Enamel
Publisher Summary
This chapter describes dental enamel as a unique biological system that provides a hard surface for the teeth and enables them to reduce food to particles sufficiently small for effective attack by the digestive enzymes. Provided that it remains free from disease, human enamel can withstand a lifetime of crushing work without becoming fractured or completely worn away. Enamel is capable of performing this function only because of its most abundant constituent, hydroxyapatite, which gives it a hardness intermediate between that of iron and carbon steel. Three main aspects of tooth structure determine how physical stress is distributed within enamel. The gross morphology of the enamel cap defines the shape of both the occlusal surface and the amelodentinal junction. Forces within the enamel are transmitted to the underlying dentine, which is softer and more elastic than enamel, and acts as a firm cushion. The internal structure of enamel at histological and ultrastructural levels has an important bearing on its elastic behavior. This concerns the division of enamel into prismatic structures; the shapes, directions, and mutual interlacing of the prisms; and the arrangement of the crystallites within the prisms. In freshly secreted enamel, crystals first appear near the ameloblasts and, as soon as they do so, the enamel takes on the pattern of its final form, an intricate beautifully arranged two-phase system that may not only last throughout life but may also survive in fossil form for millions of years.
Three main aspects of tooth structure determine how physical stress is distributed within enamel. Firstly, the gross morphology of the enamel cap defines the shape of both the occlusal surface and the amelodentinal junction. Secondly, forces within the enamel are transmitted to the underlying dentine, which is softer and more elastic than enamel and acts as a firm cushion. Thirdly, the internal structure of enamel at histological and ultrastructural levels has an important bearing on its elastic behaviour. This concerns the division of enamel into prismatic structures, the shapes, directions and mutual interlacing of the prisms and finally the arrangement of the crystallites within the prisms (Figure 32.1).
Histological structure
Most of the hydroxyapatite crystals have their long axes approximately parallel to each other and almost parallel to the prism direction (Figure 32.1). Near the edges of the prisms the directions of the crystallites progressively deviate, the ends of the crystallites which are nearer the enamel surface being turned outwards away from the prism axis and towards the prism boundary. As a result of this progressive deviation there is, at the boundary, an abrupt change of crystallite orientation from one prism to the next. The prism boundaries are rendered visible under the microscope by this discontinuity of crystal orientation, rather than as was at one time thought, by a solid prism sheath of organic matter. However, electron micrographs of the earlier stages of enamel maturation do show that the region near the prism boundary contains fewer crystallites and consequently more of the organic phase than within the prisms.
The composition of mature enamel
The physical properties and chemical resistance of enamel are quite different from those of bone, dentine and cementum. At first this appears surprising since all four tissues are mineralized with hydroxyapatite but there are two important differences between enamel and the other tissues. Firstly, whereas bone, dentine and cementum contain some 20% by weight of collagen, mature enamel has only approximately 0·6% of organic matter (Table 32.1) while apatite accounts for approximately 99% of its dry weight. Secondly, the apatite crystals in enamel are approximately ten times wider and thicker (approx. 50 × 50 nm) and much longer than those that impregnate the calcified collagens, so that their volume is at least 1000 times greater. Such large crystals of apatite cannot be produced in synthetic systems at normal temperatures and pressures.
Table 32.1
A chemical balance sheet for mature human enamel
Percentage by weight | |||
Total inorganic matter | 95·0 | ||
Ash (700°C) | 91·7 | ||
Carbonate | 2·9 | ||
Total organic matter | 0·6 | ||
Total proteinaceous material | 0·35 | ||
Low molecular weight material | 0·06 | ||
Enamelins, etc. | 0·29 | ||
Citrate | 0·02 | ||
Lactate | 0·01 | ||
Carbohydrate | 0·0016 | ||
Fatty acid | 0·01 | ||
Total water | 4·0 | ||
‘Free’ water, lost at 100°C, in vacuo | 2·2 | ||
‘Bound’ water, lost between 300 and 400°C | 0·83 |
The values given in Table 32.1 are expressed on a weight basis whereas in terms of volume about 87·1% is occupied by the inorganic phase, 11·5% by water and 1·4% by organic matter. Mature enamel thus resembles a sintered mass of crystallites with the small spaces between them occupied by water. The small proportion of organic matter is mainly associated with the water in these spaces rather than with the crystallites. Although the aqueous phase occupies a relatively small part of the total volume, it is nevertheless, the continuous phase and is probably in communication throughout the enamel. All the same, in terms of hardness and behaviour under compressive loads, enamel behaves almost as though the inorganic phase were continuous, at least in contrast to dentine and bone where the crystallites are definitely separated by collagen, resulting in these tissues being softer and more deformable under load than enamel.
