Abstract
Objectives
To evaluate the effects of airborne-particle abrasion (APA) and Er,Cr:YSGG laser irradiation on 4-point-flexural strength, phase transformation and morphologic changes of zirconia ceramics treated at pre-sintered or post-sintered stage.
Methods
Three hundred and forty-two bar shaped zirconia specimens were milled with different sizes according to the flexural strength test (n = 10), X-ray diffraction (XRD) (n = 4) and field emission scanning electron microscope (FE-SEM) (n = 4) analyses. For each test protocol, specimens were divided into 4 main groups whether the surface treatments applied before or after sintering and whether the specimens received heat treatment or not as pre-sintered, post-sintered no-heat and post-sintered heat-treated groups, and a group was served as control. Main groups were further divided into 6 equal subgroups according to surface treatment method applied (2 W-, 3 W-, 4 W-, 5 W-, 6 W-laser irradiations and APA). Surface treatments were applied to pre-sintered groups before sintering and to post-sintered groups after sintering. Post-sintered heat-treated groups were subjected to veneer ceramic firing simulation after surface treatments. Flexural strength and flexural modulus values were statistically analysed and monoclinic phase content was calculated. Weibull analysis was used to evaluate strength reliability and fractographic analysis was conducted.
Results
Highest flexural strength values were detected at post-sintered no-heat APA and 4W-laser groups (P < 0.05). Pre-sintered groups showed statistically lower flexural strength values. Heat treatment decreased the strength of the specimens. Monoclinic phase content was only detected at post-sintered no-heat groups and the highest amount was detected at APA group. Rougher surfaces and deeper irregularities were detected at FE-SEM images pre-sintered groups.
Conclusions
Application of surface treatments at pre-sintered stage may be detrimental for zirconia ceramics in terms of flexural strength.
Clinical significance
Treating the surface of zirconia ceramic before sintering process is not recommended due to significant decrease in flexural strength values. 2 W–4 W Er,Cr:YSGG laser irradiations can be regarded as alternative surface treatment methods when zirconia restoration would be subjected to veneer ceramic firing procedures.
1
Introduction
In the last decade, with increased aesthetic expectations of patients, the use of all-ceramic restorations in prosthodontics has become widely popular. Ceramic restorations have biocompatible advantages next to being aesthetic but they also have low fracture resistance [ ]. Currently, zirconia is one of the most popular ceramic material for clinicians because of its excellent optical behaviour and mechanical properties namely high flexural strength, fracture toughness and chemical durability [ ]. Hence, zirconia can be used in many dental applications such as framework for all-ceramic crowns and fixed partial dentures, monolithic restorations, post-core restorations, and implant abutments [ ].
Zirconia is a polymorphic material that can be found in three different crystalline forms depending on various temperatures as monoclinic, tetragonal and cubic. Monoclinic zirconia exists at temperatures lower than 1170 °C, and as the temperature increases, it transforms into the tetragonal and cubic phase [ ]. Zirconia material undergoes a unique microstructural transformation when a crack is encountered due to the stress. This is called the ‘transformation toughening mechanism’ resulting in an increase in volume, which can resist further propagation of the crack via tetragonal to monoclinic phase transformation [ ]. Due to the hydrothermal instability of zirconia, at low temperatures and in the presence of humidity, a tetragonal to monoclinic phase transformation occurs which is known as low temperature degradation [ ]. Microcracks in the surface and strength degradation of zirconia are the consequences of this aging process [ ].
