Abstract
Objective
To investigate whether proanthocyanidins (PA) is capable of improving dentin collagen’s biological stability through cross-linking within time periods that are clinically relevant.
Materials and methods
Demineralized dentin collagen slabs were treated with 3.75 wt% PA solution for 10 s, 1 min, 30 min, 60 min, 120 min, 360 min, and 720 min, respectively. The resultant cross-linked collagen samples were subject to digestion with 0.1% collagenase at 37 °C for 2 h, 6 h, 12 h, 24 h, 36 h, and 48 h. The percentage of weight loss after digestion was calculated to evaluate PA-treated collagen’s resistance toward enzymatic degradation. Fourier-transformed infrared (FTIR) spectroscopy was used to probe evidences of PA-collagen interactions after various periods of PA treatment.
Results
The collagenase digestion assay suggests that PA treatment as short as 10 s can enhance collagen’s resistance toward enzymatic challenge. The FTIR spectroscopy further verifies that PA is indeed incorporated into collagen regardless of treatment time, possibly via a mechanism involving the chemical interactions between PA and collagen.
Significance
This study confirmed that PA can effectively cross-link collagen and improve its biological stability in time periods as short as 10 s. The use of PA as a priming agent is therefore clinically feasible and is a promising approach to improving the durability of current dentin bonding systems.
1
Introduction
The use of adhesive systems is a common practice in current restorative dentistry to promote strong bonding between restorative materials and dentin substrates . While the short-term effectiveness of modern adhesive systems is well recognized , their long-term stability leaves a lot to be desired . One of the leading causes for restoration failure is the breakdown of the adhesive/dentin (a/d) interface . This interface, also known as the “a/d hybrid layer”, is composed of collagen fibrils and adhesive resin that form an interlocked entanglement, providing for the basis of bonding between the bulk adhesive and the underlying intact dentin . Due to the incomplete penetration of adhesive monomers into the interfacial layer , the collagen fibrils are not completely impregnated by resin as they were by hydroxyapatite mineral, rendering them susceptible to hydrolysis and enzymatic degradation. In addition, recent studies have shown that acid etching of dentin activates the otherwise dormant host-derived matrix metalloproteinases (MMPs) , increasing the risk of breakdown of the a/d hybrid layer.
As such, the improvement of collagen’s stability within the a/d hybrid layer has been an intriguing target for dentin bonding research. Previous studies have shown that collagen’s mechanical and biological stability can be enhanced after being treated with a variety of cross-linking agents . A group of naturally occurring polyphenolic compounds, proanthocyanidins (PA) is of particular interest to us, because PA is not only capable of cross-linking dentin collagen, its non-toxicity is also a much coveted property that cannot be found in other cross-linking agents such as glutaraldehyde and carbodiimides . It is generally believed that the protein-polyphenolics complexation is a result of non-covalent interactions including hydrogen bonding and hydrophobic effect , while a few studies have also indicated the possibility of covalent bond formation under certain circumstances . There are two possible options when incorporating PA in current adhesive systems: as an additive to the adhesives, or as a primer. Green et al. tested the first option , but it was found to result in a less than optimum quality of the hybrid layer, presumably due to the low degree of double bond conversion caused by PA’s radical-scavenging ability. In addition, Hechler et al. evaluated the long term performance of both options with Single Bond adhesive (3M ESPE Dental Products, St. Paul, MN) , and it turns out that after 52 weeks’ exposure to collagenase digestion, the microtensile bond strength of dentin beams with PA as a primer is significantly higher than control, whereas that of dentin beams with PA as an additive shows no significant difference to control. This observation strongly supports the use of PA as a primer. The limitation of this study, however, is that the PA priming time has been set to 1 h, which is clinically irrelevant. As such, the purpose of the present work is to evaluate PA’s ability to cross-link and stabilize dentin collagen in clinically relevant time periods.
