Introduction
The aims of this study were to determine the effects of different concentrations of platelet-rich plasma (PRP) on alveolar bone density and orthodontic tooth movement.
Methods
Seventy-six rats were divided into 2 groups: a moderate concentration PRP injection group (n = 38) and a high concentration PRP injection group (n = 38). In each group, 5 time points were studied: 3, 7, 14, 21, and 60 days. Before orthodontic mesialization of the maxillary first molars, moderate and high concentrations of PRP were injected on the right sides of the molar buccal sulcus, and the left sides served as the controls. Tooth movements were measured on 3-dimensional digital models. Alveolar bone volume density and osteoclastic activity in the first molar intraradicular areas were evaluated by histomorphometric analysis.
Results
Alveolar bone density was decreased in the experimental groups compared with the control groups ( P = 0.0001) at 3, 7, 14, and 21 days. On day 3, osteoclastic activity of the experimental groups was higher than that of the controls ( P = 0.044, P = 0.0001). On day 21, the amounts of tooth movement in the high-concentration experimental group were 1.7 times greater than in the high-concentration control group and 1.4 times greater than in the moderate-concentration experimental group ( P = 0.001). On day 60, alveolar bone density increased to the original levels in all groups.
Conclusions
Injection of both moderate and high concentrations of PRP may accelerate orthodontic tooth movement by decreasing alveolar bone density on paradental tissues by enhancing osteoclastic activity in a transient way.
Highlights
- •
We analyzed the effects of platelet-rich plasma (PRP) on orthodontic tooth movement.
- •
Faster tooth movement was seen after PRP injection.
- •
PRP accelerates orthodontic tooth movement in a dose-dependent way.
Platelet-rich plasma (PRP) is defined as an autologous concentration of platelets in a small volume of plasma and is considered to be a rich source of autologous growth factors (GFs). GFs are natural biologic mediators that regulate key cellular events that are part of the process of tissue repair and regeneration. After binding of GFs to specific cell membrane receptors of target cells, intracellular signaling pathways are induced; this typically results in the activation of genes that may ultimately change cellular activity and phenotype. However, the effect of each GF is regulated through a complex system of feedback loops, which involve other GFs, enzymes, and binding proteins. Recent advances in the areas of cellular and molecular biology have allowed better understanding of the functions of GFs. In-vitro and in-vivo studies have confirmed that GFs can enhance the capacity of tissues to regenerate by regulating cell chemo-attraction, differentiation, and proliferation. PRP was first introduced to the dental literature in 1998 in combination with autogenous bone grafts for the reconstruction of mandibular defects, reporting that the addition of PRP to bone grafts resulted in a faster radiographic maturation rate and a higher bone density than bone grafts alone. However, controversies exist regarding the potential benefits of this procedure. Although some found significant increases in bone formation and maturation rates, others did not observe any affect. Interestingly, some other authors reported that PRP can play an inhibitory role in bone metabolism in a concentration-dependent fashion. Choi et al examined the influence of PRP concentrations on the viability and proliferation of alveolar bone cells in vitro, and their results showed that the viability and proliferation of alveolar bone cells were suppressed by high PRP concentrations and stimulated by low PRP concentrations. In a more recent study, Peerbooms et al investigated whether the use of PRP mixed with bone chips improves bone healing in patients with skeletal defects using computed tomography scanning and reported that at 12 weeks the PRP group had a significantly lower bone density under the wedge compared with the control group.
The process of bone remodeling usually involves resorption of old bone by osteoclasts and formation of new bone by osteoblasts. The amount of skeletal tissue depends on equilibrium between the rates of new bone formation and old bone resorption during physiologic growth and skeletal remodeling. Mechanical loads, in particular orthodontic forces, can also affect the bone remodeling process. The turnover rate of alveolar bone determines the quality and quantity of orthodontic tooth movement. To move teeth faster and to shorten the orthodontic treatment duration, the balance between resorption and apposition needs to be modified. Any increase in osteoclastic activity results in the downturn of alveolar bone density at the region of tooth movement. Studies have reported that any alterations in bone density around the relevant tooth during orthodontic tooth movement can result in the acceleration of tooth movement.
