Abstract
The effect of preparation design and the physical properties of the interface lute on the restored machined ceramic crown–tooth complex are poorly understood. The aim of this work was to determine, by means of three-dimensional finite element analysis (3D FEA) the effect of the tooth preparation design and the elastic modulus of the cement on the stress state of the cemented machined ceramic crown–tooth complex.
The three-dimensional structure of human premolar teeth, restored with adhesively cemented machined ceramic crowns, was digitized with a micro-CT scanner. An accurate, high resolution, digital replica model of a restored tooth was created. Two preparation designs, with different occlusal morphologies, were modeled with cements of 3 different elastic moduli. Interactive medical image processing software (mimics and professional CAD modeling software) was used to create sophisticated digital models that included the supporting structures; periodontal ligament and alveolar bone. The generated models were imported into an FEA software program (hypermesh version 10.0, Altair Engineering Inc.) with all degrees of freedom constrained at the outer surface of the supporting cortical bone of the crown–tooth complex. Five different elastic moduli values were given to the adhesive cement interface 1.8 GPa, 4 GPa, 8 GPa, 18.3 GPa and 40 GPa; the four lower values are representative of currently used cementing lutes and 40 GPa is set as an extreme high value. The stress distribution under simulated applied loads was determined. The preparation design demonstrated an effect on the stress state of the restored tooth system. The cement elastic modulus affected the stress state in the cement and dentin structures but not in the crown, the pulp, the periodontal ligament or the cancellous and cortical bone. The results of this study suggest that both the choice of the preparation design and the cement elastic modulus can affect the stress state within the restored crown–tooth complex.
1
Introduction
The restored tooth is a complex compound structure that combines materials and the biological structures of the tooth and its supporting structures to restore function and esthetics. The materials used and the manner in which they are integrated and joined to each other and the enamel and dentin determines the ability of the restored tooth to withstand the stress caused by the forces of mastication. The nature of the load and the magnitude and distribution of these stresses determines the structural integrity of the tooth and its durability. The distribution of these internal stresses depends on the shape of the body and the stiffness distribution within it .
The analysis of stress and strain of any structure provides good predictors of the mechanical behavior of an object subjected to a load. Such information might be useful to manufacturers and clinicians in the design and provision of optimized restorative materials and systems.
Various techniques have been used to evaluate the stress and strain state in dental structures, including transmission and reflection microscopy, two- and three-dimensional photoelasticity and strain gauge techniques . The tooth-restoration unit possesses a complex geometry and variable stiffness values, thus the traditional stress analysis methods are not able to provide accurate results since these mathematical theories can be applied only to certain regular shapes with homogenous structure . Stress and strain analysis of complex geometries with a heterogeneous structure, such as the tooth-restoration assembly, can be undertaken using FE analysis methods applicable to solids of irregular geometries and heterogeneous properties . With this technique, the various components of a system are digitally modeled and subdivided into simpler geometric shapes or elements and the deformation (strain and stress) can be easily calculated for each component . According to the simultaneously calculated deformation for each element, the deformation of the whole structure can be assessed . Finite element analysis methods enable the study of the biomechanical behavior of the restored system under different loading conditions and the manipulation of individual/specific components of the system by specifying each element’s physical properties, such as the elastic modulus . It is suggested that the results from FE analysis may help predict the clinical behavior of restorations more reliably .
Within the compound structure of the restored tooth–crown system, the dimensions and physical properties of the adhesive lute interface is a key determinant of its mechanical behavior. The two-dimensional FE method has been used by different authors to evaluate the effect of the interface lute parameters, elastic modulus and thicknesses of the luting agent, under all-ceramic crowns .
In addition, the shape of the underlying preparation may also be responsible for the stress state in the ceramic crown–tooth complex. The traditional preparation designs for hand-made, sintered porcelain crowns is that the occlusal surface should follow the anatomical shape of the tooth with a minimal occlusal thickness of 2 mm . The advent of stronger ceramics that are adhesively bonded to the underlying tooth may enable the preparation of teeth with more conservative designs. Our understanding of the effect of the adhesive interface and the preparation design on the mechanical behavior of teeth restored with contemporary all-ceramic crowns is poor.
A successful computational model of any structure in FE analysis requires an accurate geometry of the object . Two-dimensional or three-dimensional approaches have been used for modeling purposes . Two-dimensional models are criticized as they result in oversimplified geometries of complex structures that in turn may compromise the reliability of predicting the mechanical behavior of the object .