Most previous studies have chosen collagen’s mechanical properties to gauge PA’s stabilizing effect . This approach, however, inevitably calls for long treatment times for two reasons. First, during the biosynthesis of collagen, extensive lysyl oxidase-mediated covalent cross-linking is already existent , which is the reason why dentin collagen has superior mechanical strength even when untreated. Therefore, it is inherently difficult to further increase collagen’s mechanical performance by introducing more cross-links. Second and more importantly, mechanical tests are highly sensitive to specimen size and shape in addition to the intrinsic inter- and intra-tooth differences in dentin’s tubular structures and orientation . Since all these factors are impossible to standardize, substantial noise is introduced to the test outcome . As a result, longer periods of PA treatment become necessary, because in order to observe any significant changes to collagen’s mechanical properties, PA’s stabilizing effect must be big enough to overcome the high noise level. One article by Liu et al. did examine the changes in dentin collagen’s ultimate tensile strength after being treated by PA for clinically favorable time periods (30 s, 60 s, and 120 s), but their specimen were all cut into hourglass shapes in order to reduce the noise caused by sample shape. Even by doing so, dentin collagen’s ultimate tensile strength showed no significant improvement regardless of treatment time, unless the concentration of PA was increased from 5% to 10% and 15%.
Apparently, researchers have gone to great lengths to examine collagen’s mechanical enhancement upon cross-linking. In contrast, we believe that although increased mechanical properties are certainly a manifestation of PA’s cross-linking capabilities, they are not our ultimate goal. Dentin collagen is already mechanically sound due to the innate extensive cross-links, thus the biological breakdown of collagen fibrils poses bigger threat than mechanical breakdown to the integrity of the a/d hybrid layer. In light of this, the present study aims to evaluate PA’s effect in clinically relevant durations (as short as 10 s and 1 min) on dentin collagen’s resistance toward enzymatic degradation. The null hypothesis tested is that PA could not enhance dentin collagen’s resistance toward enzymatic degradation in clinically relevant times. In addition, the FTIR spectroscopy is employed to investigate structural changes of collagen after PA treatment and to probe possible interactions between PA and collagen.
2
Materials and methods
2.1
Dentin collagen preparation
Extracted non-carious human molars stored in 0.96% (w/v) phosphate buffered saline (PBS) containing 0.002% sodium azide at 4 °C were used. All teeth were collected after obtaining the patient’s informed consent under a protocol approved by the University of Missouri-Kansas City Adult Health Sciences Institutional Review Board. A flat base was created by removing the roots of the teeth 2–3 mm below the cemento-enamel junction, using a water-cooled low-speed diamond saw (Buehler, Lake Bluff, IL). Then the teeth were attached to an aluminum block with cyanoacrylate adhesive (Zapit, Dental Ventures of America, Corona, CA), and the occlusal one-third to one-half was removed using the same saw.
These teeth were divided into two parts. Three teeth were used to make dentin films. Surface dentin of these teeth was cut into 10 μm thick films by means of a tungsten carbide knife mounted on a SM2500S microtome (Leica, Deerfield, IL). Six teeth were used to prepare dentin slabs. The same water-cooled low-speed saw was used to make perpendicular cuts into the dentin surface at 1.2 mm increments. A single cut was then made parallel to and about 1.2 mm beneath the surface dentin. This freed dentin slabs from the remaining dentin blocks. Because some tooth structure was removed due to the thickness (∼0.35 mm) of the saw blade, the final dimensions of the slabs were approximately 0.8 mm × 0.8 mm, and the length varied with respect to their position (10–12 mm). These dentin films and slabs were fully demineralized by 0.5 M EDTA solution (pH = 7.4) to make dentin collagen samples and washed with deionized water 3 times.
2.2
Proanthocyanidins (PA) and collagenase solution preparation
The PA solution was prepared by adding a powdered grape seed extract (MegaNatural Gold, containing over 90% PA, Polyphenolics, Madera, CA) to deionized water to a concentration of 3.75 wt%.