Yttria stabilized tetragonal zirconia polycrystal (Y-TZP), magnesia partially stabilized zirconia (Mg-PSZ) and cubic stabilized zirconia (CSZ) are the current zirconia materials used in dental applications. Microstructures of Y-TZP and CSZ consist of tetragonal and cubic phases, respectively, while Mg-PSZ consists of tetragonal zirconia that dispersed in cubic zirconia. Y-TZP and Mg-PSZ can undergo transformation toughening mechanism phase however CSZ has not this mechanism and has inferior mechanical properties with advantageous optical properties [ ]. In recent years translucency of zirconia ceramics has improved by decreasing the alumina content, increasing density, decreasing grain size and adding cubic zirconia to fabricate full contour, monolithic zirconia restorations [ ]. Although novel zirconia microstructures have higher translucency [ ] to improve aesthetic appearance in anterior region, they still require to be veneered by translucent glassy ceramic [ ]. Clinical success and longevity of the zirconia restorations depend on the micromechanical interlocking and the strong bond between the veneering ceramic and zirconia core [ ]. However, it is difficult to achieve adequate bond strength between zirconia and the veneering layer and clinical failure generally occurs at the interface due to the delamination of the veneering ceramic and chipping [ ]. To enhance the adhesion between zirconia core and veneering ceramic, different surface treatment methods such as airborne-particle abrasion, grinding [ ], silica coating [ ], hot etching solution [ ] laser-etching [ ] were applied to the zirconia surface and these treatment methods have been evaluated in the literature. Roughening and changing the topography of the zirconia surface either by mechanical or chemical techniques increases the surface area and produces irregularities to create a stronger micromechanical bonding [ ].
Airborne-particle abrasion with Al 2 O 3 particles and laser etching methods have been reported to improve the bond strength between veneering ceramic and zirconia core [ ]. However, it has been suggested that the application of mechanical surface treatments to fully sintered zirconia might induce the tetragonal to monoclinic phase transformation of zirconia, thus reducing the strength and toughness, decreasing reliability and increasing the fracture risk of material [ ]. Furthermore, since two different crystal structures occur after the phase transformation, the coefficients of thermal expansion of the structures show discrepancies and the bonding capability of the zirconia is also affected [ ]. Therefore, application of surface treatments to pre-sintered zirconia has been recommended to prevent the phase transformation [ ]. It has been also reported that higher roughness values can be obtained on pre-sintered zirconia surfaces [ ]. However, the data regarding the effect of different surface treatment methods on the mechanical strength and microstructure of zirconia ceramic treated at pre-sintered and post-sintered stage is limited [ ].
Therefore, the aim of this in vitro study was to investigate the effects of airborne-particle abrasion and Er,Cr:YSGG laser irradiation with different output powers on 4-point-flexural strength, phase transformation and morphologic changes of zirconia ceramic treated at pre-sintered or post-sintered stage.
2
Materials and methods
2.1
Preparation of zirconia specimens
Test design is presented in Fig. 1 . Three hundred and forty-two bar-shaped specimens were milled with a 5-axes CAD-CAM machine (Sirona inLab MC X5, Dentsply Sirona, PA, USA) from partially sintered zirconia disks (GC Initial Zirconia Disk, GC Corporation, Tokyo, Japan) in 3 different sizes according to the test protocol applied ( Fig. 1 ). For 4-point flexural strength test, 190 bar-shaped specimens with dimensions of 1.4 × 4 × 20 mm were fabricated according to International Organization for Standardization (ISO) 6872:2015 [ ] recommendations. Seventy-six zirconia specimens in dimensions of 1 × 4 × 10 mm and 76 specimens in dimensions of 1 × 4 × 5 mm were fabricated for X-ray diffraction (XRD) and field emission scanning electron microscope (FE-SEM) analyses, respectively. All specimens were polished under water for 15 s with 600-, 800-, and 1200-grit silicon carbide abrasives (English Abrasives, London, UK), using a grinding/polishing machine at 300 rev/min (Phoenix Beta Grinder/Polisher; Buehler, Düsseldorf, Germany) to achieve standardized flat surfaces. Specimens subjected to flexural strength test, XRD and FE-SEM analyses were divided into subgroups according to surface and heat treatment protocols as showed in Table 1 .

