Introduction
Three-dimensional (3D) printing technologies enable production of orthodontic models from digital files; yet a range of variables associated with the process could impact the accuracy and clinical utility of the models. The objective of this study was to investigate the effect of print layer height on the accuracy of orthodontic models printed 3 dimensionally using a stereolithography format printer and to compare the accuracy of orthodontic models fabricated with several commercially available 3D printers.
Methods
Thirty-six identical models were produced with a stereolithography-based 3D printer using 3 layer heights (n = 12 per group): 25, 50, and 100 μm. Forty-eight additional models were printed using 4 commercially available 3D printers (n = 12 per group). Each printed model was digitally scanned and compared with the input file via superimposition analysis using a best-fit algorithm to assess accuracy.
Results
Statistically significant differences were found in the average overall deviations of models printed at each layer height, with the 25-μm and 100-μm layer height groups having the greatest and least deviations, respectively. Statistically significant differences were also found in the average overall deviations of models produced using the various 3D printer models, but all values fell within clinically acceptable limits.
Conclusions
The print layer height and printer model can affect the accuracy of a 3D printed orthodontic model, but the impact should be considered with respect to the clinical tolerances associated with the envisioned application.
Graphical abstract

Highlights
- •
3-D printing at different layer heights can affect accuracy of orthodontic models.
- •
Accuracy may also be affected by the type of printer.
- •
3-D printing in a higher resolution does not necessarily mean improved accuracy.
- •
Differences in accuracy may not be clinically significant.
- •
Selection of layer height and printer type should depend on intended application.
Digital advancements are changing the landscape of orthodontics. Although the fundamentals and applications of orthodontic treatment remain the same, the incorporation of digital systems and technologies transforms the workflow of the orthodontic office. One of the more recent advancements in this digital revolution in orthodontics is the incorporation of 3-dimensional (3D) printing technologies for the fabrication of tangible parts from digital files.
Although 3D printing technologies have been available for many years, the implementation of 3D printers in the orthodontic realm has increased significantly in the last decade. This is due in part to improved accuracy and decreased costs of the technologies. Today, there are various 3D printer options within reach of the practitioner. Two popular formats among commercially available 3D printers for orthodontic applications are inkjet 3D printing and stereolithography (SLA), the latter involving a photopolymerization process. There has been an increase in the number and availability of SLA-based printers in the last few years.
Many factors should be thoughtfully considered when selecting and applying a 3D printer in orthodontics. Variables to consider include printer resolution, cost (including maintenance and materials), and print time. Additional considerations include print orientation, any postprocessing steps required, print volume, and the footprint of the printer itself. Many of these variables could impact the accuracy of the printed part. SLA and inkjet 3D printers often allow the ability to control resolution by modulating the layer height of a printed object. This tuning of layer height impacts the time it takes the printer to finish a print and may also impact the accuracy of the print. Even at a given print layer height, the accuracy of the same printed part may differ between SLA printers.
Interestingly, little research has been reported regarding the accuracy and precision of 3D printers for orthodontic applications. Most of the literature that is available generally compares linear measurements of 3D printed models with plaster cast models. Khalil et al showed comparisons in accuracy of 4 printer types, but they did not evaluate an entire dental arch.
It is important to ensure that the digital variants of these diagnostic and clinical tools are both accurate and suitable for the envisioned applications. Therefore, the aims of this study were 2-fold: to investigate the effect of layer height on model accuracy when printed with an SLA printer and to compare the accuracy of several 3D printers presently marketed for dental and orthodontic use.
Material and methods
The maxillary arch of an ideal dental typodont (Nissin, Kyoto, Japan) was used as the basis for creation of a master stereolithography format (STL) file in accordance with reported methods ( Fig 1 , A ). The file was then optimized for 3D printing by cropping gingival and typodont base areas. An extruded vertical portion was also added to the base to facilitate preparations for superimposition. A chamfer was then placed at the base of the model to assist in removal from the print platform ( Fig 1 , B ). These modifications were accomplished using 3D modeling software (Meshmixer 3.1.373; Autodesk, San Rafael, Calif).

