Abstract
Objective
We investigated the effects of the surface properties of polymer-based restorative materials on early adhesion of Streptococcus mutans (UA159) in vitro .
Methods
Four direct polymer-based restorative materials, including a nanoparticle restorative (Filtek™ Z350, 3 M ESPE, USA), a nano hybrid universal restorative (Filtek™ Z250 XT, 3 M ESPE, USA), a low shrink posterior restorative (Filtek™ P90, 3 M ESPE, USA) and a polymer-based pre-reacted glass ionomer (Beautifil II, Shofu, Japan), were selected. After polishing under different conditions, surface morphology was examined using scanning electron microscopy. Surface roughness (SR), water contact angle (CAW) and surface free energy (SFE) were determined by profilometry and the sessile drop method. Early adhesion of S. mutans was investigated using confocal laser scanning microscopy. The area occupied by adherent bacteria (A%) was calculated with COMSTAT2 software. The correlations between A% and SR, CAW, and SFE were analyzed by linear regression using SPSS 20.0 software at a significance level of 0.05.
Results
The value of A% was strongly correlated with SR ( r = 0.893, P < 0.01) for surface roughness (Ra) of 0.02–0.80 μm, whereas a weaker correlation was obtained between A% and SR when Ra ≤ 0.20 μm ( r = 0.643, P < 0.01). On super smooth surfaces (0.02 μm ≤ Ra ≤ 0.06 μm), SR did not influence early bacterial adhesion ( r = 0.001, P > 0.05), a medium positive correlation between A% and SFE was obtained ( r = 0.426, P < 0.01), and no correlation between A% and CAW was found ( r = −0.028, P > 0.05)
Conclusions
Early adhesion of S. mutans on direct polymer-based restorative materials was mainly affected by SR. SFE influenced early adhesion of S. mutans on super smooth surfaces, while hydrophobicity did not.
1
Introduction
Dental plaque or biofilms are the main causes of common dental diseases, and involve microbial adhesion to dental hard and soft tissues as well as restorative biomaterials . The mechanisms whereby oral bacteria adhere to solid surfaces are influenced by the unique adhesive properties of the adherent bacteria as well as the properties of the adhered substances. Secondary caries development is closely related to the presence of cariogenic biofilms on dental restorative materials . Previous studies have reported that the three-dimensional structure and thickness of dental biofilms, as well as the composition and activity of dental plaque influenced by restorative materials . In addition to the different compositions of diverse substances, the surface properties of restorative materials play a pivotal role in the early adhesion process of bacteria .
Polymer-based dental composites are an aesthetic alternative to amalgam, and are composed of a hydrophobic resin matrix containing hydrophilic filler particles, which implies they form a heterogeneous surface. The polymer composition, and the size and shape of fillers strongly influence the surface properties of polymer-based composites . In recent years, to improve the mechanical, physical, and biological properties of polymer-based composites, a multitude of modified polymer-based restorative materials have been developed. For example, an innovative matrix-modified resin composite based on siloxane and oxirane was developed to decrease polymerization shrinkage. The resulting siloranes exhibit similar or better mechanical and physical properties and biocompatibility characteristics than those of conventional methacrylate-based composite resins . Regarding development of inorganic fillers, nanofilled resin composites exhibit excellent hardness, toughness, and polishing characteristics. Meanwhile, pre-reacted glass-ionomer (PRG) bioactive fillers have been fabricated by the acid–base reaction between a fluoroaluminosilicate glass and polyalkenoic acid in the presence of water . The addition of bioactive fillers results in fluoride releasing/recharging properties to prevent secondary caries . These modified polymer-based composites possessing varied surface properties may influence bacterial adhesion differently.
Oral bacterial adhesion occurs in the following four phases : (1) transport of a bacterium to the surface, (2) initial adhesion with reversible and irreversible stages, (3) attachment by specific interactions, and (4) colonization to form a biofilm. Non-specific chemical and electrovalent interactions between the surface and bacteria, like Van der Waals forces, Coulomb forces, and hydrophobic, electrostatic and Lewis acid–base interactions, affect this process . The physicochemical surface properties of restorative materials, such as their surface roughness (SR), hydrophobicity, surface free energy (SFE), and surface electrochemistry, may affect dental plaque formation . It has been reported that SR is the dominant factor influencing bacterial adhesion . An increase in SR from 0.2 to 0.8 μm of intraoral hard surfaces had a significant effect on in vivo plaque formation (supra- and subgingivally) on abutments . Therefore, a threshold surface roughness (Ra) was suggested. When Ra ≤ 0.2 μm, it was considered that SR had a negligible effect on bacterial adhesion in vivo . However, with the development of polymer-based materials and polishing technology, the bacterial adhesion on polished materials below the threshold value of Ra has varied considerably . This indicates that when Ra ≤ 0.2 μm, factors other than SR influence bacterial adhesion.
