Abstract
Objectives
The objectives of this study were to develop a self-healing dental composite containing poly(urea-formaldehyde) (PUF) shells with triethylene glycol dimethacrylate (TEGDMA) and N,N -dihydroxyethyl- p -toluidine (DHEPT) as healing liquid, and to investigate the mechanical properties of the composite and its self-healing efficacy after water-aging for 6 months.
Methods
PUF microspheres were synthesized encapsulating a TEGDMA–DHEPT healing liquid. Composite containing 30% of a resin matrix and 70% of glass fillers by mass was incorporated with 0%, 2.5%, 5%, 7.5% and 10% of microcapsules. A flexural test was used to measure flexural strength and elastic modulus. A single edge V-notched beam method was used to measure fracture toughness ( K IC ) and self-healing efficacy. Specimens were water-aged at 37 °C for 1 day to 6 months and then tested for self-healing. Fractured specimens were healed while being immersed in water to examine self-healing efficacy, in comparison with that in air.
Results
Incorporation of up to 7.5% of microcapsules into the resin composite achieved effective self-healing, without adverse effects on the virgin mechanical properties of the composite ( p > 0.1). An excellent self-healing efficacy of 64–77% recovery was obtained (mean ± sd; n = 6). Six months of water-aging did not decrease the self-healing efficacy compared to 1 day ( p > 0.1). Exposure to water did not decrease the healing efficacy, compared to that healed in air ( p > 0.1).
Conclusions
A composite was developed with excellent self-healing efficacy even while being immersed in water. The self-healing efficacy did not decrease with increasing water-aging time for 6 months.
Clinical significance
The novel self-healing composite may be promising for dental applications to heal cracks, resist fracture, and increase the durability and longevity.
1
Introduction
Tooth-colored composites are increasingly used in dentistry as an alternative to amalgam to fill cavities . Of the 166 million tooth cavity restorations placed in 2005 in the United States, 52.5 million (31.6%) were amalgams, 77.3 million (46.6%) were composites, and 36.2 million (21.8%) were crowns . The increasing popularity of composites is a result of the superior esthetics, direct-filling capability, and improved physical and mechanical properties . However, studies showed that the durability of composite restorations needs to be improved . Half of all the restorations fail in less than 10 years, with fracture as one of the primary reasons . In a review on the longevity of composite restorations, it was observed that within 0–5 years after placement, the failure was primarily caused by restoration fracture, followed by secondary caries . The predominant reason for failure of composite restorations in larger cavities was also found to be fracture . Deterioration in restorations in vivo can be induced by fatigue from mastication, causing micro-flaws which can coalesce into cracks, eventually resulting in failure . Therefore, improving the fracture resistance of dental composites is of great significance. Extensive efforts have been undertaken in resin chemistry and fillers to improve the mechanical properties and performance of dental composite . However, inhibiting the crack propagation and fracture in dental composite restorations remains a challenge .
A novel approach is to develop self-healing materials that have an autonomous crack-healing ability, to repair damage and recover the load-bearing capability . An important method employs a liquid healing agent encapsulated in a polymeric shell to form microcapsules, which are then incorporated into a matrix material . When cracking occurs, the propagating crack would rupture the microcapsules, releasing the healing liquid which flows into the cracked planes via capillary action. Upon contacting the embedded catalyst in the matrix, the polymerization of the healing liquid is triggered, and the material is healed . In a previous study, dicyclopentadiene (DCPD) was encapsulated in a poly(urea-formaldehyde) (PUF) shell to form microcapsules . Grubb’s catalyst, a transition metal carbine complex, was used in an epoxy matrix to trigger the polymerization of the released DCPD, resulting in self-healing . Other researchers synthesized self-healing materials in fields varying from microelectronics to aerospace . In the dental field, a self-healing composite was developed with a healing ability to recover 57% of the virgin fracture toughness ( K IC ) . Attempts were also made to incorporate DCPD-containing microcapsules into dental resins . However, to date, there has been no further report on the use of DCPD and Grubb’s catalyst in dental materials, likely because the DCPD toxicity , Grubb’s catalyst toxicity, availability and high cost remain challenges. In a recent study , polyurethane (PU) shell-based triethylene glycol dimethacrylate (TEGDMA)-containing capsules were synthesized and added into a dental adhesive, showing that the dentin bond strength was not compromised. However, that study did not mention the use of a catalyst in the capsules, and self-healing was not reported .
