Introduction
We investigated whether ACTN3 , ENPP1 , ESR1 , PITX1 , and PITX2 genes which contribute to sagittal and vertical malocclusions also contribute to facial asymmetries and temporomandibular disorders (TMD) before and after orthodontic and orthognathic surgery treatment.
Methods
One hundred seventy-four patients with a dentofacial deformity were diagnosed as symmetric or subdivided into 4 asymmetric groups according to posteroanterior cephalometric measurements. TMD examination diagnosis and jaw pain and function (JPF) questionnaires assessed the presence and severity of TMD.
Results
Fifty-two percent of the patients were symmetric, and 48% were asymmetric. The asymmetry classification demonstrated significant cephalometric differences between the symmetric and asymmetric groups, and across the 4 asymmetric subtypes: group 1, mandibular body asymmetry; group 2, ramus asymmetry; group 3, atypical asymmetry; and group 4, C-shaped asymmetry. ENPP1 SNP-rs6569759 was associated with group 1 ( P = 0.004), and rs858339 was associated with group 3 ( P = 0.002). ESR1 SNP-rs164321 was associated with group 4 ( P = 0.019). These results were confirmed by principal component analysis that showed 3 principal components explaining almost 80% of the variations in the studied groups. Principal components 1 and 2 were associated with ESR1 SNP-rs3020318 ( P <0.05). Diagnoses of disc displacement with reduction, masticatory muscle myalgia, and arthralgia were highly prevalent in the asymmetry groups, and all had strong statistical associations with ENPP1 rs858339. The average JPF scores for asymmetric subjects before surgery (JPF, 7) were significantly higher than for symmetric subjects (JPF, 2). Patients in group 3 had the highest preoperative JPF scores, and groups 2 and 3 were most likely to be cured of TMD 1 year after treatment.
Conclusions
Posteroanterior cephalometrics can classify asymmetry into distinct groups and identify the probability of TMD and genotype associations. Orthodontic and orthognathic treatments of facial asymmetry are effective at eliminating TMD in most patients.
Highlights
- •
We used a new asymmetry classification system with posteroanterior cephalograms, producing 4 diagnostic groups.
- •
Pretreatment TMD was elevated in some asymmetric diagnostic groups.
- •
ENPP1 and ESR1 genotypes and alleles were associated with 3 of the 4 asymmetry groups.
- •
Orthodontic and orthognathic treatment of asymmetry elevates TMD symptoms for at least 1 year into retention in most patients.
- •
We gained new insights into what types of asymmetry predispose a patient to TMD.
Genesis creating symmetry and breaking of symmetry are normal developmental biologic processes. In embryogenesis, symmetry is maintained until late gastrulation and early neurulation. The nodal pathway regulates signal transduction to maintain symmetric dorsal-ventral patterning of the mesoderm and the developing nervous system. The lefty proteins, a subclass of transforming growth factor-β transcription proteins, act as extracellular antagonists to the nodal pathway. During gastrulation, lefty disrupts the symmetry in germ layers to pattern normal situs positioning of the heart, lungs, and gut coiling. In the harmonious development of brachial arches immediately rostral, maintenance of symmetry is particularly important for natural midline fusion between the right and left sides. Many genetic and environmental factors, acting separately or in unison, may produce facial clefting or developmental jaw defects in arch development. Due to the potential for congenital defects, different and more subtle influences on asymmetry might be active in normal facial development. PITX2 , an upstream effector of nodal and lefty signaling pathways, has recently been identified as differentially expressed between the left and right masticatory muscles in adults with facial asymmetry. PITX2 is necessary for mesoderm-derived first brachial arch structures, including masticatory muscles, jaw bones, and ectoderm induction for tooth formation. Therefore, it is likely that early developmental events persist into maturation, making asymmetry an etiologic and diagnostic challenge for clinical orthodontics.
