Abstract
Objective
To investigate the feasibility of iontophoretic delivery of large molecules across buccal mucosa, and to establish its potential for enhanced drug delivery.
Methods
Qualitative (6 h) and quantitative (8 and 36 h) assessment of porcine buccal mucosa, using a diffusion cell in vitro model, was carried out by fluorescent microscopy and UV/Vis spectroscopy respectively, with four fluorescently-labeled model species (3 and 10 kDa dextrans, 12 kDa parvalbumin and 66 kDa bovine serum albumin, BSA). Passive and iontophoresis parameters were obtained. The experimental iontophoresis data were compared with theoretical predictions.
Results
The two dextrans and parvalbumin showed enhanced permeation through buccal mucosa after anodal iontophoresis (1–6 h). Passive diffusion and cathodal iontophoresis resulted in minimal permeation. BSA could not be measured by either mode. Iontophoretic delivery profiles compared to passive delivery, had reduced time lags (30–50 versus ~270 min) and increased flux (~37 times faster). Time lag factor/enhancement ratio (TLF/ER) data confirmed that iontophoresis significantly enhanced permeation. The diffusion coefficients ( D , passive) for dextrans were significantly higher than for parvalbumin, with the converse obtained for solubility (C 0 ); permeability coefficients ( P ) were similar for all three species. Potential differences ( V ) for the two higher kDa species were significantly higher than for the lowest kDa species. Experimental and theoretical data were in reasonable agreement.
Significance
The experimental and theoretical data, confirming enhanced delivery of the model species via iontophoresis, gave a suitable basis for its potential application in the mouth, in a clinical setting and opens pathways to further research for delivering precious drugs topically and systemically.
1
Introduction
Topical delivery of therapeutic agents to skin and oral mucosa is a mainstay of dermatology and oral medicine practice. Skin is also widely used for systemic delivery, but maintaining good adhesion of delivery devices to moist oral mucosa long enough to achieve significant drug uptake has limited the extent to which the mouth has been used heretofore for systemic therapy. Here we report the application of iontophoresis in an in vitro porcine buccal mucosal model system to achieve accelerated delivery of active species. If successful this could expand the use of oral mucosa for systemic drug therapy.
Iontophoresis has been studied extensively for delivery through skin, via “polymeric skin patches” , and it is used widely for pain relief, hormone delivery, ophthalmology, ENT (ear–nose–throat) , and in the diagnosis and monitoring of illness . Protein iontophoresis has been used to deliver insulin in diabetes , calcitonoin in osteoporosis and human parathyroid hormone in Paget’s disesase . In the case of peptide delivery there have been reports of using ionotophoresis with analogs of vasopressin , somatorelin , somatostatin and luteinising releasing hormone . In contrast, the literature on the use of iontophoresis across oral mucosa is sparse , but the technique has been used in the treatment of herpes labialis, aphthous ulceration, dentin hypersensitive and for topical anesthesia .
Oral mucosa is significantly more permeable than skin , which makes it a plausible candidate site for topical and systemic drug delivery. If iontophoresis can be shown to achieve significantly enhanced transport of candidate species across bucccal mucosa, then the clinical potential of bucccal mucosa for drug delivery would be greatly increased. Porcine buccal mucosa has very similar permeability characteristics to human mucosa in terms of lipid content and composition, membrane morphology and permeability barrier function . These reasons make it a suitable model system for buccal permeability studies, and it has thus been used in this study.
Ionotophoresis enhances the rate of movement of ionic compounds across membranes by the application of an externally applied electric potential . It is a non invasive method that can be used for the controlled delivery of drugs, to achieve both a local effect and significant levels in the systemic circulation whilst avoiding the hepatic first pass effect . Furthermore, local delivery of large macromolecules such as peptides and protein drugs, which do not readily permeate skin, may become possible using this approach .
The iontophoretic system consists of the donor substance in its ionic form and a receptor solution separated by a limiting membrane, in this case oral mucosa, across which a voltage gradient is generated by connecting an anode and cathode to a voltage source that supplies direct constant electric current. The circuit is completed as the ions carry the current through the tissue barrier. Skin possesses a net negative charge so delivery of positively charged compounds is generally easier than negatively charged compounds .
The aim of this study was to use an in vitro model system based on porcine buccal mucosa to investigate the feasibility of iontophoresis as a method to achieve accelerated delivery of four model macromolecular species with different molecular weights (MW). The species used were labeled dextrans 3 kDa and 10 kDa; bovine serum albumin (BSA) 64 kDa and parvalbumin 12 kDa. The latter has a higher isoelectric point than the pH of the buccal tissue and can retain its charge during transport, which makes it a suitable candidate for iontophoresis ; passive delivery was used as a control. Dextrans were used as models because various molecular weights are available with essentially the same chemical structure, aiding the elucidation of transport of different MW compounds through oral mucosa. A mathematical model of passive diffusion was used to gain further insight into the mechanism by which any enhanced transport occurred.