More recently, novel self-healing poly(urea-formaldehyde) (PUF) microcapsules containing polymerizable TEGDMA and N,N -dihydroxyethyl- p -toluidine (DHEPT) were synthesized and incorporated into dental resin . The self-healing resin containing microcapsules had human fibroblast viability similar to control cells in culture medium without resin, indicating little cytotoxicity in vitro . The composite containing these microcapsules achieved good self-healing efficacy . However, in these pilot studies, the cured specimens were immersed in water for only 1 day before testing. Dental composites in the oral environment are required to survive being wet for long periods of time. It is not clear how water-aging would affect the self-healing resin matrix, the microcapsules and the encapsulated healing liquid, thereby affecting the healing liquid polymerization with the initiator in the matrix. A literature search revealed no report on the effect of water-aging on the self-healing properties of composites.
The objectives of this study were to develop a self-healing dental composite containing PUF microcapsules with TEGDMA and DHEPT healing liquid, and to investigate the mechanical properties of the composite and its self-healing efficacy after the composite was water-aged for up to 6 months. The following hypotheses were tested: (1) Incorporation of the new microcapsules into the dental composite would not compromise the original mechanical properties of composite without microcapsules; (2) the self-healing efficacy would be directly proportional to the microcapsule filler level in the composite; (3) water-aging for 6 months would not reduce the composite’s healing efficacy, compared to that at 1 day.
2
Materials and methods
2.1
Synthesis of self-healing microcapsules
Microcapsules were synthesized via in situ polymerization of formaldehyde and urea as described previously . Briefly, DHEPT (Sigma–Aldrich, St. Louis, MO) at 1% (all mass fractions) was added to TEGDMA monomer (Esstech, Essington, PA). At room temperature, 50 mL of distilled water and 13 mL of a 2.5% aqueous solution of ethylene-maleic anhydride (EMA) copolymer (Sigma–Aldrich) were mixed in a 250 mL round-bottom glass flask . The flask was suspended in a water bath on a hotplate (Isotemp, Fisher Scientific, Pittsburg, PA). The EMA solution was used as a surfactant to form an “oil-in-water” emulsion (“oil” being the TEGDMA–DHEPT monomer). Under agitation by a magnetic stir bar (diameter = 7.8 mm, length = 50 mm, Fisher Scientific) at 300 rpm, the shell-forming material urea (1.25 g), ammonium chloride (0.125 g) and resorcinol (0.125 g) (Sigma–Aldrich) were added into the solution. Resorcinol was added in the reaction of shell formation to enhance the rigidity of the shell . The pH was adjusted to 3.5 via drop-wise addition of 1 M sodium hydroxide solution. Then, the agitation rate was increased to 400 rpm, and 30 mL of the TEGDMA–DHEPT liquid was added into the flask. A stabilized emulsion of fine TEGDMA–DHEPT droplets was formed after 10 min of agitation. Then, 3.15 g of a 37% aqueous solution of formaldehyde (Sigma–Aldrich) was added, and the flask was sealed with aluminum foil to prevent evaporation. The temperature of the water bath was raised to 55 °C and the shell material was isothermally polymerized for 4 h (h) under continuous agitation . In this process, ammonium chloride catalyzed the reaction of urea with formaldehyde to form PUF at the oil–water interface to develop the shell . The microcapsules thus obtained were rinsed with water and acetone, vacuum-filtered, and air-dried for 24 h. The microcapsules were examined by optical microscope (TE2000-S, Nikon, Japan), and their sizes were measured with an image analysis software (Nis-Elements BR2.30, Nikon). The microcapsules were also examined with scanning electronic microscopy (SEM, Quanta 200, FEI, Hillsboro, OR).
2.2
Development of self-healing composite
Bisphenol A glycidyl dimethacrylate (BisGMA) and TEGDMA (Esstech) were mixed at a mass ratio of 1:1, and rendered light-curable by adding 1% by mass of a photo-initiator phenyl-bis(2,4,6-trimethylbenzoyl)phosphine oxide (BAPO, Sigma–Aldrich) . Then 0.5% by mass of benzoyl peroxide (BPO) (Sigma–Aldrich) was dissolved in the mixture. BPO serves as an initiator to react with the DHEPT in the healing liquid to trigger the self-healing polymerization. The glass fillers for the composite were barium boroaluminosilicate with a median particle size of 1.45 μm (Caulk/Dentsply, Milford, DE), which were silanized with 4% 3-methacryloxypropyltrimethoxysilane and 2% n -propylamine . These glass particles were mixed into the resin at a filler level of 70% by mass to form a cohesive paste. Self-healing microcapsules were then mixed into this composite paste. The following microcapsule mass fractions in composite were used: 0%, 2.5%, 5%, 7.5% or 10%, resulting in five composites, respectively. Each group had six specimens for each experiment under each tested condition ( n = 6). The composite paste was placed into a mold of 2 × 2 × 25 mm and covered with Mylar strips. Each specimen was photo-cured (Triad 2000, Dentsply, York, PA) for 1 min on each open side of the mold.
