Abstract
Objectives
To evaluate the effect of specimen thickness, pretreatment and hydrothermal aging on the biaxial flexural strength (BFS) of lithium di-silicate glass (e.max Cad) and magnesia-stabilized zirconia (ZirMagnum) ceramic discs.
Methods
The e.max Cad discs was studied: i) crystallized, ii) crystallized and glazed and iii) crystallized, glazed and unglazed side etched with hydrofluoric acid. The ZirMagnum discs were studied: i) as delivered, ii) after sandblasting and iii) after heat treatment similar to veneering. Hydrothermal aging was simulated by autoclave treatment.
Results
The BFS of all the ZirMagnum specimens was superior (p < 0.001) to all the e.max Cad specimens. Glazing the 0.4 mm e.max Cad discs reduced (p < 0.05) their BFS compared with the unglazed 0.8 mm specimens, whereas glazing of 0.8 mm discs had no influence (p > 0.05) on the strength. Etching and autoclaving of e.max Cad did not affect (p > 0.05) the BFS. For ZirMagnum sandblasting with 0.2 MPa or 0.6 MPa did not influence the biaxial flexural strength (p > 0.05), whereas heat treatment reduced (p < 0.01) the BFS of 0.6 MPa sandblasted ZirMagnum. Autoclaving reduced the strength (p < 0.05) compared with ZirMagnum as delivered, whereas autoclaving of the 0.6 MPa sandblasted and heat-treated specimens did not influence (p > 0.05) the BFS. Glazing, etching and sandblasting increased (p < 0.05) surface roughness.
Conclusions
The effects of glazing, heat treatment, aging and mechanical treatment of the materials evaluated should be considered since their strength could be affected.
Clinical significance
Mechanical properties of restorations made from prefabricated ceramic blocks could be affected of various treatments and could change over time.
1
Introduction
Ceramics have been an alternative for dental restorations for many years but their brittle nature has previously limited their application to the anterior region and to use as veneering material in ceramic-fused-to-metal restorations. The clinical properties of ceramics desirable in dentistry, e.g. esthetics, biocompatibility, chemical resistance and low plaque retention, have encouraged the development of ceramic materials with improved fracture resistance . Today modern manufacturing processes, such as strictly controlled prefabrication of homogenous ceramic blocks and computer-aided design/computer-aided manufacturing (CAD/CAM) techniques, make it possible to handle ceramic materials in a way that was impossible earlier . Stronger and tougher dental ceramic materials are nowadays available to be used as all-ceramic single-to-multiple units in anterior and posterior regions .
Two types of ceramics currently used in dentistry are glass-ceramics and zirconia oxide ceramics . Glass-ceramics consist mainly of silica-based glass matrix reinforced with crystals which prevents crack propagation and offers high esthetic quality regarding color and translucency. These have been used in dentistry since the 1950s . Among the advantages of glass-ceramics is that the silica content allows etching and adhesive cementation .
During the 1990s another type of glass-ceramic became available for dentistry, the lithium di-silicate glass-ceramic . This is a monolithic ceramic, which can be processed using the CAD/CAM technique in selectable colors and finished with painting and glazing. The indications are said to be inlays, onlays, single crowns and three-unit fixed partial dentures (FPDs) .
Zirconia oxides offer excellent mechanical properties and are considered among the most fracture resistant dental ceramic materials. Zirconia is mainly used as core material veneered with feldspathic or glass ceramics to improve the esthetics and reduce grinding on antagonists. The fracture resistance of zirconia depends mainly on the crystallographic form and transformation toughening properties. Pure zirconia can exist in three different phases; cubic (c), tetragonal (t) and monoclin (m) . The cubic phase occurs at a temperature of >2370 °C, the tetragonal phase between 1170 and 2370 °C and the monoclin phase between 0 and 1170 °C . During cooling an increased amount of the t → m phase occurs, which unless controlled can be catastrophic for the mechanical properties of the material . In order to control the phase transformation stabilizing oxides such as yttria, magnesia, hafnia or ceria can be added to zirconia. This is known as partially stabilized zirconia (PSZ) . The tetragonal phase will then remain at room temperature but transformation to monoclin phase can still occur, so-called low-temperature degradation (LTD) or aging, among other situations in occasion of stress, could stop crack propagation and thereby improve the fracture resistance of the material .