For the collagenase solution, TESCA buffer was first prepared by the addition of 11.5 g N-tris(hydroxymethyl)methyl-2-aminoethanesulfonic acid, 50 mg sodium azide and 53 mg CaCl 2 ·2H 2 O into distilled water to a total volume of 1000 mL, followed by pH adjustment to 7.4. Then 1 g collagenase with a molecular weight of ∼110 kDa (Collagenase Type I, Clostridiopeptidase A from Clostridium histolyticum, 125 U/mg, Sigma–Aldrich, St. Louis, MO) was dissolved in the TESCA buffer to a final concentration of 0.1% (w/v).
2.3
PA treatment, collagenase treatment and analysis of collagen slabs
Demineralized dentin collagen slabs were divided into 8 groups randomly (3 slabs per group). The slabs were treated by 3.75 wt% PA solution for 0 (control), 10 s, 1 min, 30 min, 60 min, 120 min, 360 min, and 720 min, respectively. Prior to and after PA treatment, each slab was rinsed with excessive deionized water 3 times, and then dried in a desiccator for 24 h. Dry slabs were weighed carefully using an electronic balance (AG285, Mettler Toledo, Columbus, OH), after which they were re-expanded in distilled water for 1 h, and digested by the collagenase solution for 2 h. Slabs were rinsed, dried and weighed again. The percentage weight loss was calculated with respect to the weight of the slab after PA treatment. This weigh-and-digest procedure was repeated for several times so that the digesting time was 2 h, 6 h, 12 h, 24 h, 36 h and 48 h. All treated slabs were individually incubated at 37 °C with shaking in wells of a plastic cell culture plate (Costar 24, Corning, Lowell, MA) holding ∼2.5 mL of collagenase solution per well. The collagenase solution was changed every 24 h for treatment times of 36 h and 48 h. Statistical analysis was done with two-tailed unpaired Student’s t -tests, and significance level was set to 5% ( p < 0.05).
2.4
PA treatment and FTIR spectra of collagen films
Demineralized dentin collagen films were divided into 8 groups randomly, each group containing 4 films. The films were treated by PA solution, rinsed, and dried in the same way as above. The spectra of dry films were taken by an infrared spectrometer (Spectrum One, Fourier transform infrared spectrophotometer, Perkin-Elmer, Waltham, MA) at a resolution of 4 cm −1 . The films were made to attach the diamond crystal top-plate of an Attenuated Total Reflectance (ATR) accessory (Perkin-Elmer, Waltham, MA) with a gauge force of 100. The ATR crystal is zinc selenide (ZnSe) with a transmission range between 650 and 4000 cm −1 .
2
Materials and methods
2.1
Dentin collagen preparation
Extracted non-carious human molars stored in 0.96% (w/v) phosphate buffered saline (PBS) containing 0.002% sodium azide at 4 °C were used. All teeth were collected after obtaining the patient’s informed consent under a protocol approved by the University of Missouri-Kansas City Adult Health Sciences Institutional Review Board. A flat base was created by removing the roots of the teeth 2–3 mm below the cemento-enamel junction, using a water-cooled low-speed diamond saw (Buehler, Lake Bluff, IL). Then the teeth were attached to an aluminum block with cyanoacrylate adhesive (Zapit, Dental Ventures of America, Corona, CA), and the occlusal one-third to one-half was removed using the same saw.
These teeth were divided into two parts. Three teeth were used to make dentin films. Surface dentin of these teeth was cut into 10 μm thick films by means of a tungsten carbide knife mounted on a SM2500S microtome (Leica, Deerfield, IL). Six teeth were used to prepare dentin slabs. The same water-cooled low-speed saw was used to make perpendicular cuts into the dentin surface at 1.2 mm increments. A single cut was then made parallel to and about 1.2 mm beneath the surface dentin. This freed dentin slabs from the remaining dentin blocks. Because some tooth structure was removed due to the thickness (∼0.35 mm) of the saw blade, the final dimensions of the slabs were approximately 0.8 mm × 0.8 mm, and the length varied with respect to their position (10–12 mm). These dentin films and slabs were fully demineralized by 0.5 M EDTA solution (pH = 7.4) to make dentin collagen samples and washed with deionized water 3 times.