2
Materials and methods
2.1
Materials
Samples of porcine buccal mucosa (Chealmeats, Brentwood, UK) were obtained and stored at −70 °C until use. Four fluorescently-labeled model compounds with different molecular weights were obtained from Molecular Probes, Inc., Eugene, OR, USA:
- (i)
Tetramethyl rhodamine labeled 3 kDa dextran (1 mg/ml in HEPES buffer ; pH 7.4, Melford Laboratories, UK).
- (ii)
Fluoresceinated 10 kDa dextran (1 mg/ml).
- (iii)
Alexa Fluor ® 488 conjugated parvalbumin (12 kDa; 1 mg/ml).
- (iv)
Tetramethyl rhodamine labeled bovine serum albumin (66 kDa; 5 mg/ml).
Electrical equipment: silver/silver chloride electrodes were used due to their ability to avoid large pH shifts, and prepared according to Green et al. .
2.2
Methods
2.2.1
Permeability measurements
A 7 mm diameter Teflon flow-through chamber (“diffusion chamber”; Crown Glass Company, USA; Fig. 1 ) was used in all passive and iontophoresis permeation studies. Samples of buccal mucosa were thawed and sandwiched between the donor and receptor compartments of the diffusion chamber (area of exposed epithelium was 0.38 cm 2 ; 7 mm diameter) and checked for leakage. Fluorescently labeled probes were applied to the epithelial surface in the donor chamber; the receptor compartment was kept completely immersed in HEPES buffer (30 ml, pH 7.4) in a petri dish.
2.2.2
Passive diffusion
Samples of receptor solution (1 ml) were collected hourly up 8 h after the application of the fluorescent probe and analysed by UV/vis spectroscopy (UV4 Spectrometer with a Vision software version 3.42, ATI Unicam Ltd., Cambridge, UK; wavelengths ranging from 190 nm to 900 nm).
2.2.3
Iontophoresis
Iontophoretic delivery was assessed using silver/silver chloride electrodes connected to a Phoresor ® II auto system power supply (Phoresor-850; Iomed Inc, Salt Lake City, USA). All four probes were anionic at pH 7.4 and therefore the cathode was suspended in the donor solution without touching the epithelium and the anode suspended in the receptor solution. Variations in the pH values (3 and 10), were introduced for parvalbumin because pH may reverse the protein charge depending on its isoelectric point.
The voltage drop across the tissue was adjusted automatically to keep the electric current constant, using the two-electrode system. When the resistance across the system increased, the power supply responded by increasing the voltage to drive the same current through the tissue. All 4 probes were tested at a current of 0.1 mA although 10 kDa dextran, 12 kDa parvalbumin and BSA, due to their molecular weights, were also investigated at higher currents (0.2 and 0.3 mA). The potential difference (V, volts) across the diffusion cell was measured at the beginning, mid-point and end of each experiment, using a digital voltmeter (Maintask Ltd., UK); electric parameter ( ν ) was also measured. Samples (1 ml) of the receptor solution were collected up to 8 h and permeation measured by UV-vis spectroscopy as described above.
In all the above experiments, passive diffusion studies were used as controls. Three replicates were conducted for each experimental series per active species. The total number of replicates per cell was 9 per active species.
2.2.4
Qualitative assessment of tissue permeation
Samples of the tissues used for both passive and iontophoretic delivery were also collected at 1, 2, 4 and 6 h, fixed in neutral buffered formalin and processed to paraffin wax. 5 μm sections were cut and viewed by fluorescence microscopy to qualitatively assess the extent of permeation through the tissue.
2.3
Analysis of data
2.3.1
Passive diffusion data
In the passive mode the Diffusion coefficient ( D ) and solubility ( C 0 ) of the four permeants were calculated. The resulting plot of the accumulated permeant (collected in the receptor cell), was characterized by the time lag, τ (intercept of the linear plot with time axis) and the slope of the graph (s). D was calculated using:
where D characterizes the rate of permeation through the membrane and L is the thickness of the membrane.
The slope ( s ) of the graph is given by:
where C 0 is the solubility of the permeant in the membrane, and A is the cross-sectional area of the tissue in the cell.
The product, DC 0 = P is defined as the permeability coefficient. Being the product of two other constants, it is of less significance.
2.3.2
Iontophoresis diffusion data
In the iontophoretic mode, the reduction in the time lag (time lag factor, TLF) and enhancement of the flux (Enhancement Ratio, ER) were determined for the permeants from Eqs. (4) and (5) respectively:
where the suffux ‘ i ’ refers to iontopheric delivery, and ‘ p ’ passive delivery; τ i = time lag for iontophoretic delivery, and τ p = time lag for passive delivery.