It has been observed that SFE and hydrophobicity also affect bacterial adhesion on polymer-based materials . However, the published results are quite controversial . Possible reasons for these controversial results may be the heterogeneous composition of polymer-based composite surfaces, and the methods used to evaluate SFE and hydrophobicity. It has been reported that the SFE and hydrophobicity (contact angle of water, CAW) values calculated with the sessile drop method is more accurate when the surface is smoother . Therefore, the effects of SFE and CAW on bacterial adhesion should be interpreted with caution. Furthermore, it has been considered that the effects of SFE and CAW on bacterial adhesion may be confounded by the dominant role of SR, especially when Ra is above 0.06 μm . Therefore, it remains unclear if the SFE and/or hydrophobicity of polymer-based composite materials influence bacterial adhesion, especially on smooth surfaces.
In this study, the SR, CAW, and SFE of polymer-based restorative materials, along with early adhesion of Streptococcus mutans on them are investigated. The correlations between these factors are analyzed. The aim of this study is to gain more insight into the surface properties responsible for initial adhesion of S. mutans on polymer-based restorative materials. We hypothesize that the levels of early adhesion of S. mutans are influenced not only by SR, but also by hydrophobicity and SFE, especially on smooth surfaces.
2
Materials and methods
2.1
Preparation of polymer-based restorative material specimens
Four polymer-based restorative materials were used in this study, including a nanoparticle restorative (Filtek™ Z350 [Z350], 3M ESPE, USA), a nano-hybrid universal restorative (Filtek™ Z250 XT [Z250 XT], 3 M ESPE, USA), a low-shrink posterior restorative based on siloxane and oxirane (Filtek™ P90 [P90], 3 M ESPE, USA) and a polymer-based pre-reacted glass ionomer (Beautifil II [BF], Shofu, Japan). The parameters of these materials are summarized in Table 1 . Each polymer-based material was placed in a fabricated Teflon model (4 × 4 × 2 mm) and covered with a Mylar film. A glass slide was placed over the Mylar film, and pressure was applied. After curing under high-intensity light (1100 mW/cm 2 ) on both sides for 40 s (LEDition, Ivoclar vivadent), all specimens were stored in distilled water at 37 °C for 24 h, and then polished with a polisher (AutoMet ® 250 Grinder-Polisher Family, Buehler, USA). A continuous force of 5 N in the vertical direction rotation speed of 60 rpm/150 rpm at contrary motion was applied. The specimens were sequentially polished with 11-μm grit (grain 1200 wet abrasive paper disc, White Dove, China), 9-μm grit, 3-μm grit, 1-μm disc (diamond disc, Lapping Film ϕ250 mm, Grish, China), and finally nano-silicon dioxide fabric (Polishing Pad S0-PGZ-2 ϕ250 mm, GRISH, China). After polishing, specimens were rinsed with distilled water and ultrasonically cleaned in distilled water for 3 min. According to the final polishing grit, the specimens were divided into five groups for each material: Group 1: 11-μm grit; Group 2: 9-μm grit; Group 3: 3-μm grit; Group 4: 1-μm grit; Group 5: nano grit.