2.3
Flexural strength and elastic modulus measurement
The cured specimens were stored in distilled water at 37 °C for 24 h. A Universal Testing Machine (5500R, MTS, Cary, NC) was used to fracture the specimens in three-point flexure with a span of 10 mm at a crosshead speed of 1 mm/min. Flexural strength was S = 3P max L /(2 bh 2 ), where P max is load-at-failure, L is span, b is specimen width and h is thickness. Elastic modulus was E = ( P / d )( L 3 /4 bh 3 ]), where load P divided by displacement d is the slope in the linear elastic region of the load–displacement curve. The specimens were tested within a few minutes after being taken out of water and were fractured while being wet.
2.4
Fracture toughness ( K IC ) and self-healing efficacy measurements
The cured composites were stored in water at 37 °C for 24 h. A single edge V-notched beam (SEVNB) method was used to measure the K IC . A notch depth of 500 μm was machined into each specimen using a diamond blade with a thickness of 150 μm (Buehler, Lake Bluff, IL) . Then, a 3-μm diamond paste (Buehler) was placed into the notch tip, and a new razor blade was used to cut the notch further to a total depth of about 700–800 μm . This resulted in a relatively sharp notch with a tip radius of about 20 μm . The notch length was measured using an optical microscope (TE2000-S) on both sides of the specimen and averaged. Specimens were tested in three-point flexure with the notch on the tensile side, and the loading pin aligned with the notch under a 3× magnifier. K IC was calculated following an established method . This yielded the virgin fracture toughness of the specimen, K IC-virgin .
To test self-healing efficacy, immediately following fracture, the two halves of the specimen were placed back into the mold to ensure a good contact of the two fractured planes, following a previous study . This would be similar to the case of a composite restoration in a tooth cavity, where the cracked restoration would stay in the tooth cavity to allow the released healing liquid to heal the crack. The healing of a completely cracked specimen would be a more rigorous test than the healing of a partial or small crack in the specimen. Because the fracture ruptured the microcapsules in the composite, the released TEGDMA–DHEPT liquid from the microcapsules would react with the BPO in the resin matrix. This would cause the polymerization of the released liquid to heal and bond the two cracked planes into a cohesive specimen . The healing specimens were incubated in a humidor at 37 °C in air for 24 h. The healed specimen was tested, which yielded the healed toughness, K IC-healed . The self-healing efficacy ( η ) was assessed following previous studies : η = K IC-healed / K IC-virgin . Selected fracture surfaces were sputter-coated with gold and examined in SEM (Quanta 200).
2.5
Water-aging of self-healing composite specimens
The composite with 7.5% microcapsules had a flexural strength similar to that with 0% microcapsules, while having a high self-healing efficacy; the composite with 10% microcapsules had a lower flexural strength. Therefore, the composite containing 7.5% microcapsules was selected for water-aging test for 6 months. The cured specimens were immersed in distilled water at 37 °C for 1 day and 1, 2, 3 and 6 months. For each time period, six specimens were immersed in 200 mL of water in a sealed polyethylene container. The water was changed once every week. After each water-aging time period, the K IC-virgin was measured. Then the two broken halves of each specimen were allowed to heal as described above, and the K IC-healed was measured. This yielded the self-healing efficacy η vs. water-aging time for the composite.
2.6
Self-healing while being immersed in water
The composite containing 7.5% microcapsules was tested. In the previous sections, the healing specimens were placed in a humidor in air with 100% relative humidity for the two broken halves to heal, not immersed in water. It is unclear how water immersion would affect the self-healing process. Therefore, in this section, after the specimen was fractured and the two halves were placed back into the mold, the construct was immediately immersed in water at 37 °C for 24 h, while the specimen healed. K IC-healed was then measured as described above.
2.7
Statistical analysis
One-way and two-way analyses-of-variance (ANOVA) were performed to detect the significant effects. Tukey’s multiple comparison was used to compare the data at p of 0.05.