Craniofacial asymmetry may be considered a type of dentofacial deformity that arises during facial maturation at the same time that sagittal and vertical jaw discrepancies become clinically evident. The association of skeletal asymmetry with Class II or Class III skeletal malocclusion varies with the population being treated, ranging from 8% to 50% and indicating that genetic influences from gastrulation to maturation play an important role in etiology. Skeletal malocclusions are complex trait conditions, developing from an interplay of genetic and functional (environmental) influences ; new findings point toward a similar etiology for facial asymmetry. To identify genetic contributions to skeletal malocclusions, a relatively precise description of phenotype and phenotypic variations is necessary. In orthodontics, there have been many approaches for classification of skeletal asymmetry using either posteroanterior cephalograms or submentovortex radiographs; however, there is no universally accepted method or classification system. Most recently, cone-beam computed tomographic (CBCT) imaging has been used to more accurately describe and subclassify the types of craniofacial asymmetry in populations with dentofacial deformity. The classification system of Baek et al has identified 4 types of asymmetry with computed tomography that arise from different growth imbalances of the jaws, teeth, nasal septum, and cranial base. These new classification systems help remove much of the previous diagnostic uncertainty, since asymmetry can be masked by variations in head posture, canting of the occlusal plane, or other dental and soft tissue compensations. This etiologic-based phenotypic classification may be useful in identifying gene single nucleotide polymorphisms (SNPs) associated with asymmetry.
We have been investigating how genetic variations are associated with the development of skeletal malocclusions in patients undergoing orthognathic surgery at the University of Lille in France. This is a typical population of French patients, relatively young with a normal distribution of open-bite, deepbite, Class II, and Class III skeletal malocclusions. In our subjects and in other studies of patients with dentofacial deformity, pain in the temporomandibular joint or masticatory muscles is a common and often debilitating comorbid condition estimated to occur in over 50% of these patients. The classification of Baek et al might also be useful in identifying an association between the subclasses of asymmetry and the prevalence of temporomandibular disorder (TMD).
In previous studies, we identified significant associations between SNPs in ACTN3 (α-actinin 3) and ESR1 (estrogen receptor α) with Class II malocclusion and ENPP1 (ectonucleotide pyrophosphatase/phosphodiesterase 1) with mandibular prognathism. We also found differences in gene expression for PITX1 and PITX2 between the left and right masseter muscles in subjects with assymetrey. In this study, we explored associations between these genes and subclassifications of asymmetry. We also sought to determine whether the subclassifications are related to the prevalence or severity of TMD before and after orthodontic treatment with jaw osteotomy.
Material and methods
For this study, we recruited 174 patients undergoing comprehensive orthodontic treatment with mandibular or mandibular and maxillary osteotomies for correction of jaw deformities during their presurgical consultations at the Department of Oral and Maxillofacial Surgery at the University of Lille. At that time, the patients signed consent for participation according to human subject research protocols approved by the Committee for Personal Protection, University of Lille (CPP 12/44), and the institutional review board committees at the University of Pittsburgh (PRO 12080373) and Temple University (13438). The patients provided a saliva sample collected in Oragene kits (DNA Genotek, Ontario, Canada) for genotyping. Deidentified demographic information, panoramic radiogram, lateral and posterior anterior cephalograms, and clinical examination information were compiled for analysis. The subjects had a mean age of 25.7 years; the majority were female (76%), and there was a normal mixture of sagittal (66% Class II, 33% Class III) and vertical jaw deformations (75% with open bite, 25% with deepbite). Sagittal and vertical malocclusion classifications were based on the Delaire Cephalometric Analysis of lateral cephalograms, which is particularly useful in planning the type of osteotomy repositioning necessary to correct the malocclusion.
Skeletal asymmetry was classified into 4 groups based on a new system adapted from Baek et al : group 1, lateralization of the mandibular body only (mandibular body asymmetry); group 2, difference in ramus heights with menton deviation to the shorter ramus side (ramus asymmetry); group 3, difference in ramus heights with menton deviation to the longer ramus side, gonion contour more prominent on the larger mandibular side, and reverse maxillary canting (atypical asymmetry); and group 4, difference in ramus heights with menton deviation to the short ramus side and severe maxillary canting (C-shaped asymmetry) ( Fig 1 ). Since this classification system was derived from CBCT images of orthognathic patients, we developed a posteroanterior cephalometric analysis, which allowed us to perform comparable measurements using digital 2-dimensional images with morphometric software (Dolphin Imaging, Chatsworth, Calif).