The aim of this study is to investigate the effect of the modulus of elasticity of the cement interface and the preparation design for all-ceramic crown preparations on the restored ceramic crown–tooth complex. This is assessed by means of finite element analysis of a digital model created from a real tooth–ceramic crown system with the aid of micro-CT scanning and computational modeling.
2
Materials and methods
2.1
Preparation of the physical model
Two matching human maxillary first premolars extracted for orthodontic reasons, from the same patient, were selected with appropriate ethical approval obtained. All-ceramic crown preparations were carried out with a 1 mm rounded shoulder finish line and 12 degrees of taper. One tooth had a traditional anatomic occlusal preparation design, with an overall 2 mm occlusal reduction that reflected the occlusal morphology of the inclined cusp surfaces (anatomic design). The other tooth had a flat design with a 1.2 mm flat occlusal reduction, measured from the central occlusal fissure (flat design) ( Table 1 ). The axial element of the preparations were standardized using a paralleling device and gauged burs.
Area of reduction | Anatomic occlusal reduction (Group #1) | Flat occlusal reduction (Group #2) |
---|---|---|
Occlusal | 2 mm from the occlusal center, following the cuspal profile | 1.2 mm from the occlusal center, flat reduction across the cusp tips |
Axial reduction | 1.2–1.5 mm | 1.2–1.5 mm |
Taper | 12° | 12° |
The crowns were machined from CEREC Vita Mark-II blocks (shade A 2, VITA Mark II, 2M2C I12, 12 mm long, Vita Zahnfabrik, Bad Sackingen, Germany). The outer shape of the ceramic crown was a replica of the unprepared crown of the natural tooth to be restored. This was achieved by using the “correlation” mode of the CEREC 3 CAD/CAM (CEREC inLab software version 6.30) program. Briefly, the unprepared teeth were powdered with an optical scanning powder (CEREC powder VITA Zahnfabric, Germany) before tooth preparation. An optical impression of the tooth was then taken with the Eos laser scanner (SIRONA Dental Systems, GmbH). The ceramic crown was designed and milled using VITA Mark II blocks. The milled restoration was cemented on the prepared teeth using Rely X Unicem Clicker (3M ESPE) cement following the manufacturer’s instructions.
2.2
Preparation of the finite element model
A high resolution micro-CT scanner (Sky scan 1072 micro-CT; SkyScan, Aartselaar, Belgium) was used to scan the teeth restored with the cemented all-ceramic machined restorations. A resolution of 12.5 μm (distance between slices) was selected to obtain a high accuracy image.
After micro-CT scanning, the data acquired were reconstructed using Nrecon Reconstruction software (Sky scan 1072 micro-CT; SkyScan, Aartselaar, Belgium). The reconstruction was conducted after exclusion of any possible errors in the sample scanning procedure, including possible movements that may have resulted in a blurred image. The sharpness of the images taken was assessed by systematically selecting different areas of the tooth and using the “preview option” to analyse these. The output format of the acquired data were selected to be transferred as a bitmap (bmp) file to ensure compatibility with the processing software. Interactive medical image processing software; Mimics 13.1 (Materialize Co. Ltd.) was used to reconstruct the three-dimensional images allowing precise calculations from various directions.
An accurate three-dimensional model of the restored tooth system was created with a combination of Mimics software (Materialize Co. Ltd.) and a phantom haptic device and freeform software (SensAble Technologies Inc.). The different components of the tooth–crown complex were identified and separated using a segmentation procedure as Standard Triangle Language (STL) files with Mimics software. Modeling of the periodontal ligament (PDL) and bone structures was carried out using the haptic technology. The STL files are objects composed of small triangles (mesh) in a shell format. STL files are not recognized by FE software programs and hence are not directly transferable to enable the creation of an FE model. This problem was overcome by importing the files into a link program, ICEM software (version 11, ANSYS Inc.), that modifies the STL files so that they can be read by FEA programs. All the error elements, defects, and unnecessary projections were defined and refined using ICEM software. The correction procedure was carried out for each individual component and all the resultant data were exported in STL format.
In order to analyse the stress state with FEA modeling, the shell type was converted to the volume mesh using Hypermesh software (Version 10.0, Altair Engineering Inc.). The end result was a series of highly refined and accurate volume mesh constructs replicating the natural crown–tooth complex.