Based on the findings of cell culture studies, histomorphometric studies, and computed tomography studies that reported an inhibition effect of PRP on bone cell division and a decrease in bone density in high-concentration applications, it can be hypothesized that PRP application might be effective on the rate of orthodontic tooth movement.
Therefore, the purpose of this study was to investigate whether different concentrations of PRP administration can accelerate orthodontic tooth movement by causing a decrease in bone density next to the periodontal area.
Material and methods
This study was approved by the Institutional Animal Care and Use Committee (protocol approval number 03.10.2013-89) of the University of Marmara, Istanbul, Turkey. A total of 81 young adult Sprague-Dawley rats (348 ± 29 g, 9-10 weeks old) were obtained and acclimatized in the University Laboratory Animal Science Centre. The animals were divided into the following groups: donor group (killed for the preparation of PRP; n = 5), high-platelet concentration injection group (hPRP, n = 38), and moderate platelet concentration injection group (mPRP, n = 38). In the latter 2 groups, 5 time points were studied on days 3, 7, 14, 21, and 60. Both the hPRP and mPRP injection groups consisted of 8 animals per time point, except for the day-60 groups, which had 6 animals each. The animals were fed a soft rat diet to prevent any damage to the intraoral appliance and water ad libitum. To limit the influence of interanimal variation in response to the PRP injection, a split-mouth design was used, and the right side of each animal’s maxilla served as the experimental side (hPRP-E and mPRP-E), whereas the left side served as the control side (hPRP-C and mPRP-C) without receiving any PRP injection. All procedures were completed under general anesthesia with intraperitoneal administration of a combination of ketamine (100 mg/kg) and xylazine (10 mg/kg).
Orthodontic tooth movements were achieved with superelastic closed-coil springs (9 mm; G&H Wire Company, Franklin, Ind) placed between the maxillary molars and incisors as previously described. The superelasticity of the coil springs ensured a constant force of 40 cN, and no reactivation was performed during the experimental period. The closed-coil springs were attached to the maxillary first molars and incisors with a stainless steel ligature wire (0.008 in; G&H Wire Company). To improve the fixation of the appliance, a 0.5-mm hole was made by using a metal bur with a slow-speed hand piece. The hole was drilled from the distal surfaces of the incisors, through the teeth, perpendicular to the longitudinal axis of the incisor at the gingival level. A steel wire was inserted through the hole and then tied. The free ends of the ligatures on both molars and incisors were covered with glass ionomer cement (Multi-Cure Glass Ionomer Orthodontic Band Cement; 3M Unitek, Monrovia, Calif) to prevent slipping and gingival irritation. The mandibular incisors were trimmed weekly with a coarse diamond bur to ensure that they were out of occlusion and not at risk of breaking the appliance.
The production of PRP began with a 10-mL homologous blood sample that was withdrawn from the donor animal via cardiac puncture. One milliliter of the blood sample was set apart to determine the concentration of platelets and leukocytes in whole blood. The remaining 9 mL was mixed with an anticoagulant (3.8%, 1-mL sodium citrate) to prevent clotting. The blood sample was centrifuged at 113 g for 5 minutes to separate the plasma containing the platelets from the red cells. The plasma was drawn off the top and centrifuged for an additional 2 minutes at 3772 g to separate the platelets. Then, suitable platelet concentrations were achieved by the resuspension of PRP with plasma (high platelet concentration, 5 times the concentration in whole blood; moderate concentration, 2 times the concentration in whole blood).
The concentrations of platelets and leucocytes in whole blood and the PRP were analyzed automatically (Genius KT Veterinary, Shenzhen Genius Electronics, Guangdong, China) using modified software for animal probes. Triple measurements were performed, and the mean value was recorded as the final measurement.
After each animal’s appliance was placed in a certain subgroup (example: 3-day hPRP-E, n = 8; 3-day mPRP-E, n = 8), a donor rat was killed for the preparation of fresh PRP. The concentrations were measured, and 10 μL of PRP was injected by means of a microsyringe (Hamilton Robotics, Bonaduz, Switzerland) into the buccal vestibular mucosa next to the mesial root of the right first molar of each rat while it was under general anesthesia. All injections were volumetrically equivalent, and the determined volume limit of 10 μL was the limit that the tissue could accept without repulsion. Injections were done only once at day 0 and not repeated again. The remaining PRP samples from both the hPRP-E and mPRP-E groups were stored at −4°C for future biochemical analysis to determine the level of platelet-derived growth factor- ββ (PDGF ββ ) at both high and moderate PRP concentrations.