Six cephalometric measurements were used: occlusal plane tilt, maxillary canting, menton deviation, mandibular width to midsagittal plane, mandibular width to menton, and ramal height ( Tables I and II ). In the maxilla, occlusal plane tilt was determined by the difference between the Frankfort horizontal and the horizontal line bisecting the buccal cusp tips of the maxillary and mandibular right first molars, as well as the buccal cusp tips of the maxillary and mandibular left first molars, measured in degrees. If the occlusal plane tilt was greater than 2°, the subject was considered to have maxillary canting. To further verify that the maxillary canting was skeletal in its etiology, the left and right vertical distances from the jugal process and the frontozygomatic suture were compared. If the difference between the left and right sides was greater than 3 mm, the subject was considered to have maxillary canting. In the mandible, menton deviation was determined by the angle between the midsagittal plane and the line connecting anterior nasal spine and menton. If the angle was greater than 2°, the subject was considered to have mandibular facial asymmetry with menton deviation. To compare the left and right mandibular widths, the distance between the antegonial notch and the midsagittal plane was compared with the contralateral side. If the difference was greater than 2 mm, the subject was considered to have mandibular deviation. We also measured the distance between the antegonial notch and menton, and compared it with its contralateral side. Again, if the difference was greater than 2 mm, the subject was considered to have mandibular body asymmetry. Ramal heights of the left and right sides were compared. If the difference between the 2 sides was greater than 3 mm, ramus asymmetry was diagnosed.
Landmark | Definition |
---|---|
ANS | Anterior nasal spine |
AG/GA | The highest points in the antegonial notches (left and right) |
Co | Condylion; most superior point on the condylar head |
Crista galli | Most superior point at its intersection with the sphenoid |
Gonion/most lateral ramus | Most inferior, posterior, and lateral point at the gonial angle of the mandible |
JL/JR | Bilateral points on the jugal process at the intersection of the outline of the tuberosity of the maxilla and zygomatic buttress |
Me | Menton; most inferior point at symphysis |
Midsagittal plane | A plane bisecting the head and face through crista galli, ANS, and genial tubercles in a symmetric face |
Occlusal plane | Horizontal line bisecting UR6 and LR6 and UL6 and LL6 |
UR6/UL6 | Buccal cusp tips of the maxillary right and left first molars |
LR6/LL6 | Buccal cusp tips of the mandibular right and left first molars |
ZL/ZR | Medial aspect of the frontozygomatic suture (bilateral) |
Measurement | Definition |
---|---|
Maxilla | |
Occlusal plane tilt (°) | Difference between the Frankfort horizontal and the horizontal lines bisecting UR6 and LR6 and UL6 and LL6 |
Maxillary canting | |
JL or JR-ZL or ZR (mm) | Difference between the vertical distance from the left or right jugal process between the left or right frontozygomatic suture |
Mandible | |
Menton deviation | |
A-Me-MSR (°) | Angle formed between the midsagittal plane and the line though ANS and menton |
Mandibular width to midsagittal plane | |
GA or AG-MSR (mm) | Distance between the left or right antegonial notch and the midsagittal plane |
Mandibular width to menton | |
GA or AG-menton (mm) | Distance between the left or right antegonial notch and menton |
Ramal height (right or left) (mm) | Linear distance between condylion to the most lateral ramus (gonion) |
Subjects were diagnosed as symmetric if there was no maxillary canting (<2°), no menton deviation (<2°), and no significant difference in ramal height (<3 mm). Asymmetric subjects were further divided into 4 groups. Group 1 subjects had a menton deviation greater than 2° without maxillary canting or a significant ramal height difference. Group 2 subjects had a menton deviation greater than 2° with a shorter ramal height on the deviated side. Group 3 subjects displayed atypical symmetry, with a shorter ramal height opposite the deviated side and slight maxillary canting toward or opposite the deviated side. Group 4 subjects showed both shorter ramal height and maxillary canting toward the deviated side, displaying C-shaped asymmetry as described by Baek et al. Furthermore, principal component analysis explaining more than 5% of the facial skeletal variation was selected for genotype-phenotype correlation analysis. Data were normalized and standardized using a linear model to assess the possible effects of age and sex and to consider the possibility of age-by-sex interactions.