2.3
Material properties
Five different values for the elastic modulus of resin cements were selected to test their effect on the stress distribution throughout the different layers of the restored tooth–crown compound structure: 1.8 GPa, 4 GPa, 8 GPa, 18.3 GPa and 40 GPa. The value of 1.8 GPa was selected as matching that of the lowest value of a commercially available material (Super bond C&B) . The value of 40 GPa was selected as a hypothetical, extreme high, elastic modulus. The middle three values were selected as being intermediate and matching that of resin luting materials currently available on the market ( Table 2 ).
Cement material | Elastic modulus (GPa) |
---|---|
Super bond C&B, self-cure dental adhesive resin cement | 1.8 |
Rely X Luting plus resin-modified glass-ionomer cement | 4 |
Rely X Unicem, dual-polymerized self-adhesive universal resin cement | 8 |
Panavia F 2.0, dual-polymerized phosphate-modified resin cement | 18.3 |
Hypothetical, extreme high value material | 40 |
All the material’s properties were assumed to be isotropic, homogenous and linear elastic. The interface between layers was assumed rigid. The elastic modulus and Poisson’s ratio for each material has been selected in accordance with the existing literature ( Table 3 ).
Material type | Modulus of elasticity (MPa) | Poisson’s ratio | Reference |
---|---|---|---|
Crown | 69,000 | 0.25 | |
Cement | 1800 | 0.25 | |
4000 | 0.35 | ||
8000 | 0.33 | ||
18,300 | 0.30 | ||
40,000 | 0.35 | ||
Dentin | 18,600 | 0.31 | |
Pulp | 2 | 0.45 | |
Periodontal ligament | 50 | 0.45 | |
Trabecular bone | 13,700 | 0.30 | |
Spongy bone | 1370 | 0.30 |
2.4
Interface condition
The current study simulated a perfect bonding (rigid) condition with no slip interface conditions between the components (ceramic crown, resin cement layer, dentin structure, PDL, cancellous and cortical bones).
2.5
Boundary condition and load application
Following the creation of the 3D meshes, a boundary condition was applied to simulate the natural relationship of the tooth supported by the PDL and the bone structure. Thus a boundary condition (zero displacement) for the analysis purposes was defined at all nodes of the cortical bone that were constrained in all directions ( X , Y , and Z ).
To analyse the stress state, the load was applied to the occlusal surface of the crown. For this investigation, a two point (collection of nodes) occlusal load was applied on the palatal incline of the buccal cusp and the buccal incline of the palatal cusp. A load of 150 N was applied to the group of nodes of each of the two points, which collectively would be 300 N ( Fig. 1 ). A fair comparison was assured by analysing each model with exactly the same boundary condition and load application.
A convergence test, which is an effective way to identify the balancing accuracy of the FE result and computing resources, was applied in this study. In the convergence test method, meshes with successively smaller element numbers are used until it has been demonstrated that further refinement of the model does not alter stress values calculated at each layer of the model. The number of elements increased by 10% and a convergence analysis was carried out. The resulting data were compared in order to find out, the most beneficial element size in each structure.
2
Materials and methods
2.1
Preparation of the physical model
Two matching human maxillary first premolars extracted for orthodontic reasons, from the same patient, were selected with appropriate ethical approval obtained. All-ceramic crown preparations were carried out with a 1 mm rounded shoulder finish line and 12 degrees of taper. One tooth had a traditional anatomic occlusal preparation design, with an overall 2 mm occlusal reduction that reflected the occlusal morphology of the inclined cusp surfaces (anatomic design). The other tooth had a flat design with a 1.2 mm flat occlusal reduction, measured from the central occlusal fissure (flat design) ( Table 1 ). The axial element of the preparations were standardized using a paralleling device and gauged burs.
Area of reduction | Anatomic occlusal reduction (Group #1) | Flat occlusal reduction (Group #2) |
---|---|---|
Occlusal | 2 mm from the occlusal center, following the cuspal profile | 1.2 mm from the occlusal center, flat reduction across the cusp tips |
Axial reduction | 1.2–1.5 mm | 1.2–1.5 mm |
Taper | 12° | 12° |
The crowns were machined from CEREC Vita Mark-II blocks (shade A 2, VITA Mark II, 2M2C I12, 12 mm long, Vita Zahnfabrik, Bad Sackingen, Germany). The outer shape of the ceramic crown was a replica of the unprepared crown of the natural tooth to be restored. This was achieved by using the “correlation” mode of the CEREC 3 CAD/CAM (CEREC inLab software version 6.30) program. Briefly, the unprepared teeth were powdered with an optical scanning powder (CEREC powder VITA Zahnfabric, Germany) before tooth preparation. An optical impression of the tooth was then taken with the Eos laser scanner (SIRONA Dental Systems, GmbH). The ceramic crown was designed and milled using VITA Mark II blocks. The milled restoration was cemented on the prepared teeth using Rely X Unicem Clicker (3M ESPE) cement following the manufacturer’s instructions.
