Highlights
- •
Montmorillonite can be successfully functionalised by a methacrylate-based compound with an increase of interlayer space from 11.6 Å to 14.2 Å.
- •
Dental resin composites can be prepared by mixing organically modified montmorillonite, barium glass and a methacrylate matrix.
- •
Substitution of clay results in higher storage modulus as observed by DMA.
- •
Clays may have a barrier effect to monomers release as observed in HPLC analysis and biological tests.
- •
Clays interfere with light transmittance as observed in spectrometry, water sorption and Vickers hardness.
Abstract
Objective
To prepare organically modified montmorillonite (OM MMT) and assess mechanical, physical, chemical and biological effects of its introduction into resin-composites.
Methods
Natural MMT clay was modified by a methacrylate functionalized quaternary ammonium intercalating agent. Interlayer distance was measured by X-ray diffraction. Dental composites were then prepared with x = 0, 1, 2.5, 5 or 7.5 wt.% of OM MMT, (75 − x) wt.% of silanated barium glass and 25 wt.% of methacrylate based matrix). Relative weight loss was measured and the effect of the substitution on mechanical properties was studied by dynamic mechanical analysis and hardness tests. Properties of resin composites were evaluated in terms of water sorption, light transmittance, biological tests and by high-performance liquid chromatography (HPLC).
Results
Resin based composites with well-dispersed organically modified MMT were successfully prepared. There were no significant weight loss differences shown by TGA within all samples. The DMA analysis showed that the introduction of clays have a beneficial effect in increasing the storage and elastic modulus of composites. Clay presence was shown to interfere with the blue light transmittance, affecting Vickers hardness and water sorption levels. The amount of released monomers measured from extracts was below expected levels for this type of materials and biological tests show satisfactory cell compatibility.
Significance
This paper reports the successful functionalization of MMT by a methacrylate group and further incorporation in experimental dental composites. Physical and biological results show a potential interest to the application of nanoclays into dental resin composites.
1
Introduction
Dental caries and its clinical management is still a major public health concern in dentistry all over the world. Recent publications have even reported an increase in global prevalence, resulting in a great demand for direct restorative materials. Mainly because of their aesthetic properties, dental composites are gradually becoming the preferred restorative solution adopted by the dentists. They are typically a mixture of methacrylate resin-based matrix, a photo initiator, and a high percentage of inorganic fillers that confer mechanical, optimal and radiopacity properties.
In spite of many improvements on dental composites since the early 60s, concerns still exist about composites longevity especially when materials are placed in high load bearing sites . To overcome this limitation, expectations for innovations lay on the development of composite systems with improved properties such as higher mechanical strength , fracture resistance and biocompatibility .
Nanoclay fillers are being commonly added to engineering composites as reinforcing phase to afford great properties enhancements in comparison with conventional micrometric counterparts . Notably, clay-based composites are of great interest for use in food packaging, protective coatings, and other applications where efficient barriers are needed but have not been widely studied in the dental restorative materials field.
These peculiar properties come from the natural clays inner structure composed of stack of 1 nm thick, 100 nm wide layered crystalline aluminosilicate sheets or platelets. Once unstacked and dispersed in a polymeric matrix the sheets can improve mechanical and barrier properties of the resulting nanocomposite .
Several steps are necessary to achieve clay platelets dispersion. Initially in natural clay, hydrated alkali or earth-alkali cations such as Na + or Ca 2+ are present in interlayer galleries to charge balance the negatively charged sheets . These sheets are linked together by means of weak polar and Van der Waals forces and are not easily exfoliated inside polymeric matrices. One of the first steps to incorporate clays into a polymeric matrix is to increase the inter platelet distance by ionically exchanging the cations by bulkier cationic surfactants such as ammonium salt solutions . The increased interlayer distance allows for an easier invasion of the clay galleries by the matrix polymeric chains. Furthermore, the ammonium salts can possess chemical functional groups that improve the clay compatibility with the matrix.