Saliva samples, 1 per patient, were collected in the Oragene kits and prepared for DNA extraction and posterior genotyping using TaqMan chemistry and end-point analysis in an automatic sequence-detection instrument (ABI Prism 7900HT; Applied Biosystems, Foster City, Calif), as described previously. Thirteen SNPs were selected for genotyping: in ACTN3 rs1815739 and rs678397 ; in ENPP1, rs937300, rs6569759, rs858339, and rs1409181 ; in ESR1, rs1643821, rs302318, rs3020377, and rs2077647 ; in PITX,1 rs1131611; and in PITX2, rs2595110 to determine whether specific allelic variants were overrepresented in subjects with malocclusion subclassifications. Thirty-three additional anonym SNPs were genotyped.
TMD was assessed using the routine clinical examination done by maxillofacial surgeons before surgical treatment and entered into the Research Diagnostic Criteria for Temporomandibular Disorders. In addition, the jaw pain and function (JPF) questionnaire was used to determine the presence and severity of TMD, as a subjective patient report. This questionnaire was developed as a screening tool to determine the presence or absence of TMD conditions. It consists of 8 questions relating to jaw pain and 5 questions related to jaw function. The questionnaire has been validated to reliably distinguish between normal and TMD subjects with up to 98% sensitivity and 100% specificity when a cutoff score of 6 is used for responses. The questionnaire has been translated for use in German, and we prepared a French version for our use as a standard assessment of the presence and severity of TMD.
One hundred twenty-one patients attended a 1-year posttreatment reevaluation appointment, where they completed a second JPF survey. Patients were divided into 5 groups for comparative purposes based on the difference in presurgical and 1-year posttreatment JPF scores. They were classified in the following 5 groups: no change in TMD, improvement of TMD if the JPF score decreased by 3 or more; worsening of TMD if the JPF score increased by 3 or more; cured of TMD if the JPF score was 6 or greater before surgery and less than 6 after treatment; and iatrogenic TMD if the JPF score was less than 6 before surgery and greater than 6 after treatment. We used the terms “cured” or “iatrogenic” since a score of 6 or greater is diagnostic for the presence or absence of TMD with the JPF assessment.
Statistical analysis
For the cephalometric assessment of asymmetry, an unpaired t test was used to compare whether differences between sides for individual cephalometric measurements were significantly different between the symmetric and asymmetric patients. Analysis of variance (ANOVA) was used between the 4 subclassifications of asymmetry to determine anatomic differences between them. Tests for measurement error included intrarater reliability in cephalometric measurements; repeating the cephalometric tracings on 10% of the radiographs by 1 examiner (K.C.) resulted in an R 2 value of 0.98.
For genotype assessment, the subjects’ characteristics were analyzed with the usual rules of descriptive statistics: frequencies and percentages for categorical variables, and means and standard deviations for quantitative variables. The associations between the changes in JPF scores, TMD diagnoses, and the subjects’ clinical or surgical characteristics were assessed with the chi-square test for categorical variables or the Fisher exact test in the case of small numbers. Quantitative variables were analyzed using the Student t test. When the distribution of the variable was not normal, the nonparametric Wilcoxon test was performed. After ensuring compliance with the Hardy-Weinberg equilibrium, the association of different SNPs was also sought with the chi-square or Fisher exact test when there were small numbers. For each SNP, the analysis was performed by considering the 3 genotypes separately, as well as by calculating the total number of alleles.
For principal component analysis, SNPs were coded 0, 1, and 2 according to the number of minor allele copies. Multivariate linear regressions adjusting for age, sex, and ethnicity were performed to test for associations between each SNP (1 at a time) and the selected principal components. The same Bonferroni threshold described above was used here. All analyses were performed with SPSS software for Windows (version 20.0; IBM, Armonk, NY).
For the TMD assessment, an unpaired t test was used to determine whether there were significant differences in presurgical JPF scores between symmetric and asymmetric patients, and ANOVA was performed between the 4 asymmetric groups. ANOVA was also used to compare any significant changes in JPF scores 1 year after treatment between the symmetric group and the 4 asymmetric groups. To further evaluate, a post hoc t test was done to compare individual JPF scores in the asymmetric groups.