One type of zirconia used as biomaterial is magnesia-stabilized zirconia (Mg-PSZ), used for example in odontology and orthopedics . Mg-PSZ is one of the toughest zirconia-based ceramics and is usually sintered at ∼1800 °C. It has a grain size ∼30–60 μm . One interesting property of Mg-PSZ as a biomaterial is that it is said to be more resistant to LTD than yttria-partially stabilized zirconia (Y-TZP) .
Since it has been demonstrated that various pretreatments, such as veneering, heat treatment and sandblasting could affect the properties of zirconia ceramics and it has been stated that Mg-PSZ exhibits good resistance to LTD and aging it was of interest to study the effect on the strength of Mg-PSZ of various pretreatments used in the production of dental restorations. In addition, it has recently been stated that in studies on the effect of various pretreatments and aging on lithium di-silicate glass-ceramics various testing parameters have been used making direct comparisons between the studies difficult . The aim of the present work was, therefore, to study the effect of various pretreatments and hydrothermal aging on the fracture strength of lithium di-silicate and Mg-PSZ ceramics.
2
Materials and methods
2.1
Preparation of specimens
Using computer-aided machining (Cad.esthetics Softwear system, Cad.esthetichs AB, Skellefteå, Sweden) disc-shaped specimens were sliced from prefabricated blocks of a lithium di-silicate glass-ceramic (IPS e.max Cad, Ivoclar-Vivadent, Schaan, Lichtenstein. Batch No. N79116) and a magnesia-stabilized zirconia ceramic, Mg-PSZ (ZirMagnum, Cad.esthetichs AB. Batch No. SM-0936). The diameter of the discs was 13 mm. The thickness of the e.max Cad discs was 0.4 mm and 0.8 mm and of the ZirMagnum discs 0.25 mm, 0.4 mm and 1.3 mm. The specimens were ultrasonically (Bransonic B221; Bransonic Ultrasonic Co, Danbury, Conn., USA) cleaned for 10 min in tap water, rinsed in distilled water and air-dried. To study the effect of glazing and hydrofluoric acid (HF-acid) on the strength of the e.max Cad the biaxial flexural fracture strength was determined after the e.max Cad discs were: i ) crystallized, ii ) crystallized and glazed, iii ) crystallized, glazed and the unglazed side etched with hydrofluoric acid gel for 20 s (9.5% HF-acid, Ultradent Porcelain Etch, Ultradent Products Inc., South Jordan, Utah, USA. Batch no B3K28). Crystallization and glazing were carried out in accordance with the manufacturer’s instructions (Ivoclar-Vivadent). For glazing IPS e.max Cad Crystal/Glaze (Ivoclar-Vivadent. Lot No. N74262) was used. According to the manufacturer’s information the composition (weight%) of the glazing paste was SiO 2 60–65%, K 2 O 15–19%, Al 2 O 3 6–10.5% and other oxides, pigments 5.5–30%. Crystallization and glaze firing were performed in one step. The firing temperature was 840 °C.
To study the effect of glazing on the biaxial flexural fracture strength the e.max Cad specimens were loaded in different directions; one group were placed in the sample holder (The European Standard EN ISO 6872, 2008) with the glazed surface of the specimens facing down (in tension) bearing on the three stainless-steel supporting balls, whereas the other group was placed in the same position but with the unglazed surface facing down, i.e ., the glazed surface in compression and the unglazed surface in tension. The specimens etched with hydrofluoric acid were placed in the sample holder with the unglazed and etched surface in tension. To ensure that the thickness of the glazed layer of the e.max Cad specimens did not exceed 30 μm, and considering it to be a mono-layered construction , the thickness of the specimens was measured before and after glazing using a digital caliper (Solar Absolute Digmatic, Mitutoyo, Japan).
