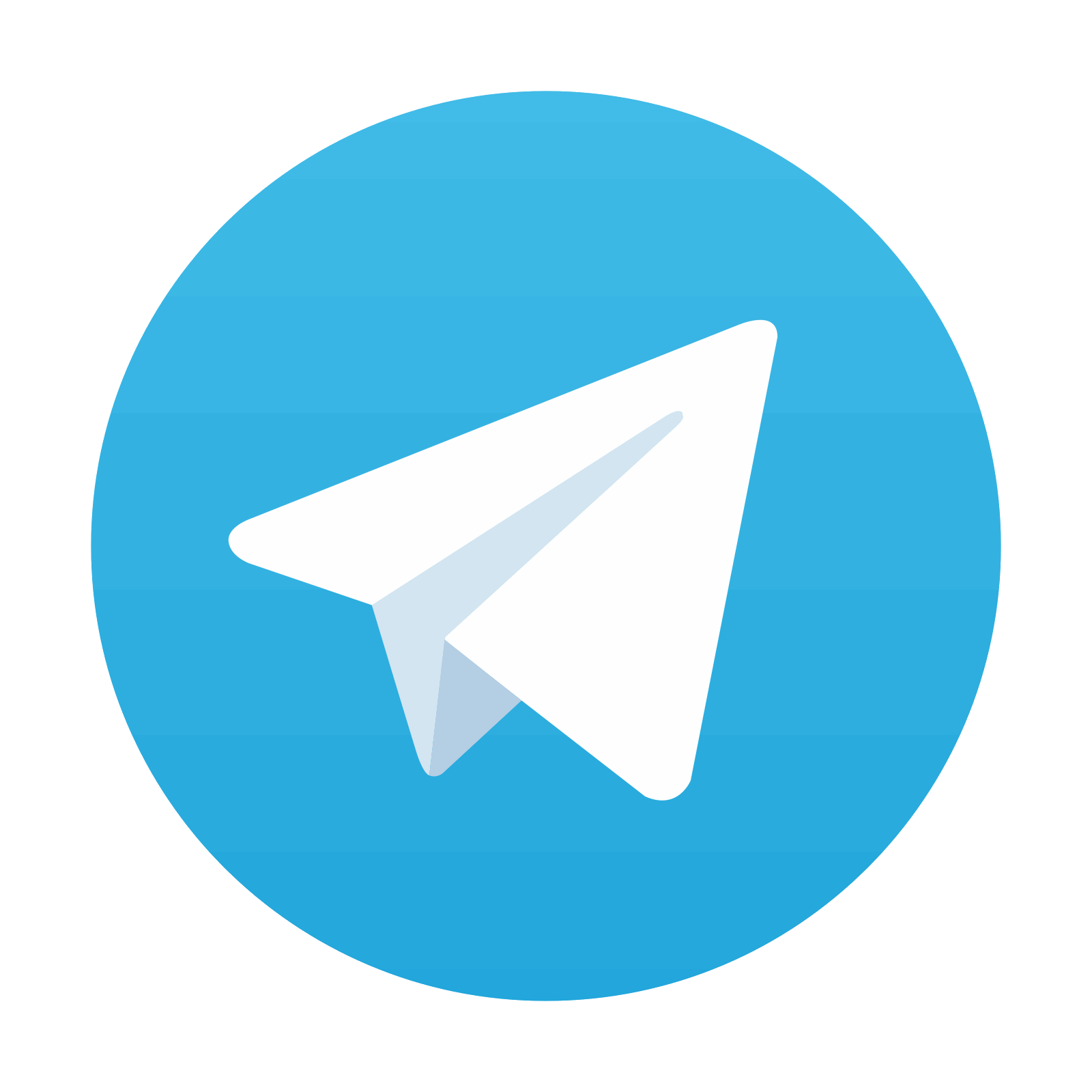
Stay updated, free dental videos. Join our Telegram channel

VIDEdental - Online dental courses
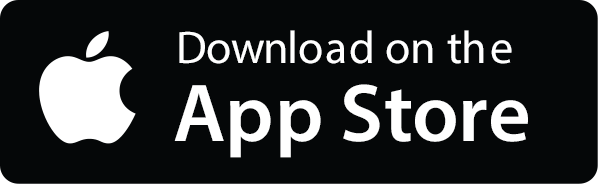
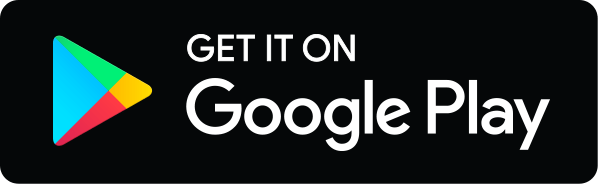