2.2
Preparation of the finite element model
A high resolution micro-CT scanner (Sky scan 1072 micro-CT; SkyScan, Aartselaar, Belgium) was used to scan the teeth restored with the cemented all-ceramic machined restorations. A resolution of 12.5 μm (distance between slices) was selected to obtain a high accuracy image.
After micro-CT scanning, the data acquired were reconstructed using Nrecon Reconstruction software (Sky scan 1072 micro-CT; SkyScan, Aartselaar, Belgium). The reconstruction was conducted after exclusion of any possible errors in the sample scanning procedure, including possible movements that may have resulted in a blurred image. The sharpness of the images taken was assessed by systematically selecting different areas of the tooth and using the “preview option” to analyse these. The output format of the acquired data were selected to be transferred as a bitmap (bmp) file to ensure compatibility with the processing software. Interactive medical image processing software; Mimics 13.1 (Materialize Co. Ltd.) was used to reconstruct the three-dimensional images allowing precise calculations from various directions.
An accurate three-dimensional model of the restored tooth system was created with a combination of Mimics software (Materialize Co. Ltd.) and a phantom haptic device and freeform software (SensAble Technologies Inc.). The different components of the tooth–crown complex were identified and separated using a segmentation procedure as Standard Triangle Language (STL) files with Mimics software. Modeling of the periodontal ligament (PDL) and bone structures was carried out using the haptic technology. The STL files are objects composed of small triangles (mesh) in a shell format. STL files are not recognized by FE software programs and hence are not directly transferable to enable the creation of an FE model. This problem was overcome by importing the files into a link program, ICEM software (version 11, ANSYS Inc.), that modifies the STL files so that they can be read by FEA programs. All the error elements, defects, and unnecessary projections were defined and refined using ICEM software. The correction procedure was carried out for each individual component and all the resultant data were exported in STL format.
In order to analyse the stress state with FEA modeling, the shell type was converted to the volume mesh using Hypermesh software (Version 10.0, Altair Engineering Inc.). The end result was a series of highly refined and accurate volume mesh constructs replicating the natural crown–tooth complex.
2.3
Material properties
Five different values for the elastic modulus of resin cements were selected to test their effect on the stress distribution throughout the different layers of the restored tooth–crown compound structure: 1.8 GPa, 4 GPa, 8 GPa, 18.3 GPa and 40 GPa. The value of 1.8 GPa was selected as matching that of the lowest value of a commercially available material (Super bond C&B) . The value of 40 GPa was selected as a hypothetical, extreme high, elastic modulus. The middle three values were selected as being intermediate and matching that of resin luting materials currently available on the market ( Table 2 ).