Montmorillonite is one of the most commonly used clay to produce polymer layered silicate nanocomposites and presents general formula (Al 2 Mg 3 )Si 4 O 10 (OH) 2 M + where M + is the monovalent cation. Few studies show the effect of clays on dental materials, but some promising yet preliminary results are reported in the current literature. The incorporation of ion exchanged and vinyl modified montmorillonite into dental monomers was successfully achieved even if the authors could not avoid the formation of agglomerates and did not obtain well distributed platelets into the composite bulk . PMMA modified montmorillonite has proven to be an effective reinforcing agent to dental adhesive monomers and results in significant improvements on material mechanical properties . However, for the production of experimental dental composites, the use of clay treated with ammonium salt did not improve the studied properties of the experimental materials . The step of replacing interlayer cations by organic molecules, producing an organically modified clay material, is not only interesting in terms of compatibilization of clay polarity with that of polymers but also to contribute to expand interlayer distance .
Clays are not easily exfoliated into individual layers and the successful exfoliation of layered silicates into a polymeric matrix remains a current scientific and technical challenge in material science . Recent publications on polymer-layered silicate nanocomposites suggest that controlling the exfoliation process is crucial to control the microstructure and the properties of the produced composites .
One of the most promising reactions to create clay mineral–polymer nanocomposites is the in situ polymerization of the corresponding monomers within the interlayer space. The monomers size allows for easier infiltration in clay interlayers galleries. Interlayer polymerization reactions were studied using 4-vinyl pyridine , acrylates , acrylonitrile , and others. In this study, an aqueous solution of methacrylate-terminated ammonium salt (2-(methacryloyloxy)ethyl trimethylamoniun chloride) solution was used to treat natural clay. This agent is expected to organically modify the clay, achieving a notably higher compatibility with the methacrylate monomers present in the matrix. The conditions are such that the polymerization is performed within the interlayer space, contributing to clay exfoliation. New organically modified montmorillonite clays and experimental resin based dental composites were then prepared and characterized. Microstructure of clays by X-ray diffraction (XRD) was investigated and the effect of organically modified clay content on physical, chemical, biological and optical properties of nanocomposites was evaluated. Our hypothesis is that the incorporation of organically modified montmorillonite (OM MMT) improves mechanical, physical, chemical and biological properties of resin-composites. The null hypothesis tested is that there is no difference in mechanical, physical, chemical and biological properties of resin-composites with the incorporation of organically modified montmorillonite (OM MMT).
2
Materials and methods
Organically modified clay was prepared by cation exchange of natural montmorillonite (MMT) clay (Cloisite ® Na+, BYK Additives Inc.) with 2-(methacryloyloxy)ethyl trimethylamoniun chloride solution (TCI Europe). Natural clay was dispersed in water using magnet stirrer at ambient temperature for 30 min and with the application of ultrasound (UP400S, Hielscher) at ambient temperature for another 30 min with 3 mm sonotrode at 100% amplitude. An excess of salt solution was calculated from CEC 0.92 meq/g and added little by little under ultrasound agitation. The resulting treated clay was washed 5 times with distilled water to remove salt excess, dried at 65 °C, ground and sieved below 63 μm.
X-ray diffraction (XRD) was performed on dry natural and treated clay powder. The scans were run using a diffractometer (X’Pert PRO, PANanalytical MPD) with Cu Kα radiation ( λ = 1,54 Å) under room temperature with an angle range 2 θ from 4° to 10° at 2°/min. The equipment was operating at 45 kV and 30 mA.
The methacrylate matrix was prepared by mixing 40 wt.% urethane dimethacrylate (UDMA), 30 wt.% bisphenol A glycidyl methacrylate (BisGMA), 30 wt.% triethylene glycol dimethacrylate (TEGDMA) and traces of camphorquinone and dihydroxyethyl-para-toluidine (DHEPT) (all items were supplied by Esstech Inc.) under controlled light.