The biaxial flexural fracture strength of the ZirMagnum specimens was studied i ) as delivered, ii ) after sandblasting one of the flat surfaces of the discs and iii ) after a heat treatment similar to veneering. The sandblasting of one of the flat surfaces of the discs was done at a pressure of 0.2 MPa or 0.6 MPa using 110 μm Al 2 O 3 applied for 90 s at a distance of approximately 2 mm between the nozzle and the surface of the disc (Basic Quattro, Renfert GmbH, Hitzingen, Gemany). After sandblasting the ZirMagnum discs were ultrasonically (Bransonic B22) cleaned for 10 min in tap water, rinsed in distilled water and air-dried. During the biaxial flexural test the sandblasted specimens were placed in the sample holder with the sandblasted surface face down bearing on the three stainless-steel supporting balls, i.e. , the sandblasted surface in tension.
For both types of the ceramics, i.e . e.max Cad and ZirMagnum, all the groups examined in the present study comprised 10 specimens.
2.2
Hydrothermal aging
Ten of the 0.4 mm crystallized e.max Cad discs, 10 of the 0.8 mm crystallized glazed and HF-etched e.max Cad discs, 10 of the 0.4 mm as delivered ZirMagnum discs and 10 of the 0.6 MPa sandblasted and heat-treated ZirMagnum discs were hydrothermally aged at 134 °C and 0.2 MPa for 2 × 5 h (Autoklav Typ GE 446, Getinge AB, Halmstad, Sweden). Between the two autoclave treatments the specimens were stored at room temperature for 16 h.
2.3
Surface roughness
After the specimens were ultrasonically cleaned (Bransonic B221) for 5 min in tap water, rinsed in distilled water and air-dried the average surface roughness (Ra; μm) of the surface intended to be in tensile stress during the biaxial flexural testing was determined using a measuring profilometer (Taylor/Hobson Precision Form Taylor Surf 50, Taylor Hobson, IL, USA), stylus tip radius 2 μm, cut-off length 0.8 mm, evaluation length 4 mm and stylus speed 0.5 mm/s. These settings were in accordance with the EN 623-4:2004 standard . Ten parallel scans were made at 0.5 mm intervals resulting in 10 scans for each specimen, giving a total of 100 scans for each test group.
2.4
Biaxial flexural strength test
A universal testing machine (Tinius Olsen H10K-T, Horsham, PA, USA) and test set-up in accordance to the ISO standard 6872 were used to determine the load at facture (Newton) of the various ceramic discs. The support area for the discs consisted of three steel balls with a diameter of 3.2 mm each, positioned 120° distance from each other, creating a circle of Ø 10 mm (ISO Standard 6872, 2008) . The load was applied at the center of the specimen with a flat dowel pin with a diameter of 1.6 mm and the crosshead speed was 1 mm/min (ISO standard 6872, 2008) . After the specimens were loaded to fracture a microscope (Leitz UWM-Dig-S, Ernst Leitz GmbH, Wetzlar, Germany) was used to determine the thickness of the specimens. The microscope accuracy was 0.5 μm and the thickness was measured at 20× magnification at 3 selected measuring points; one in the center of the specimen and the other two 1 mm from the border of the specimen at the fractured surface. The mean of the 3 measurements was then used for ‘ d ’ in the formula (1) below.