Group name | Surface treatment protocol |
---|---|
Control | No surface treatment |
Pre2W | 2W Er,Cr:YSGG laser irradiation prior to sintering |
Pre3W | 3W Er,Cr:YSGG laser irradiation prior to sintering |
Pre4W | 4W Er,Cr:YSGG laser irradiation prior to sintering |
Pre5W | 5W Er,Cr:YSGG laser irradiation prior to sintering |
Pre6W | 6W Er,Cr:YSGG laser irradiation prior to sintering |
PreAPA | Airborne-particle abrasion prior to sintering |
Post2W | 2W Er,Cr:YSGG laser irradiation after sintering, no heat |
Post3W | 3W Er,Cr:YSGG laser irradiation after sintering, no heat |
Post4W | 4W Er,Cr:YSGG laser irradiation after sintering, no heat |
Post5W | 5W Er,Cr:YSGG laser irradiation after sintering, no heat |
Post6W | 6W Er,Cr:YSGG laser irradiation after sintering, no heat |
PostAPA | Airborne-particle abrasion after sintering, no heat |
Post2WHT | 2W Er,Cr:YSGG laser irradiation after sintering + heat treatment |
Post3WHT | 3W Er,Cr:YSGG laser irradiation after sintering + heat treatment |
Post4WHT | 4W Er,Cr:YSGG laser irradiation after sintering + heat treatment |
Post5WHT | 5W Er,Cr:YSGG laser irradiation after sintering + heat treatment |
Post6WHT | 6W Er,Cr:YSGG laser irradiation after sintering + heat treatment |
PostAPAHT | Airborne-particle abrasion after sintering + heat treatment |
2.2
Surface treatment methods
The specimens were randomly divided into 4 main groups whether the surface treatments applied before or after sintering and whether the specimens received heat treatment or not as (1) pre-sintered, (2) post-sintered no heat and (3) post-sintered heat-treated groups, and a group was served as (4) control with no surface treatment. Main groups that will receive surface treatment (1–3) were further divided into 6 equal subgroups according to the surface treatment method applied (2 W-, 3 W-, 4 W-, 5 W-, 6 W- Er,Cr:YSGG laser irradiations and airborne-particle abrasion) ( Table 1 ). In this manner, including the control group, a total of 19 test groups were obtained and each group was consisting of 10 specimens (n = 10). Surface treatment procedures were applied as follows:
2.2.1
Control group
Specimens received no surface treatment.
2.2.2
Airborne-particle abrasion
One entire surface of the specimens was subjected to airborne-particle abrasion with 110-μm Al 2 O 3 particles (Korox 110, Bego, Bremen, Germany) for 15 s at 2-bar pressure from a distance of 10 mm.
2.2.3
Er,Cr:YSGG laser irradiation
One entire surface of each specimen was irradiated with Er.Cr:YSGG laser (Waterlase MD, Biolase, Irvine, CA, USA) using a MG6 sapphire tip (Biolase Technology Inc., Irvine, CA, USA) on a non-contact hard tissue mode at energy level of 2–6 W according to subgroup, a repetition rate of 10 Hz, and 140 μs pulse duration with 55% water and 65% air. The tip scanned over the whole surface area at a 10 mm distance for 30 s.
The specimens in pre-sintered groups were subjected to surface treatments prior to sintering whereas the surface treatments were applied to specimens in post-sintered groups after sintering process. Specimens were sintered at 1450 °C for 8 h in a sintering furnace (Sirona inFire HTC speed, Dentsply Sirona, PA, USA) according to manufacturer’s instructions.
2.3
Heat treatment
Before flexural strength test, XRD and FE-SEM analyses, the specimens in heat treatment groups were heated in a ceramic furnace (800 °C) (Programat EP5000, Ivoclar Vivadent, Schaan, Liechtenstein) without veneering but simulating the veneering ceramic (Initial Zr-Fs Ceramic Dentin, GC Europe, Leuven, Belgium) firing cycle, according to manufacturer’s instructions.