Cement material | Elastic modulus (GPa) |
---|---|
Super bond C&B, self-cure dental adhesive resin cement | 1.8 |
Rely X Luting plus resin-modified glass-ionomer cement | 4 |
Rely X Unicem, dual-polymerized self-adhesive universal resin cement | 8 |
Panavia F 2.0, dual-polymerized phosphate-modified resin cement | 18.3 |
Hypothetical, extreme high value material | 40 |
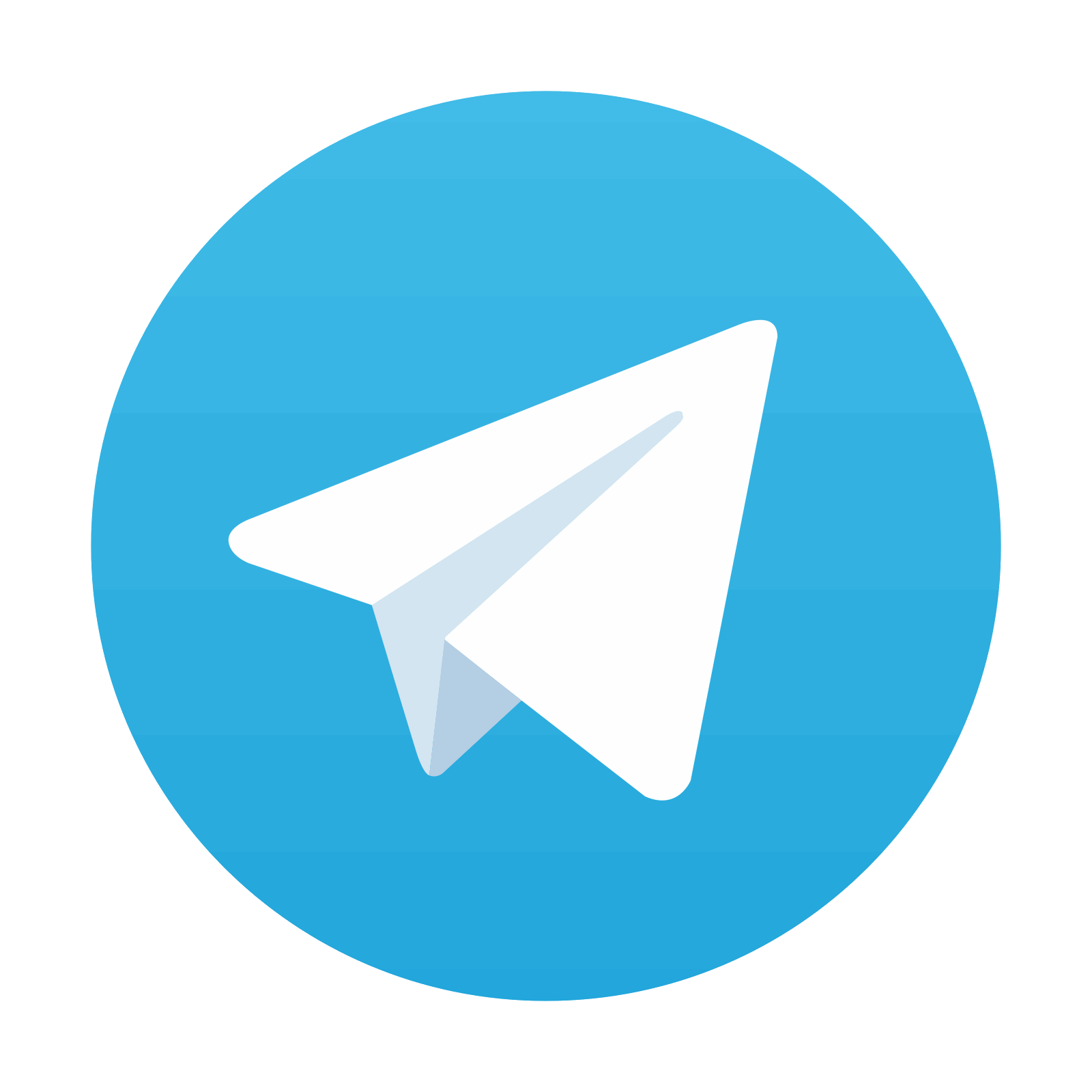
Stay updated, free dental videos. Join our Telegram channel

VIDEdental - Online dental courses
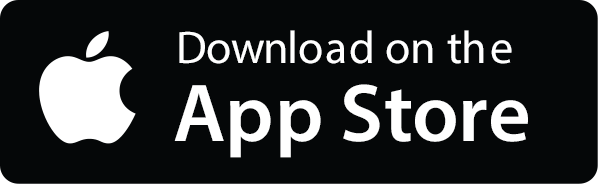
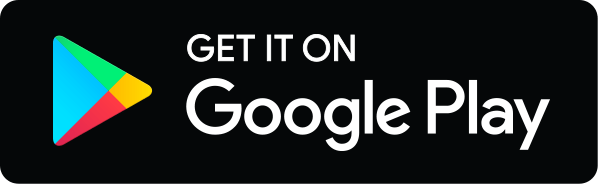
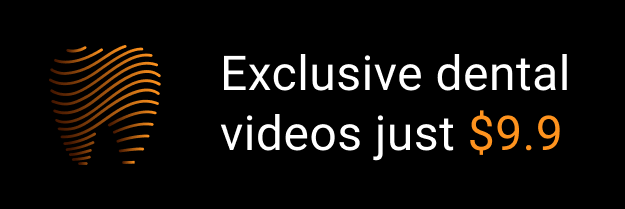