Type | Brand name | Matrix composition | Filler composition | Manufacturer |
---|---|---|---|---|
Nanoparticle composite | Filtek™ Z350 [Z350] | Bis-GMA, Bis-EMA, UDMA, TEGDMA | 5–20 nm non-agglomerated silica and 5–20 nm zirconium/silica nanoagglomerate. 0.6-1.4 μm agglomerated particles. Loading percentage by weight: 82% | 3M ESPE, St. Paul, MN, USA |
Nano hybrid composite | Filtek™ Z250 XT [Z250 XT] | Bis-GMA, Bis-EMA, UDMA, TEGDMA, PEGDMA | 20 nm surface modified silica and 0.1–10 μm surface modified zirconia/silica particles. Loading percentage by weight: 82% | 3M ESPE, St. Paul, MN, USA |
Silorane composite | Filtek™ P90 [P90] | Bis-3,4-epoxy cyclohexylethyl phenyl methyl silane-, 3,4-epoxy cyclohexyl cyclopolymethyl siloxane, di- and epoxy-functional oligosiloxane | trifluoride ITRE, 0.1–2 μm quartz particles. Loading percentage by weight: 76% | 3M ESPE, St. Paul, MN, USA |
Giomer | Beautifil II [BF] |
Bis-GMA, TEGDMA | 0.01–4.0 μm multi-functional glass filler and S-PRG filler based on fluoroboroaluminosilicate glass. Loading percentage by weight: 81% | Shofu Inc., Japan |
2.2
Surface morphology
The surface morphology of specimens was examined with a scanning electron microscope (SEM, S-4800, Hitachi, Japan). After mounting on aluminum stubs and sputter coating with gold, the specimens were observed with 1000× magnification.
2.3
Surface roughness measurement
The SR of each specimen was measured using a surface profilometer (Surftest SJ-401, Mitutoyo, Kanagawa, Japan) with a stress force of 0.75 mN, standard cutoff of 1.0 mm, transverse length of 0.8 mm, amplitude height of 2.5 μm, and stylus speed of 0.5 mm/s. Two measurements of Ra were performed at cross directions for each specimen, and the numerical average of these values is reported.
2.4