This master STL file was then used to 3 dimensionally print 36 identical resin models using a Form 2 printer (Formlabs, Somerville, Mass) and a grey photopolymer resin (FLGPGR02; Formlabs). Specifically, each print job yielded 3 resin models, and 4 print jobs were completed at each of the 3 layer heights (25, 50, and 100 μm) to produce a total of 36 models (12 per layer height). All models were printed so that the occlusal plane was parallel to the build platform, and the tooth cusps were oriented away from the build platform ( Fig 1 , C ).
At the completion of each print job, the models ( Fig 1 , D ) were removed from the build platform and washed in 2 separate immersion baths of 2-propanol for 10 minutes each, per the manufacturer’s instructions. The models were removed from the second bath and allowed to air dry at room temperature. The bottom of each model was labeled to identify its layer height and corresponding print job.
An additional 48 models based on the master STL file were fabricated from the following 3D printers by third-party laboratories: Juell 3D (Park Dental, New York, NY) (12 models printed with 100-μm layer height), Objet Eden260V Dental Advantage (Stratasys, Eden Prairie, Minn) (12 models printed with 28-μm layer height), large-frame Vector 3SP (EnvisionTEC, Dearborn, Mich) (12 models printed with 100 μm layer height), and Perfactory Desktop Vida (EnvisionTEC) (12 models printed with 100-μm layer height). The Objet printer varied from the other printers in vertical resolution because it is an inkjet printer and prints in a layer height of either 16 or 28 μm. All additional models were also printed so that the occlusal plane was parallel with the build platform, and the tooth cusps were oriented away from the build platform. The bottom of each model was labeled to identify its printer source and given a number for model identification.
All 84 printed models were digitally scanned using a R700 desktop scanner (3Shape, Copenhagen, Denmark) to produce an STL digital representation of the physical model. Lemos et al found that this scanner was reliable in producing a digital version of a physical model. The scanner was calibrated before use and all models placed in the same orientation on the scanner bed platform. Each digital model, including the modified master STL model, was cropped to exclude nonanatomic portions of the file. The digital model for each printed part (labeled as the test file) was then individually superimposed on the master STL file (labeled as the reference file) using an automated best-fit algorithm as shown in Figure 2 (Geomagic Control 2015.1.1; 3D Systems, Rock Hill, SC).

STL files essentially comprise a meshwork of triangulated surfaces, with points residing at the vertices of each mesh triangle. A 0.25-mm threshold parameter was set in the Geomagic software to analyze differences between the master digital file (reference file) and each superimposed model scan (test file) ( Fig 2 ). Any points in the test file deviating more than 0.25 mm in the positive and negative directions were considered to be beyond the upper and lower bounds, respectively ( Fig 3 ). Reports were generated for each superimposition that elucidated the total number of points in each superimposition and the total positive and negative deviations separately.

Statistical analysis
Mean average absolute deviations, mean percentages of points outside the upper and lower bounds, and mean total percentages of the points outside the bounds of the superimpositions were analyzed using 1-way analysis of variance (ANOVA) to examine the effect of layer height and type of printer, respectively. Histograms and stem-leaf plots were depicted and inspected to confirm the normality of the data. Least-square means and 95% confidence intervals (95% CI) were reported for each layer height or type of printer. Pairwise comparisons were conducted among all levels of the factors of study interest. The Tukey method was used to adjust for multiple pairwise comparisons. All P values were 2-sided, and P values less than 0.05 were considered significant. All statistical analyses were performed using statistical analysis software (SAS 9.4; SAS Institute, Cary, NC).
Results
One-way ANOVA ( P <0.001) indicated a statistically significant difference in the average absolute deviations between each of the 3 treatment groups printed with the Form 2 SLA printer (25, 50, and 100 μm). Post hoc comparisons indicated that the 100-μm layer height group had the least average overall deviation at 0.064 mm (95% CI, 0.060 mm-0.068 mm), followed by the 50-μm layer height group at 0.076 mm (95% CI, 0.072 mm-0.080 mm), and the 25-μm layer height group at 0.083 mm (95% CI, 0.079 mm-0.087 mm) ( Fig 4 ). The 100-μm layer height group also had the fewest points beyond the upper and lower bounds with a total at 0.38% (95% CI, 0.10%-0.65%), again followed by the 50-μm layer height group at 1.15% (95% CI, 0.87%-1.42%), which had fewer points beyond the positive and negative thresholds than did the 25-μm layer height group at 1.71% (95% CI, 1.44%-1.98%). The Tukey post hoc pairwise comparisons found each of these differences between layer height groups to be statistically significant ( P <0.05) ( Fig 5 ).
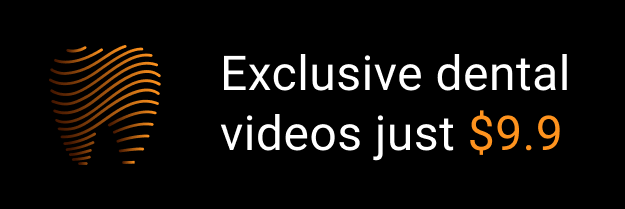