2
Materials and methods
2.1
Synthesis of self-healing microcapsules
Microcapsules were synthesized via in situ polymerization of formaldehyde and urea as described previously . Briefly, DHEPT (Sigma–Aldrich, St. Louis, MO) at 1% (all mass fractions) was added to TEGDMA monomer (Esstech, Essington, PA). At room temperature, 50 mL of distilled water and 13 mL of a 2.5% aqueous solution of ethylene-maleic anhydride (EMA) copolymer (Sigma–Aldrich) were mixed in a 250 mL round-bottom glass flask . The flask was suspended in a water bath on a hotplate (Isotemp, Fisher Scientific, Pittsburg, PA). The EMA solution was used as a surfactant to form an “oil-in-water” emulsion (“oil” being the TEGDMA–DHEPT monomer). Under agitation by a magnetic stir bar (diameter = 7.8 mm, length = 50 mm, Fisher Scientific) at 300 rpm, the shell-forming material urea (1.25 g), ammonium chloride (0.125 g) and resorcinol (0.125 g) (Sigma–Aldrich) were added into the solution. Resorcinol was added in the reaction of shell formation to enhance the rigidity of the shell . The pH was adjusted to 3.5 via drop-wise addition of 1 M sodium hydroxide solution. Then, the agitation rate was increased to 400 rpm, and 30 mL of the TEGDMA–DHEPT liquid was added into the flask. A stabilized emulsion of fine TEGDMA–DHEPT droplets was formed after 10 min of agitation. Then, 3.15 g of a 37% aqueous solution of formaldehyde (Sigma–Aldrich) was added, and the flask was sealed with aluminum foil to prevent evaporation. The temperature of the water bath was raised to 55 °C and the shell material was isothermally polymerized for 4 h (h) under continuous agitation . In this process, ammonium chloride catalyzed the reaction of urea with formaldehyde to form PUF at the oil–water interface to develop the shell . The microcapsules thus obtained were rinsed with water and acetone, vacuum-filtered, and air-dried for 24 h. The microcapsules were examined by optical microscope (TE2000-S, Nikon, Japan), and their sizes were measured with an image analysis software (Nis-Elements BR2.30, Nikon). The microcapsules were also examined with scanning electronic microscopy (SEM, Quanta 200, FEI, Hillsboro, OR).
2.2
Development of self-healing composite
Bisphenol A glycidyl dimethacrylate (BisGMA) and TEGDMA (Esstech) were mixed at a mass ratio of 1:1, and rendered light-curable by adding 1% by mass of a photo-initiator phenyl-bis(2,4,6-trimethylbenzoyl)phosphine oxide (BAPO, Sigma–Aldrich) . Then 0.5% by mass of benzoyl peroxide (BPO) (Sigma–Aldrich) was dissolved in the mixture. BPO serves as an initiator to react with the DHEPT in the healing liquid to trigger the self-healing polymerization. The glass fillers for the composite were barium boroaluminosilicate with a median particle size of 1.45 μm (Caulk/Dentsply, Milford, DE), which were silanized with 4% 3-methacryloxypropyltrimethoxysilane and 2% n -propylamine . These glass particles were mixed into the resin at a filler level of 70% by mass to form a cohesive paste. Self-healing microcapsules were then mixed into this composite paste. The following microcapsule mass fractions in composite were used: 0%, 2.5%, 5%, 7.5% or 10%, resulting in five composites, respectively. Each group had six specimens for each experiment under each tested condition ( n = 6). The composite paste was placed into a mold of 2 × 2 × 25 mm and covered with Mylar strips. Each specimen was photo-cured (Triad 2000, Dentsply, York, PA) for 1 min on each open side of the mold.
2.3
Flexural strength and elastic modulus measurement
The cured specimens were stored in distilled water at 37 °C for 24 h. A Universal Testing Machine (5500R, MTS, Cary, NC) was used to fracture the specimens in three-point flexure with a span of 10 mm at a crosshead speed of 1 mm/min. Flexural strength was S = 3P max L /(2 bh 2 ), where P max is load-at-failure, L is span, b is specimen width and h is thickness. Elastic modulus was E = ( P / d )( L 3 /4 bh 3 ]), where load P divided by displacement d is the slope in the linear elastic region of the load–displacement curve. The specimens were tested within a few minutes after being taken out of water and were fractured while being wet.