Biaxial flexural strength was determined in accordance to the ISO Standard 6872 using the equations
S = − 0.2387 P ( X − Y ) d 2
where S is the maximum tensile stress (MPa), P is the load at fracture (N),
X = ( 1 + ν ) ln ( r 2 r 3 ) 2 + [ ( 1 − ν ) 2 ] ( r 2 r 3 ) 2
Y = ( 1 + ν ) [ 1 + ln ( r 1 r 3 ) 2 ] + ( 1 − ν ) ( r 1 r 3 ) 2
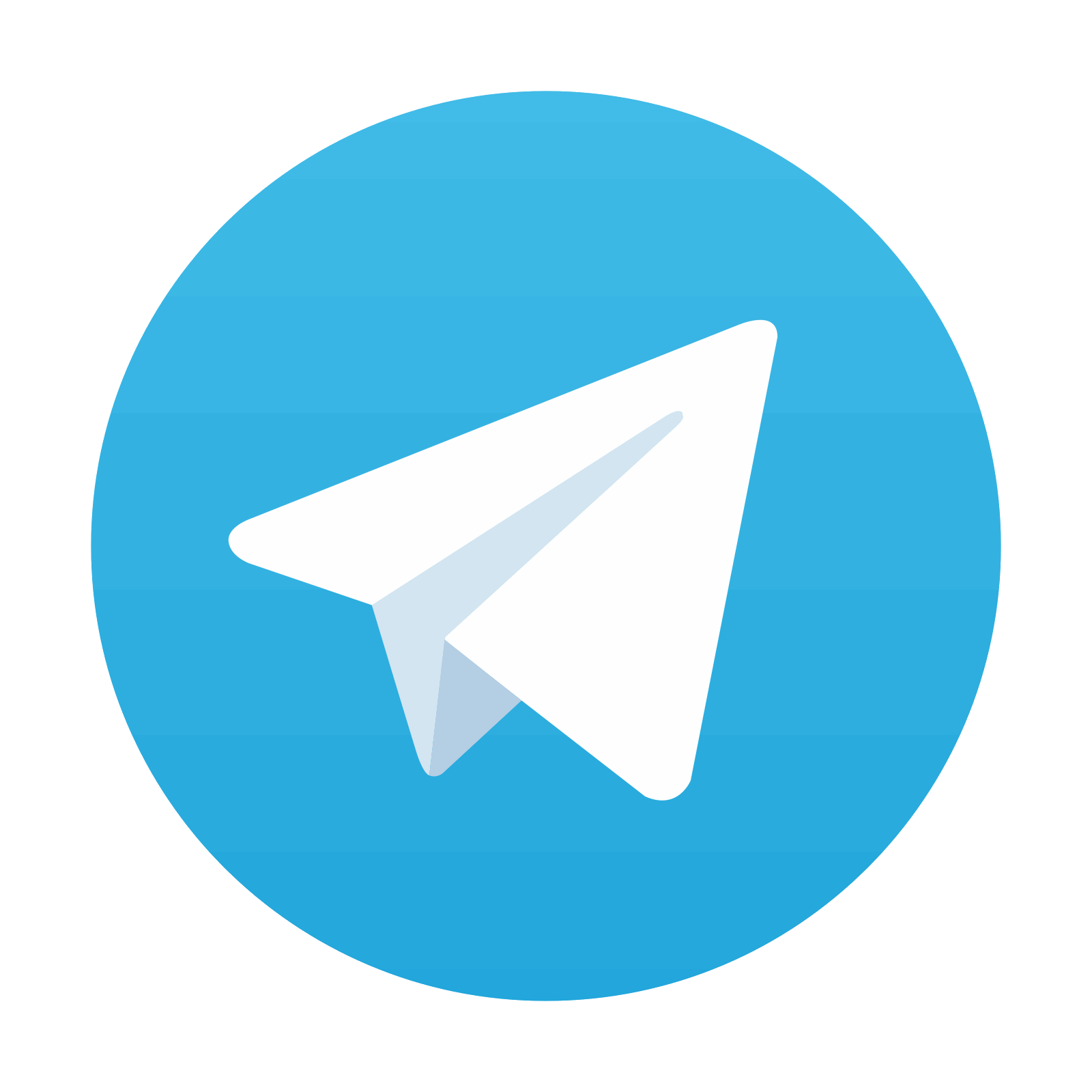
Stay updated, free dental videos. Join our Telegram channel

VIDEdental - Online dental courses
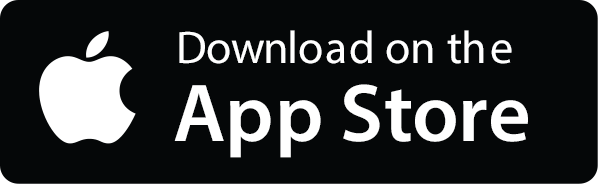
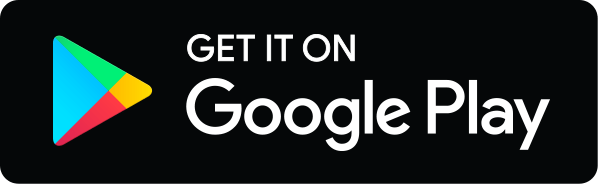