2.4
Four-point flexural strength test
Four-point flexural strength test was performed by a universal testing machine (EZtest–500 N Shimadzu; Kyoto, Japan) following the instructions of ISO standard 6872:2015 [ ]. Each zirconia specimen with a 45° chamfer at major edges was placed on 2 steel sample holders (support rods) (1.5 mm in diameter) located with a 16 mm test span. The loading rods were located with an inner span of 8 mm. The specimens were positioned as the treated surface of the specimens was placed downward facing to supporting device (tension side) while the untreated surface was in contact with the loading rods (compression side). The load was applied with a crosshead speed of 1 mm/min until failure. The maximum load (N) was recorded and the flexural strength (σ) was calculated in MPa using the following formula:
(P: fracture load (N), L: length of test span (mm), w: width of the specimen (mm), and b: thickness of the specimen (mm), respectively).
During the flexural test procedure, a computer software (Nexygen, Lloyd Instruments, Farehem, England) was used to record load-deflection curves to determine flexural modulus of the specimens.
2.5
Weibull analysis
Weibull analysis was carried on the flexural strength data to obtain Weibull modulus and characteristic strength. Weibull distribution was determined using the following formula [ ]:
P f = 1 − exp [ ( − σ σ 0 ) m ]
(P f : fracture probability, σ: flexural strength, σ 0 : characteristic strength at fracture probability of 63.21%, and m: Weibull modulus which is equal to the slope of the ln(ln[1/(1 − P f )]) versus in ln σ plots).
2.6
XRD analysis
The phase compositions of 76 zirconia specimens were analysed (n = 4) by X-ray diffractometry (Smart Lab, Rigaku, Tokyo, Japan) at 2θ range between 20°–40° with a step size of 0.02° and a normalized count time of 1.8 s/step. The effect of surface treatments on monoclinic phase content (%) was evaluated by calculating the phase composition with the use of following formula [ ]:
Where X m is the monoclinic peak intensity ratio, I m (−111) and I m (111) are the monoclinic peak intensities at 2θ = 28.2° and 31.4°, respectively, and I t (111) is the tetragonal peak intensity at 2θ = 31.1°.
After the calculation of X m , monoclinic phase volume percentage (V m ) was calculated using the following formula [ ]:
2.7
FE-SEM analysis
FE-SEM analysis was conducted to investigate the surface topography of the specimens (n = 4). All specimens were coated with platinum/palladium prior to mounting on the stub of FE-SEM (SUPRA 55, Carl Zeiss, Oberkochen, Germany). Specimens were observed at an acceleration voltage of 15 kV and a magnification of 1000–25.000×. All measurements on digital FE-SEM images were performed by one blind calibrated examiner.
Fractographic analysis was performed with FE-SEM to evaluate the fractured surfaces of the zirconia specimens from different surface treatment groups. FE-SEM images were examined for arrest and hackle lines to determine the origin of fracture.
2.8
Statistical analysis
Shapiro–Wilks test was used for the assessment of the distribution of data and parametric tests were performed since the data showed a normal distribution. Flexural strength and flexural modulus values of control and test groups were analysed with two-way analysis of variance (ANOVA) for two factors (surface treatment and heat treatment) and Post hoc comparisons were carried out with the Bonferroni test, when significance was detected. Values of P < 0.05 were accepted as statistically significant.