2.4
Fracture toughness ( K IC ) and self-healing efficacy measurements
The cured composites were stored in water at 37 °C for 24 h. A single edge V-notched beam (SEVNB) method was used to measure the K IC . A notch depth of 500 μm was machined into each specimen using a diamond blade with a thickness of 150 μm (Buehler, Lake Bluff, IL) . Then, a 3-μm diamond paste (Buehler) was placed into the notch tip, and a new razor blade was used to cut the notch further to a total depth of about 700–800 μm . This resulted in a relatively sharp notch with a tip radius of about 20 μm . The notch length was measured using an optical microscope (TE2000-S) on both sides of the specimen and averaged. Specimens were tested in three-point flexure with the notch on the tensile side, and the loading pin aligned with the notch under a 3× magnifier. K IC was calculated following an established method . This yielded the virgin fracture toughness of the specimen, K IC-virgin .
To test self-healing efficacy, immediately following fracture, the two halves of the specimen were placed back into the mold to ensure a good contact of the two fractured planes, following a previous study . This would be similar to the case of a composite restoration in a tooth cavity, where the cracked restoration would stay in the tooth cavity to allow the released healing liquid to heal the crack. The healing of a completely cracked specimen would be a more rigorous test than the healing of a partial or small crack in the specimen. Because the fracture ruptured the microcapsules in the composite, the released TEGDMA–DHEPT liquid from the microcapsules would react with the BPO in the resin matrix. This would cause the polymerization of the released liquid to heal and bond the two cracked planes into a cohesive specimen . The healing specimens were incubated in a humidor at 37 °C in air for 24 h. The healed specimen was tested, which yielded the healed toughness, K IC-healed . The self-healing efficacy ( η ) was assessed following previous studies : η = K IC-healed / K IC-virgin . Selected fracture surfaces were sputter-coated with gold and examined in SEM (Quanta 200).
2.5
Water-aging of self-healing composite specimens
The composite with 7.5% microcapsules had a flexural strength similar to that with 0% microcapsules, while having a high self-healing efficacy; the composite with 10% microcapsules had a lower flexural strength. Therefore, the composite containing 7.5% microcapsules was selected for water-aging test for 6 months. The cured specimens were immersed in distilled water at 37 °C for 1 day and 1, 2, 3 and 6 months. For each time period, six specimens were immersed in 200 mL of water in a sealed polyethylene container. The water was changed once every week. After each water-aging time period, the K IC-virgin was measured. Then the two broken halves of each specimen were allowed to heal as described above, and the K IC-healed was measured. This yielded the self-healing efficacy η vs. water-aging time for the composite.
2.6
Self-healing while being immersed in water
The composite containing 7.5% microcapsules was tested. In the previous sections, the healing specimens were placed in a humidor in air with 100% relative humidity for the two broken halves to heal, not immersed in water. It is unclear how water immersion would affect the self-healing process. Therefore, in this section, after the specimen was fractured and the two halves were placed back into the mold, the construct was immediately immersed in water at 37 °C for 24 h, while the specimen healed. K IC-healed was then measured as described above.
2.7
Statistical analysis
One-way and two-way analyses-of-variance (ANOVA) were performed to detect the significant effects. Tukey’s multiple comparison was used to compare the data at p of 0.05.
3
Results
All data in this study were verified to have a normal distribution. The microcapsule diameters were measured (mean ± sd; n = 200) to be 70 ± 24 μm. An SEM image of microcapsules is shown in Fig. 1 (A). These microcapsules were incorporated into the composite, and the mechanical properties of the composite after water-immersion for 1 day are plotted in (B) for flexural strength, and (C) for elastic modulus (mean ± sd; n = 6). There was no significant difference in strength and elastic modulus with microcapsule mass fractions from 0% to 7.5% ( p > 0.1). However, the mechanical properties at 10% microcapsules were significantly lower than the other groups ( F = 7.45, p = 0.0004 for flexural strength; F = 23.85, p < 0.0001 for elastic modulus; one-way ANOVA).
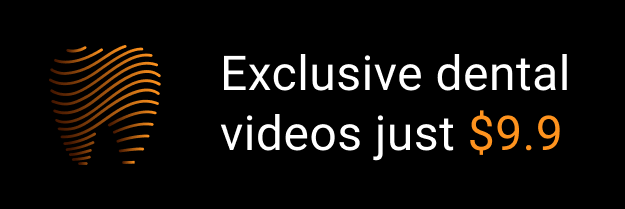