Experimental dental composites were prepared under controlled light using 75 wt.% of fillers and 25 wt.% of matrix by mechanical mixing (SpeedMixer, Flacktek Inc.) for 1 min at room temperature. The fillers were composed of silanated barium glass powder (8235 UF0.7 sil 4.2%, SCHOTT Glass) and 0, 1, 2.5, 5 and 7.5 wt.% substitution by treated clay comprising 75 wt.% filler content. Obtained composites are homogeneous, presenting adequate consistency to prepare all the samples. Composites were stored in dark pots and manipulated under controlled light during each utilization.
The thermal degradation of composites was studied by thermogravimetric analysis (TGA) using a Nietzsch TG209 F3 Tarsus equipment. Weight changes were followed as a function of temperature increase from 30 °C to 650 °C at a heating rate of 10 °C/min in air atmosphere (flow 20 ml/min) in 10–20 mg spherical composite samples. Samples were polymerized for 40 s (2 times 20 s) using a LED light-curing device with high light intensity at 1.000 mW/cm 2 (Celalux 2, VOCO). All samples were prepared using the same light-curing device.
The thermo-mechanical response of the composites was evaluated using a home-made Dynamic Mechanical Analysis (DMA) apparatus in torsion mode at 1 Hz from 223 K (−50 °C) to 423 K (150 °C). Samples had typically 20 mm length (the distance between grips around 15 mm), 6–7 mm width and 0.35–0.55 mm thickness. Samples were polymerised in only one side placing the exit window of the external energy source at one extremity of the specimen, covering the largest area possible. This section was irradiated for 40 s (2 times 20 s). The exit window was moved to the next section, slightly overlapping the previous section, and irradiated for more 40 s. This analysis output is the elastic, storage or Young’s modulus (G′), which is an indicator of elastic behavior and the viscous or loss modulus (G″), a measure of the viscous response of the material.
Disc samples of 15 mm diameter and 1 mm height (n = 3) were prepared by filling split moulds with composites and photocured over a polyester strip for 40 s according to ISO 4049 . No lubricating agent was used. Samples were vertically installed into supports with 5 mm spam between each sample and transferred to a vacuum oven at 37 °C until a constant mass in μg is obtained (METLER TOLEDO AE 100). After final drying, diameter and the thickness were measured and the volume V (mm 3 ), calculated. Then, the samples installed on the supports were immersed in 60 ml of distilled water at (37 ± 1) °C for 7 days. At the end of 7 days, samples were removed from water, water excess removed and mass m 2 (μg) weighed. A new cycle of drying was performed until constant mass m 3 (μg) is obtained. Water sorption was calculated according with the following formula:
W a t e r s o r p t i o n = m 2 − m 3 V
For Vickers hardness tests, bar samples of 2 × 2 × 25 mm (n = 6) were prepared by filling split moulds with composites and photocured over a polyester strip in 3 sections for 40 s (Celalux 2, VOCO) for each section. Samples were then removed from the mould and immersed in distilled water for 24 h at 37 °C prior to hardness tests using 300 g load for 10 s, on top (surface receiving the polymerization light) and bottom sides of samples.
Composite discs of 10 mm diameter and 1 mm thick were prepared. UV–vis spectrophotometer (V670, Jasco) was used to evaluate absorbance and transmittance of light in the wavelength range of 330–800 nm using an integrating sphere sample holder. Additionally to the composites, discs prepared with pure matrix and a mix of matrix with 5 wt.% of clay were prepared.