Gross composition of developing enamel and its changes during maturation
The composition of enamel at the beginning and end of maturation is given in Table 32.2, while the values for inorganic matter, water and organic matter in various intermediate stages of maturation are plotted against density in Figure 32.2.
Table 32.2
Gross composition of pig enamel at the lowest and highest degrees of mineralization
Lowest mineralization (Very soft; density 1·45) | Highest mineralization (Very hard; density 2·76) | |||||
mg mm−3 | %by weight | Volume* | mg mm−3 | %by weight | Volume* | |
Inorganic | 0·54 | 37·0 | 0·16 | 2·62 | 95·0 | 0·82 |
Organic | 0·27 | 19·0 | 0·20 | 0·05 | 1·8 | 0·04 |
Water | 0·64 | 44·0 | 0·64 | 0·12 | 4·3 | 0·12 |
Total | 1·45 | 100·0 | 1·00 | 2·79 | 101·1 | 0·98 |
*Volume in mm3 occupied by each constituent, calculated assuming densities of 3·18 for inorganic and 1·31 for organic material
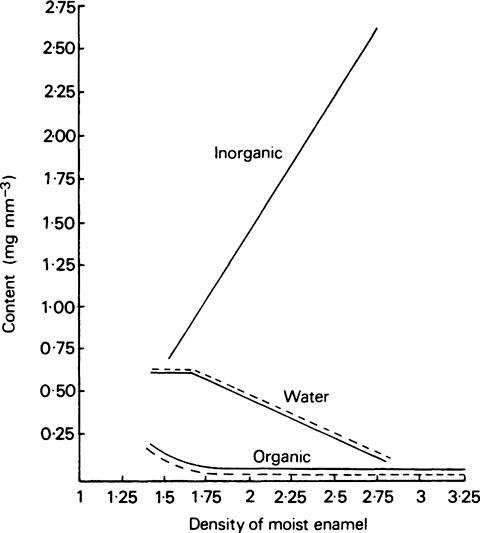
The total protein of developing enamel has an unusual amino acid composition (Figure 32.3) which distinguishes it from collagens, keratins and the protein of mature enamel. Possession of a unique protein system during its formative stages and the subsequent differential loss of its major protein components during maturation are characteristic features of enamel development which distinguish it from other tissues. The term amelogenins is used to designate those proteins that predominate in newly secreted enamel and which are removed during maturation of the enamel, a process which accompanies its transition from a material with the softness of jelly to one with the hardness of rock. The minor protein components of newly secreted enamel, known as enamelins, are not removed during maturation and largely persist in the fully mature enamel (Figure 32.4). Amelogenins and enamelins contrast strikingly in amino acid composition (cf. Tables 32.4 and 32.5).