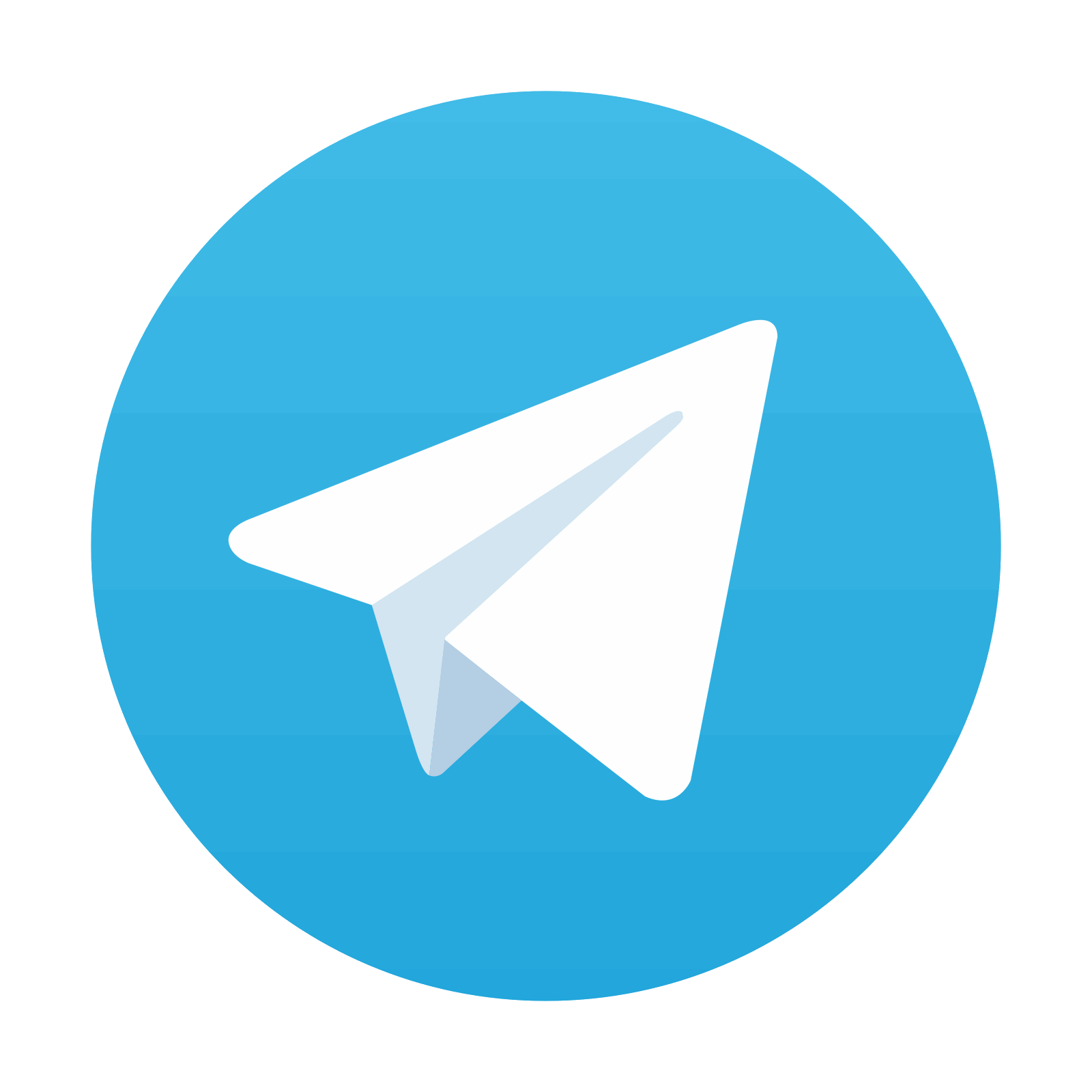
Stay updated, free dental videos. Join our Telegram channel

VIDEdental - Online dental courses
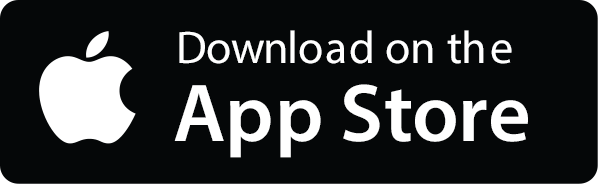
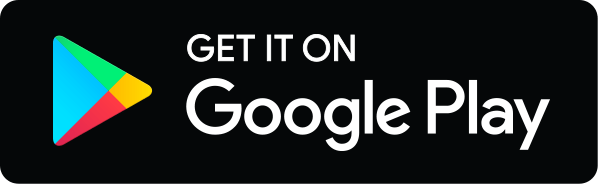