Results
The patient population represented a normal demographic distribution of subjects seeking orthodontic and orthognathic surgery treatment for dentofacial deformity malocclusion from the geographic areas of Northern France and Southern Belgium. All patients treated for this condition were referred to the Oral and Maxillofacial Surgery Department at the University of Lille under the National Health Care Service of France. Fifty-two percent of the patients were diagnosed as symmetric, and 48% were diagnosed as asymmetric. Segregating the asymmetric subjects into the 4 proposed subtypes was relatively easy to accomplish with the 11 cephalometric anatomic landmarks and 6 cephalometric measurements ( Tables I and II ; Fig 1 ). This classification system was validated by the significant differences obtained by comparing the cephalometric measurements between the symmetric and asymmetric groups, and the asymmetric subtypes ( Table III ). All 6 posteroanterior cephalometric measurements were compared between the symmetric and asymmetric patients, and across the 4 asymmetry subclassification groups. For the bilateral points, the values are the difference between the left and right sides. The posteroanterior cephalometric analysis results demonstrated significant differences in all 6 cephalometric measurements between the symmetric and asymmetric patients. All mandibular measurements—menton deviation, mandibular width, and ramal height—showed notably significant differences between the symmetric and asymmetric patients, clearly indicating mandibular asymmetry.
Measurement | Symmetric | Asymmetric | P value ∗ |
---|---|---|---|
Occlusal plane tilt (°) | 0.87 ± 0.59 | 1.36 ± 1.47 | 0.0040 |
Maxillary canting | |||
JL or JR-ZL or ZR (mm) | 0.68 ± 0.44 | 2.70 ± 5.17 | 0.0003 |
Menton deviation | |||
A-Me-MSR (°) | 0.31 ± 0.29 | 4.47 ± 2.10 | <0.0001 |
Mandibular width to midsagittal plane | |||
GA or AG-MSR (mm) | 1.51 ± 1.31 | 14.2 ± 12.8 | <0.0001 |
Mandibular width to menton | |||