Cytotoxicity of experimental dental composites was tested by using extracts obtained as described in ISO 10993-5 . Composites were placed in disc molds (n = 10; 10 mm diameter × 2 mm height), covered with a polyester strip on both sides to control surface finish and polymerised for 10 s on each side (Celalux 2, VOCO). Immediately after polymerization, each sample was weighted, and placed into a UV chamber for 15 s/face to avoid any possible contamination. Extracts of composites were prepared by adding the discs into sterile tube with medium culture following the ratio of 1 mL of medium for each 0.2 g of material, and incubating for 24 h at 37 °C with 5% of CO 2 and humidified atmosphere and then stored at 4 °C. Human Dermal Fibroblasts (HDFa) cells (Tebu-bio) at passage 7 were seeded (5000 cells/wells) in 96-wells microtiter plate with RPMI (Roswell Park Memorial Institute) medium supplemented with 10% of fetal calf serum, 1% of penicillin–streptomycin and incubated at 37 °C with 5% CO 2 under humidified atmosphere for 24 h. RPMI medium, fetal calf serum and penicillin–streptomycin were purchased from DUTSCHER. After 24 h, extracts were diluted in cell culture medium at 25, 50, 75 and 100% (pure extract) and pipetted into the cell wells under controlled conditions. Medium was distributed to obtain 200 μL total/well. Extracts were left in contact with the cells for 24 h, 3 and 6 days . Cell growth was evaluated using PrestoBlue™ technique (Invitrogen, Carlsbad, California, USA), which allows following the culture during several days. PrestoBlue™ reagent uses resazurin (blue and non-fluorescent) which is quickly reduced by metabolically active cells in resorufin (red–pink and highly fluorescent), providing a quantitative measure of viability and cytotoxicity . Fluorescence intensity was measured using a fluorimeter (INFINITE PRO 200, Tecan) with excitation at 535 nm, emission at 610 nm and gains at 40 and 38. The cell viability is expressed as a percentage relative to the number of cells in the negative control (polystyrene treated for cell culture) tested during the same experiment. To study reproducibility, each condition was tested on three samples, and each evaluation was realized three times (n = 9).
High-performance liquid chromatography (HPLC) analysis was performed with an Agilent 1200 series (Agilent Technologies) with a guard cartridge and a C18 reverse phase column (Jupiter 5 μm, 250 × 4.6 mm, Phenomenex) using non-diluted medium extracts (100% extracts) obtained for cytotoxicity tests. Separation was carried out at room temperature, with a flow rate of 1 mL/min, using solvent A acetonitrile/water mixture (20:80 vol./vol.) and solvent B acetonitrile 100%. A linear gradient was applied during chromatography, starting with 0% of solvent B and increasing to 100% at the end. Detection was performed by a diode array detector (G1315B). The monitoring wavelengths were 280 nm and 460 nm for Camphorquinone (CQ). The results were analyzed with the Chemstation for LC 3D systems (Agilent Technologies). For reference, the following standards were used BisPhenol A, Bis-GMA, Camphorquinone (CQ), TEGDMA, UDMA. The standards were prepared at concentrations of 10, 20 and 50 μg/mL using water and acetonitrile (50:50). These reference standards were used to generate a standard curve. 150 μL of each sample was taken and an equal volume of acetonitrile (HPLC grade) was added. The samples were mixed very well by vortexing and centrifuged at 100 rpm for 5 min. Finally, the samples were analyzed by HPLC using an injection volume of 100 μL (three injections per sample). The same raw materials used to produce the composites served as references to equipment calibration, except from Bisphenol A that was purchased from Fluka, St. Louis, MO, USA.
One-way analysis of variance (ANOVA) test, followed by post-hoc HSD Tukey-Kramer for multiple comparisons between groups was used to test significant differences, at a significance level set at p ≤ 0.05 (JMP 11.2.1, SAS Institute, UK). Spearman (ρ) rank correlation tests were used to verify the significance of correlations between water sorption, Vickers hardness, storage and loss modulus, light transmittance and the amount of BISGMA and TEGDMA leached by the composites.