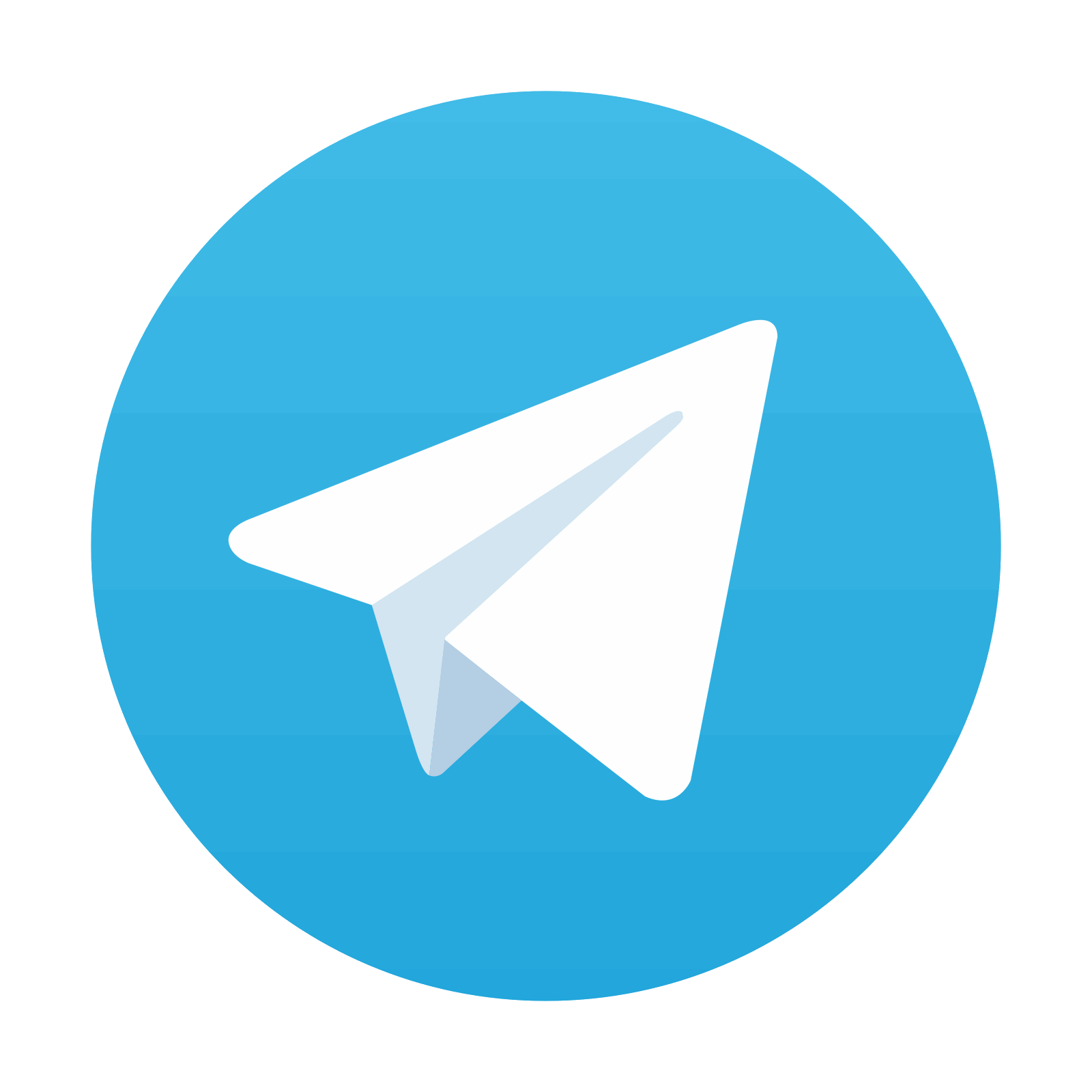
Stay updated, free dental videos. Join our Telegram channel

VIDEdental - Online dental courses
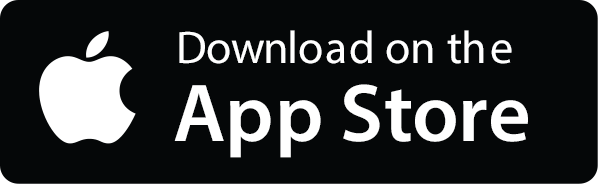
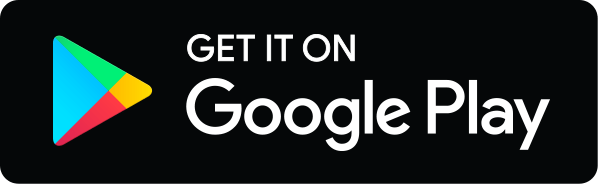