Tooth movement distances were measured by palatal superimposition of 3-dimensional (3D) digital models as previously described. Silicone (Express XT Quick; 3M ESPE, Seefeld, Germany) impressions of the rats’ maxillary dentitions at baseline and at every observational time point before they were killed were directly scanned with a 3D scanner (3 Shape R 700; CadBlu Dental, Chicago, Ill). The validity and precision of the scanner was 0.05 mm. Reconstructions of 3D models were performed by 3 Shape Scanniorthodontics 2008 (CadBlu Dental), and the pretreatment and postmesialization models were superimposed on the area of the palatal rugae. After superimposition, the amount of tooth movement was calculated by using Mimics software (version 12.11; Materialise, Leuven, Belgium). The midpoints of the mesial marginal ridge of the maxillary right and left first molars were marked on the pretreatment and posttreatment models. The distances between these points were measured. All measurements were repeated 3 times by the same investigator (A.G.) blinded to the treatment groups.
PRP samples stored at −4°C were brought to room temperature, and to ascertain the concentration of PDGF ββ , the hPRP and mPRP samples were assayed in a rat PDGF ββ immunoassay (Cusabio Biotech, Wuhan, China) as described by the manufacturer. The minimum detectable dose was 31.25 pg per milliliter. Measurements for each sample were made in duplicate. In brief, the microplate provided in the kit was precoated with an antibody specific to PDGF ββ . Standards and samples were added to each well for 2 hours of incubation at 37°C. A biotin-conjugated antibody specific for PDGF ββ added to the well and incubated again at 37°C for 1 hour. After they were washed 3 times, the wells received avidin conjugated to horseradish peroxidase solution and incubated for 1 hour at 37°C. After they were washed 5 times with the wash solution, the TMB (3, 3′, 5, 5′-Tetramethylbenzidine) substrate solution was added to each well, and the wells were incubated for 15 to 30 minutes at 37°C in the dark. The reaction was stopped by adding stop solution, and the intensity of the color was measured spectrophotometrically at 450 nm using a microplate reader (RT-2100C; Rayto Life and Analytical Sciences, Shenzhen, China). The data were analyzed via 4-parameter logistic curve fitting using CurveExpert Basic (version 1.40; CurveExpert and GraphExpert Software, Madison, Ala.).
Eight animals from each group were killed on days 3, 7, 14, and 21, and 6 animals from each group were killed on day 60. After that, the maxillae were removed, stripped of soft tissues, and prepared for histology. Tissue samples were fixed in 10% neutral formaldehyde in 0.1 mol of phosphate buffered saline solution (pH = 7.4). Samples were decalcified with Morse solution (10% sodium citrate and 22.5 % formic acid) for 4 to 5 weeks, dehydrated in an alcohol series, divided in 2 hemimaxillar halves, and embedded in paraffin. Paraffin blocks were sectioned longitudinally (10 μm thick) parallel to the long axis of each tooth, from mesial to distal aspects. As a second step, every tenth tissue sample section was selected through a set of consecutive sections. Choosing the first section was done in a systematic random manner. Sampled sections were stained with Masson’s trichrome techniques.
A semiautomated stereology workstation for image and stereologic analyses composed of a CCD digital camera (Microfire 1600 × 1200P; Optronics, Goleta, Calif), image capture card (ATI FireGL; Advanced Micro Devices, Camberley, United Kingdom), personal computer, and computer-controlled motorized specimen stage (BioPrecision Group, Howthorne, New York), a microcator (Heidenhain, Traunreut, Germany), and a light microscope (DM 4000B; Leica, Wetzlar, Germany) were used. As software, Stereo Investigator (version 7.0; MicroBrightField, Williston, Vt) was used. The measurements were made using a C Plan X10 objective (Leica) (NA = 0.22).