GA or AG-menton (mm) | 1.51 ± 2.69 | 21.4 ± 14.9 | <0.0001 |
Ramal height (mm) | 1.41 ± 0.79 | 14.5 ± 12.1 | <0.0001 |
Cephalometric measurements were also compared across the different asymmetry groups ( Fig 2 ). Generally, group 4 showed greater amounts of canting and deviation when we compared cephalometric measurements. The subjects in this group had the most severe malformations, with occlusal plane tilt, maxillary canting, and mandibular deviation that were proportionally much more imbalanced than in the other asymmetry groups. Because group 4 had maxillary canting, the anterior nasal spine to menton line used to measure chin deviation in the mandible was higher than in the other 3 groups ( P = 0.003; Fig 2 ). The second characteristic evident with asymmetry was the worsening of the ramal height differences from group 1 to group 4. Differences in the posteroanterior cephalometric measurements indicated that the 4 subtypes may be considered as anatomically different forms of asymmetry that can be compared for differences in genotype variations. We therefore compared differences in genotype for the symmetric and asymmetric subjects and between the asymmetric subtypes ( Table IV ).
Gene | SNP | Genotype | Group 1 | Group 2 | Group 3 | Group 4 | Symmetric | Symmetry vs asymmetry | |
---|---|---|---|---|---|---|---|---|---|
P value-genotype | P value-allele | ||||||||
ENPP1 | rs9373000 | AA | 4 (57) | 17 (65) | 9 (41) | 6 (67) | 35 (54) | ||
AG | 2 (29) | 7 (27) | 10 (46) | 3 (33) | 28 (43) | ||||
GG | 1 (14) | 2 (8) | 3 (13) | 0 (0) | 2 (3) | 0.24 | 0.83 | ||
P value genotype | 0.33 | 0.21 | 0.15 | 0.62 | |||||
P value allele | 0.23 | 0.46 | 0.13 | 0.19 | |||||
rs6569759 | GG | 5 (72) | 6 (21) | 5 (22.5) | 2 (22) | 11 (17) | |||
AG | 1 (14) | 12 (46) | 12 (55) | 5 (56) | 38 (58) | ||||
AA | 1 (14) | 8 (33) | 5 (22.5) | 2 (22) | 16 (25) | 0.27 | 0.38 | ||
P value genotype | 0.004 ∗ | 0.56 | 0.83 | 0.92 | |||||
P value allele | 0.016 ∗ | 1 | 0.66 | 0.76 | |||||