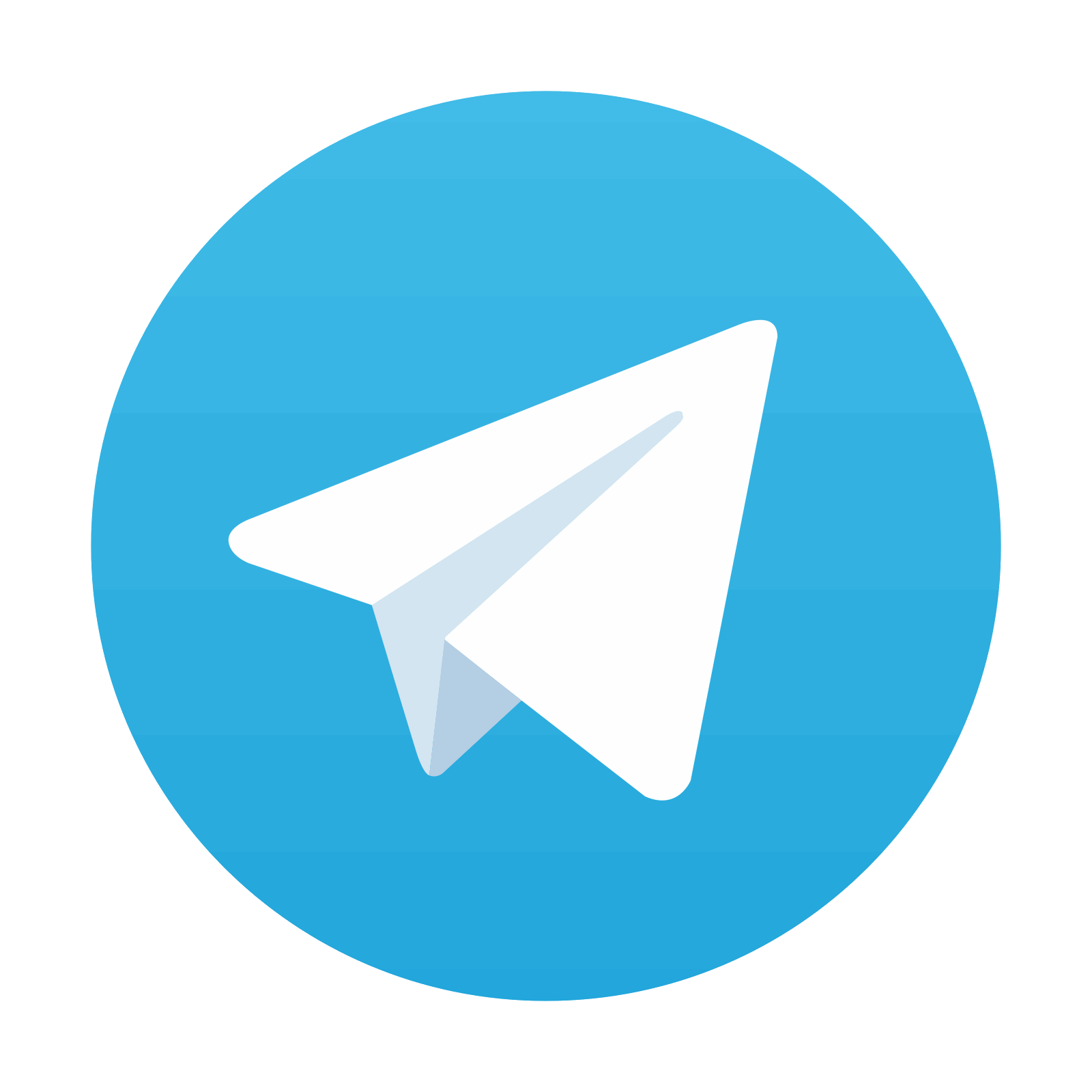
Stay updated, free dental videos. Join our Telegram channel

VIDEdental - Online dental courses
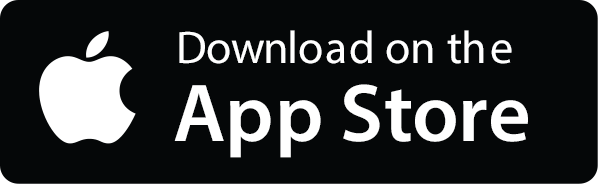
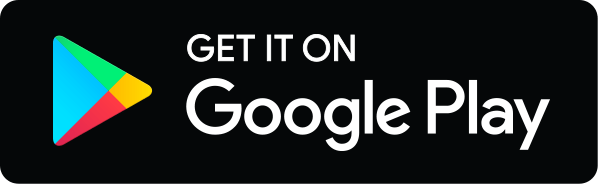