The Cavalieri principle was used to estimate the volume of the alveolar bone using the previously described stereologic workstation. A point counting grid (d = 100 μm) was applied for volume estimation to obtain maximum efficiency. The representative areas per point (a/p) were 1000 μm 2 for each point. After applying the point counting grid to the sampled sections in a systematic random fashion, the numbers of points hitting alveolar bone in the sample were counted. Results were used for the estimation of alveolar bone volumes using the following formula:
volume alveolar bone = t ( μm ) × a/p ( μm 2 ) × Σ p
where, t is the mean section thickness; a/p represents the area of each point on the point counting grid; and Σ p is the total number of points hitting the sectioned area. Then, the total volume of sampled sections from each rat hemimaxilla was multiplied by the section sampling fraction to estimate alveolar bone volume.
The efficiency of sampling and convenient point density for volume estimation were checked by estimation of coefficient of error and coefficient of variation as previously described ( Tables I and II ).
hPRP | Day 3 hPRP-E/hPRP-C | Day 7 hPRP-E/hPRP-C | Day 14 hPRP-E/hPRP-C | Day 21 hPRP-E/hPRP-C | Day 60 hPRP-E/hPRP-C | |||||
---|---|---|---|---|---|---|---|---|---|---|
n = 6 | n = 6 | n = 6 | n = 6 | n = 6 | ||||||
Section thickness (μm) | 10 | 10 | 10 | 10 | 10 | |||||
Number of sampled sections | 13 | 13 | 15 | 15 | 14 | 14 | 13 | 12 | 14 | 14 |