Hydrophobicity and surface free energy determination
Hydrophobicity was determined by measuring the water contact angles with an automated contact angle measurement device (OCA20, DataPhysics, Germany). Right and left contact angles for droplets (1 μL) were averaged and are reported as CAW (°).
To determine the SFE of each specimen, three liquids with different polarities were chosen for contact angle measurements, including distilled water, glycerol, and ethylene glycol. The SFE of each specimen is listed in Table 2 . The total SFE (γ TOT ) for each sample was calculated according to the approach proposed by van Oss et al.:
γ S T O T ( m N / m ) = γ S D + γ S P ;
γ S P = 2 ( γ S + γ S − ) 0.5 ;
( 1 + cos θ ) γ L T O T = 2 ( γ S D γ L D ) 0.5 + 2 ( γ L − γ S + ) 0.5 + 2 ( γ L + γ S − ) 0.5 .
Liquid | Surface tension data (mN·m −1 ) | |||
---|---|---|---|---|
<SPAN role=presentation tabIndex=0 id=MathJax-Element-4-Frame class=MathJax style="POSITION: relative" data-mathml='γLTOT’>γTOTLγLTOT γ L T O T |
<SPAN role=presentation tabIndex=0 id=MathJax-Element-5-Frame class=MathJax style="POSITION: relative" data-mathml='γLD’>γDLγLD γ L D |
<SPAN role=presentation tabIndex=0 id=MathJax-Element-6-Frame class=MathJax style="POSITION: relative" data-mathml='γL+’>γ+LγL+ γ L + |
<SPAN role=presentation tabIndex=0 id=MathJax-Element-7-Frame class=MathJax style="POSITION: relative" data-mathml='γL−’>γ−LγL− γ L − |
|
Glycerol | 64 | 34 | 3.92 | 57.4 |
Ethylene glycol | 48 | 29 | 3 | 30.1 |
Distilled water | 72.8 | 21.8 | 25.5 | 25.5 |
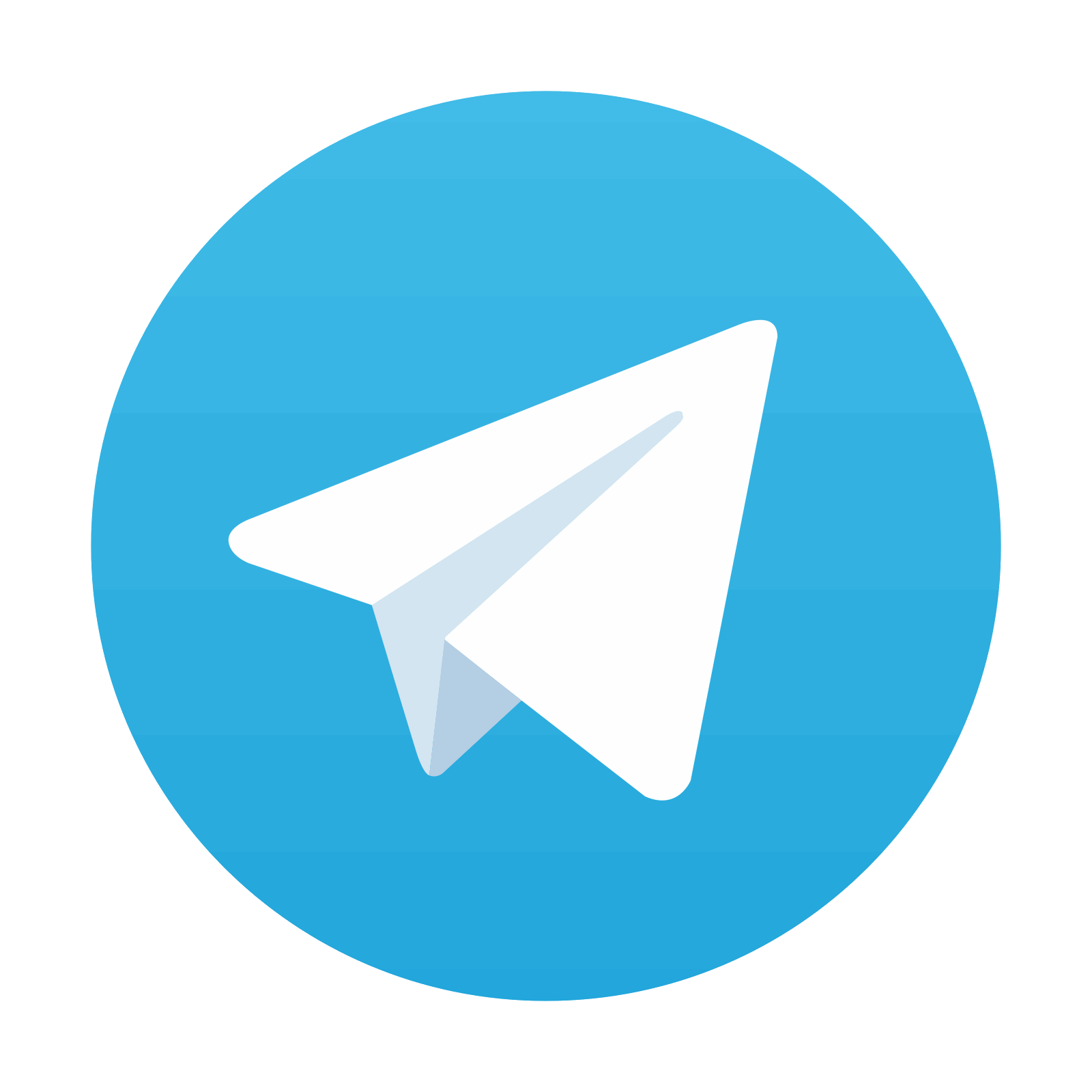
Stay updated, free dental videos. Join our Telegram channel

VIDEdental - Online dental courses
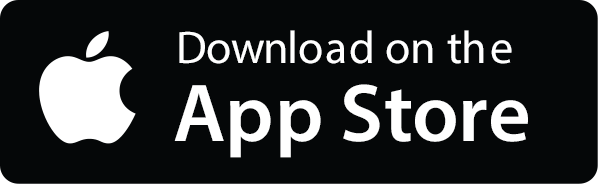
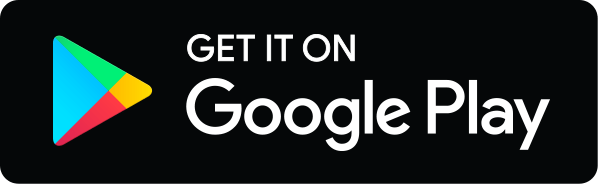
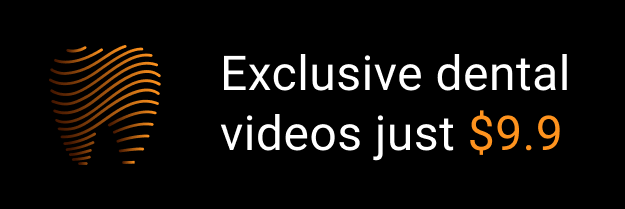