rs858339 | TT | 1 (16) | 3 (9) | 0 (0) | 0 (0) | 20 (30) | |||
AT | 3 (42) | 10 (36) | 13 (59) | 4 (44) | 12 (18) | ||||
AA | 3 (42) | 15 (55) | 9 (41) | 5 (56) | 33 (52) | 0.002 ∗ | 0.55 | ||
P value genotype | 0.33 | 0.13 | 0.002 ∗ | 0.33 | |||||
P value allele | 0.27 | 0.77 | 0.14 | 0.24 | |||||
rs1409181 | CC | 1 (14) | 5 (12) | 5 (22.5) | 1 (12) | 9 (15) | |||
CG | 4 (57) | 15 (55) | 13 (59) | 7 (76) | 36 (55) | ||||
GG | 2 (29) | 8 (33) | 4 (18.5) | 1 (12) | 20 (30) | 0.53 | 0.31 | ||
P value genotype | 0.99 | 0.88 | 0.41 | 0.41 | |||||
P value allele | 0.92 | 0.69 | 0.21 | 0.49 | |||||
ESR1 | rs1643821 | GG | 1 (16) | 3 (9) | 3 (13) | 3 (32) | 4 (7) | ||
AG | 3 (42) | 14 (50) | 10 (46) | 5 (56) | 36 (55) | ||||
AA | 3 (42) | 11 (41) | 9 (41) | 1 (12) | 25 (38) | 0.23 | 0.3 | ||
P value genotype | 0.63 | 0.72 | 0.48 | 0.019 ∗ | |||||
P value allele | 0.58 | 0.8 | 0.76 | 0.02 ∗ | |||||
rs3020318 | TT | 3 (43) | 12 (46) | 12 (55) | 1 (12) | 24 (37) | |||
CT | 4 (57) | 13 (45) | 7 (32) | 7 (76) | 28 (43) | ||||
CC | 0 (0) | 3 (9) | 3 (13) | 1 (12) | 13 (20) | 0.39 | 0.27 | ||
P value genotype | 0.47 | 0.46 | 0.4 | 0.16 | |||||
P value allele | 0.17 | 0.29 | 0.23 | 0.4 | |||||
rs3020377 | GG | 3 (43) | 13 (45) | 12 (55) | 3 (33) | 27 (41) | |||
AG | 4 (57) | 12 (46) | 6 (26.5) | 6 (67) | 31 (48) | ||||
AA | 0 (0) | 3 (9) | 4 (18.5) | 0 (0) | 7 (11) | 0.78 | 0.51 | ||
P value genotype | 0.65 | 0.8 | 0.23 | 0.28 | |||||
P value allele | 0.22 | 0.51 | 0.73 | 0.91 | |||||
rs2077647 | TT | 2 (29) | 2 (8) | 3 (13) | 1 (12) | 7 (11) | |||
CT | 2 (29) | 20 (71) | 11 (51) | 6 (67) | 34 (52) | ||||
CC | 3 (42) | 6 (21) | 8 (36) | 2 (21) | 24 (37) | 0.63 | 0.53 | ||
P value genotype | 0.37 | 0.2 | 0.98 | 0.67 | |||||
P value allele | 0.71 | 0.52 | 0.93 | 0.59 |
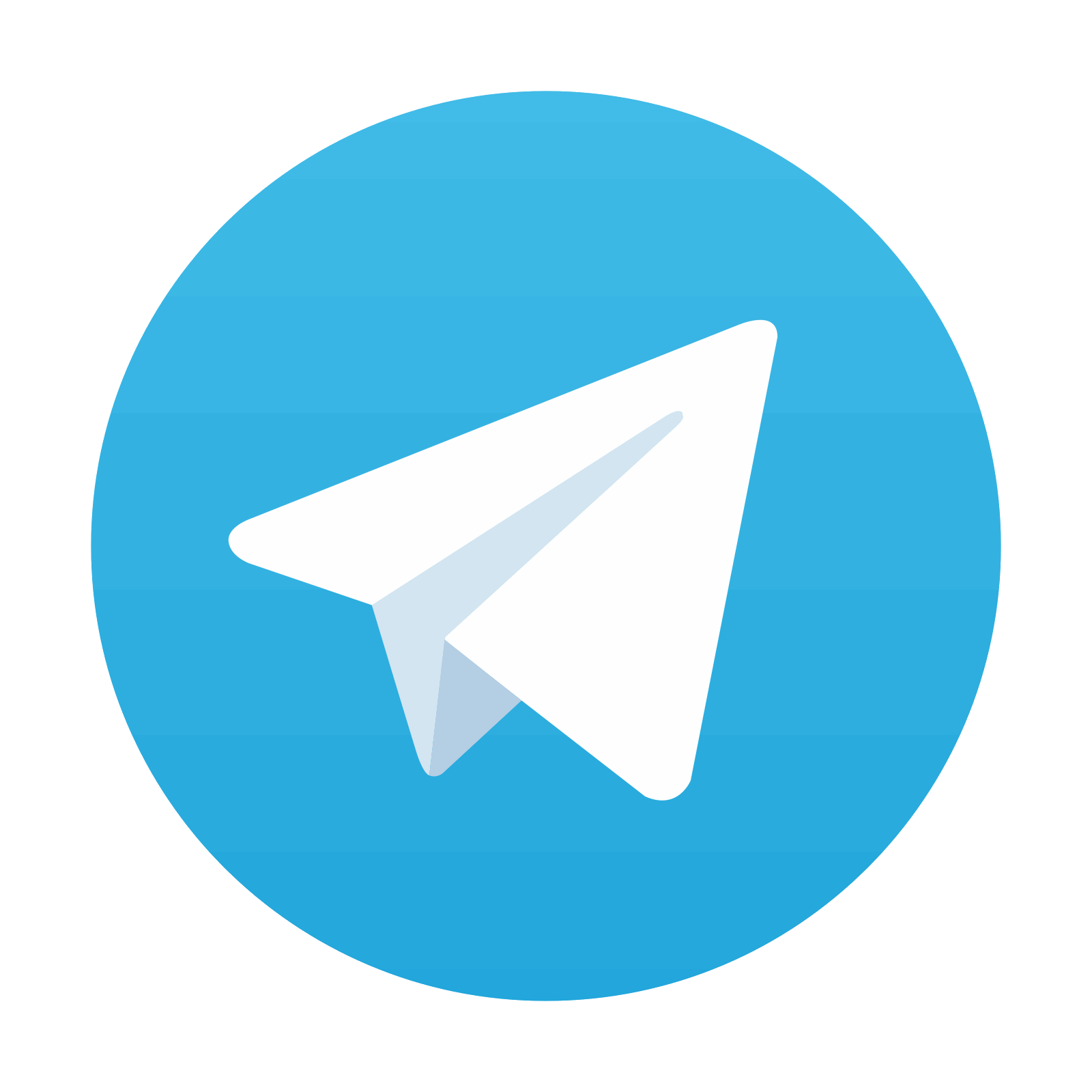
Stay updated, free dental videos. Join our Telegram channel

VIDEdental - Online dental courses
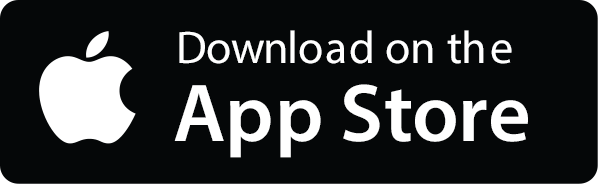
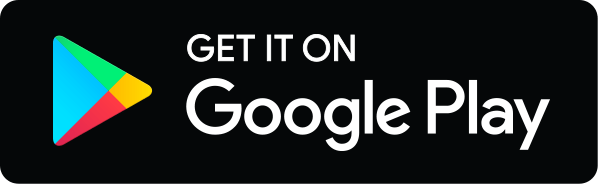
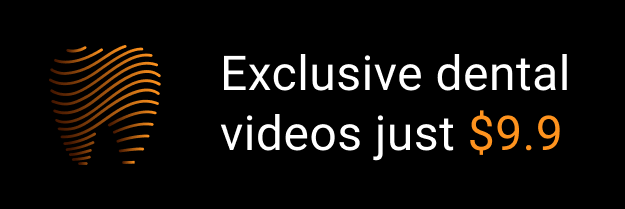