2.2
Proanthocyanidins (PA) and collagenase solution preparation
The PA solution was prepared by adding a powdered grape seed extract (MegaNatural Gold, containing over 90% PA, Polyphenolics, Madera, CA) to deionized water to a concentration of 3.75 wt%.
For the collagenase solution, TESCA buffer was first prepared by the addition of 11.5 g N-tris(hydroxymethyl)methyl-2-aminoethanesulfonic acid, 50 mg sodium azide and 53 mg CaCl 2 ·2H 2 O into distilled water to a total volume of 1000 mL, followed by pH adjustment to 7.4. Then 1 g collagenase with a molecular weight of ∼110 kDa (Collagenase Type I, Clostridiopeptidase A from Clostridium histolyticum, 125 U/mg, Sigma–Aldrich, St. Louis, MO) was dissolved in the TESCA buffer to a final concentration of 0.1% (w/v).
2.3
PA treatment, collagenase treatment and analysis of collagen slabs
Demineralized dentin collagen slabs were divided into 8 groups randomly (3 slabs per group). The slabs were treated by 3.75 wt% PA solution for 0 (control), 10 s, 1 min, 30 min, 60 min, 120 min, 360 min, and 720 min, respectively. Prior to and after PA treatment, each slab was rinsed with excessive deionized water 3 times, and then dried in a desiccator for 24 h. Dry slabs were weighed carefully using an electronic balance (AG285, Mettler Toledo, Columbus, OH), after which they were re-expanded in distilled water for 1 h, and digested by the collagenase solution for 2 h. Slabs were rinsed, dried and weighed again. The percentage weight loss was calculated with respect to the weight of the slab after PA treatment. This weigh-and-digest procedure was repeated for several times so that the digesting time was 2 h, 6 h, 12 h, 24 h, 36 h and 48 h. All treated slabs were individually incubated at 37 °C with shaking in wells of a plastic cell culture plate (Costar 24, Corning, Lowell, MA) holding ∼2.5 mL of collagenase solution per well. The collagenase solution was changed every 24 h for treatment times of 36 h and 48 h. Statistical analysis was done with two-tailed unpaired Student’s t -tests, and significance level was set to 5% ( p < 0.05).
2.4
PA treatment and FTIR spectra of collagen films
Demineralized dentin collagen films were divided into 8 groups randomly, each group containing 4 films. The films were treated by PA solution, rinsed, and dried in the same way as above. The spectra of dry films were taken by an infrared spectrometer (Spectrum One, Fourier transform infrared spectrophotometer, Perkin-Elmer, Waltham, MA) at a resolution of 4 cm −1 . The films were made to attach the diamond crystal top-plate of an Attenuated Total Reflectance (ATR) accessory (Perkin-Elmer, Waltham, MA) with a gauge force of 100. The ATR crystal is zinc selenide (ZnSe) with a transmission range between 650 and 4000 cm −1 .