Mean intraradicular alveolar bone point number | 2153 | 2279 | 1860 | 2461 | 1622 | 1976 | 1050 | 1481 | 1954 | 1989 |
CE intraradicular alveolar bone | 0.008 | 0.007 | 0.005 | 0.006 | 0.008 | 0.006 | 0.006 | 0.007 | 0.008 | 0.006 |
Mean intraradicular total point number | 4376 | 5067 | 4621 | 5111 | 5179 | 5196 | 4491 | 4851 | 4858 | 4868 |
CE intraradicular total region | 0.007 | 0.006 | 0.009 | 0.007 | 0.008 | 0.005 | 0.007 | 0.007 | 0.008 | 0.008 |
mPRP | Day 3 mPRP-E/mPRP-C | Day 7 mPRP-E/mPRP-C | Day 14 mPRP-E/mPRP-C | Day 21 mPRP-E/mPRP-C | Day 60 mPRP-E/mPRP-C | |||||
---|---|---|---|---|---|---|---|---|---|---|
n = 6 | n = 6 | n = 6 | n = 6 | n = 6 | ||||||
Section thickness (μm) | 10 | 10 | 10 | 10 | 10 | |||||
Number of sampled sections | 13 | 14 | 15 | 15 | 14 | 13 | 13 | 13 | 14 | 13 |
Mean intraradicular alveolar bone point number | 2047 | 2117 | 1932 | 2004 | 1491 | 1668 | 1192 | 1169 | 2034 | 2061 |
CE intraradicular alveolar bone | 0.007 | 0.005 | 0.005 | 0.007 | 0.008 | 0.007 | 0.005 | 0.008 | 0.008 | 0.006 |
Mean intraradicular total point number | 4376 | 4603 | 4621 | 4713 | 5179 | 4374 | 4491 | 4343 | 4868 | 4744 |
CE intraradicular total region | 0.008 | 0.005 | 0.007 | 0.008 | 0.007 | 0.005 | 0.005 | 0.008 | 0.007 | 0.006 |
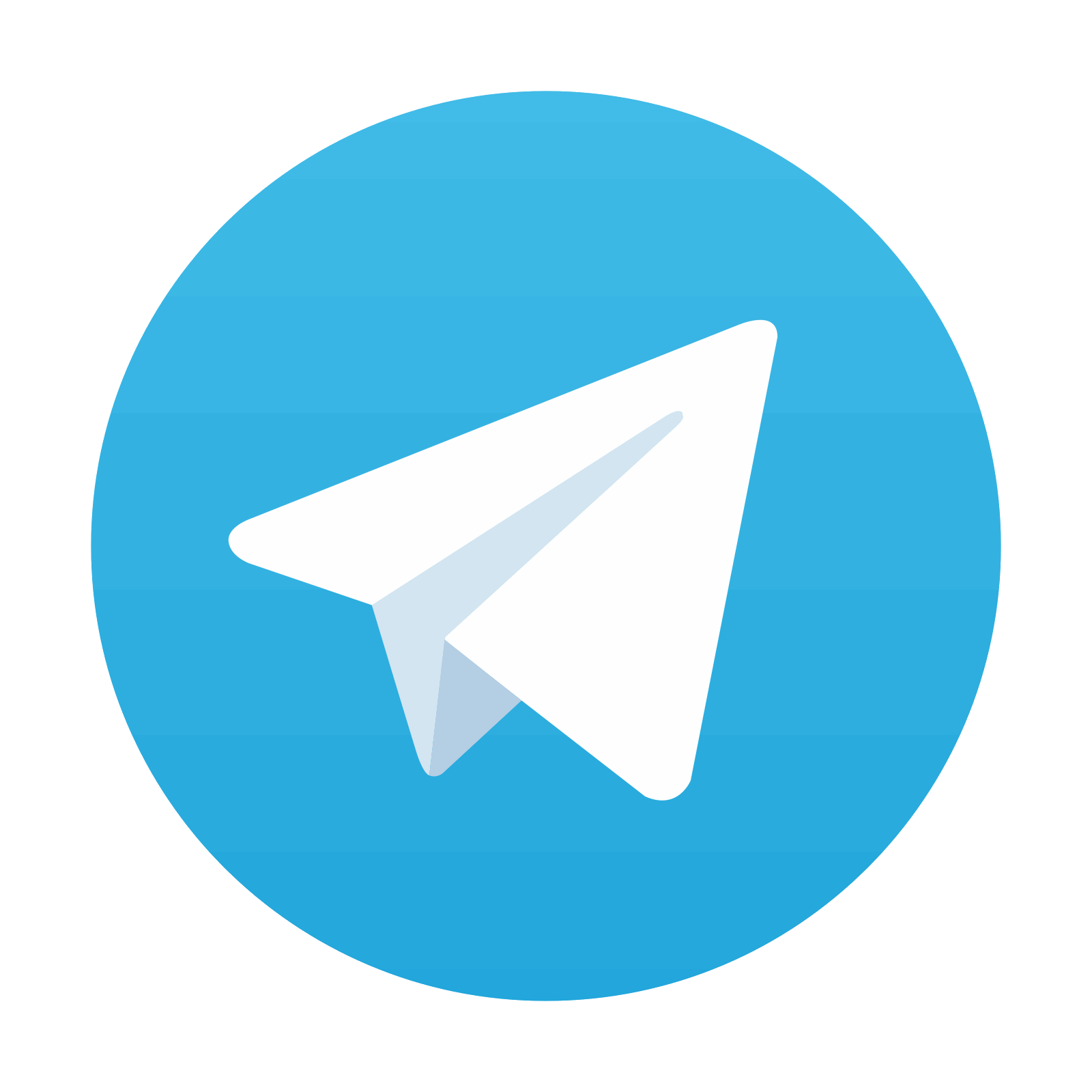
Stay updated, free dental videos. Join our Telegram channel

VIDEdental - Online dental courses
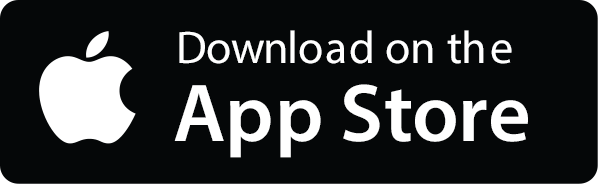
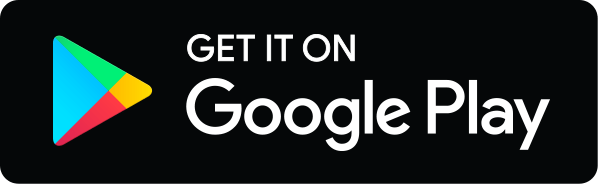
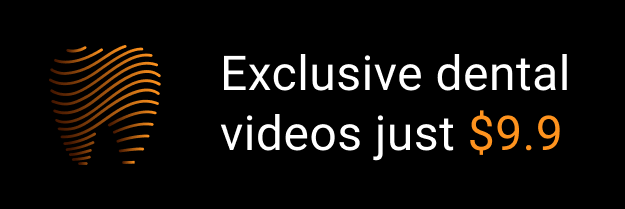