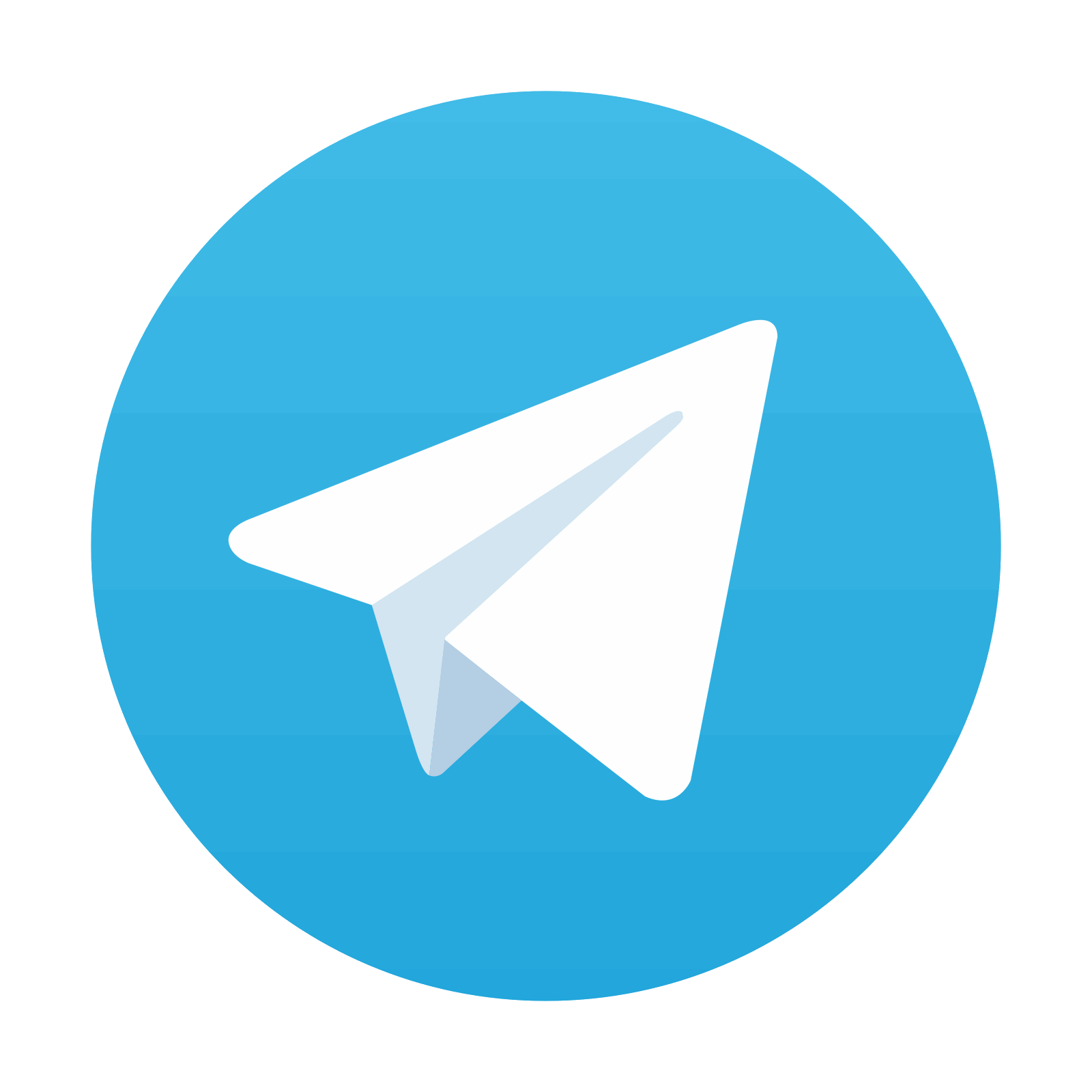
Stay updated, free dental videos. Join our Telegram channel

VIDEdental - Online dental courses
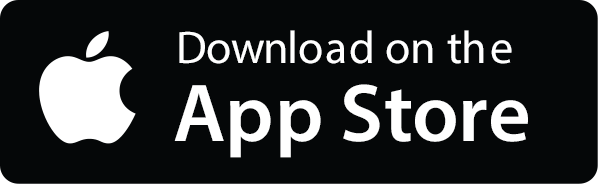
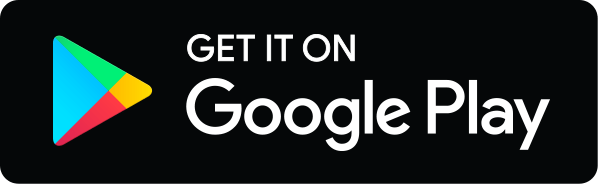