2
Materials and methods
Organically modified clay was prepared by cation exchange of natural montmorillonite (MMT) clay (Cloisite ® Na+, BYK Additives Inc.) with 2-(methacryloyloxy)ethyl trimethylamoniun chloride solution (TCI Europe). Natural clay was dispersed in water using magnet stirrer at ambient temperature for 30 min and with the application of ultrasound (UP400S, Hielscher) at ambient temperature for another 30 min with 3 mm sonotrode at 100% amplitude. An excess of salt solution was calculated from CEC 0.92 meq/g and added little by little under ultrasound agitation. The resulting treated clay was washed 5 times with distilled water to remove salt excess, dried at 65 °C, ground and sieved below 63 μm.
X-ray diffraction (XRD) was performed on dry natural and treated clay powder. The scans were run using a diffractometer (X’Pert PRO, PANanalytical MPD) with Cu Kα radiation ( λ = 1,54 Å) under room temperature with an angle range 2 θ from 4° to 10° at 2°/min. The equipment was operating at 45 kV and 30 mA.
The methacrylate matrix was prepared by mixing 40 wt.% urethane dimethacrylate (UDMA), 30 wt.% bisphenol A glycidyl methacrylate (BisGMA), 30 wt.% triethylene glycol dimethacrylate (TEGDMA) and traces of camphorquinone and dihydroxyethyl-para-toluidine (DHEPT) (all items were supplied by Esstech Inc.) under controlled light.
Experimental dental composites were prepared under controlled light using 75 wt.% of fillers and 25 wt.% of matrix by mechanical mixing (SpeedMixer, Flacktek Inc.) for 1 min at room temperature. The fillers were composed of silanated barium glass powder (8235 UF0.7 sil 4.2%, SCHOTT Glass) and 0, 1, 2.5, 5 and 7.5 wt.% substitution by treated clay comprising 75 wt.% filler content. Obtained composites are homogeneous, presenting adequate consistency to prepare all the samples. Composites were stored in dark pots and manipulated under controlled light during each utilization.
The thermal degradation of composites was studied by thermogravimetric analysis (TGA) using a Nietzsch TG209 F3 Tarsus equipment. Weight changes were followed as a function of temperature increase from 30 °C to 650 °C at a heating rate of 10 °C/min in air atmosphere (flow 20 ml/min) in 10–20 mg spherical composite samples. Samples were polymerized for 40 s (2 times 20 s) using a LED light-curing device with high light intensity at 1.000 mW/cm 2 (Celalux 2, VOCO). All samples were prepared using the same light-curing device.
The thermo-mechanical response of the composites was evaluated using a home-made Dynamic Mechanical Analysis (DMA) apparatus in torsion mode at 1 Hz from 223 K (−50 °C) to 423 K (150 °C). Samples had typically 20 mm length (the distance between grips around 15 mm), 6–7 mm width and 0.35–0.55 mm thickness. Samples were polymerised in only one side placing the exit window of the external energy source at one extremity of the specimen, covering the largest area possible. This section was irradiated for 40 s (2 times 20 s). The exit window was moved to the next section, slightly overlapping the previous section, and irradiated for more 40 s. This analysis output is the elastic, storage or Young’s modulus (G′), which is an indicator of elastic behavior and the viscous or loss modulus (G″), a measure of the viscous response of the material.
Disc samples of 15 mm diameter and 1 mm height (n = 3) were prepared by filling split moulds with composites and photocured over a polyester strip for 40 s according to ISO 4049 . No lubricating agent was used. Samples were vertically installed into supports with 5 mm spam between each sample and transferred to a vacuum oven at 37 °C until a constant mass in μg is obtained (METLER TOLEDO AE 100). After final drying, diameter and the thickness were measured and the volume V (mm 3 ), calculated. Then, the samples installed on the supports were immersed in 60 ml of distilled water at (37 ± 1) °C for 7 days. At the end of 7 days, samples were removed from water, water excess removed and mass m 2 (μg) weighed. A new cycle of drying was performed until constant mass m 3 (μg) is obtained. Water sorption was calculated according with the following formula:
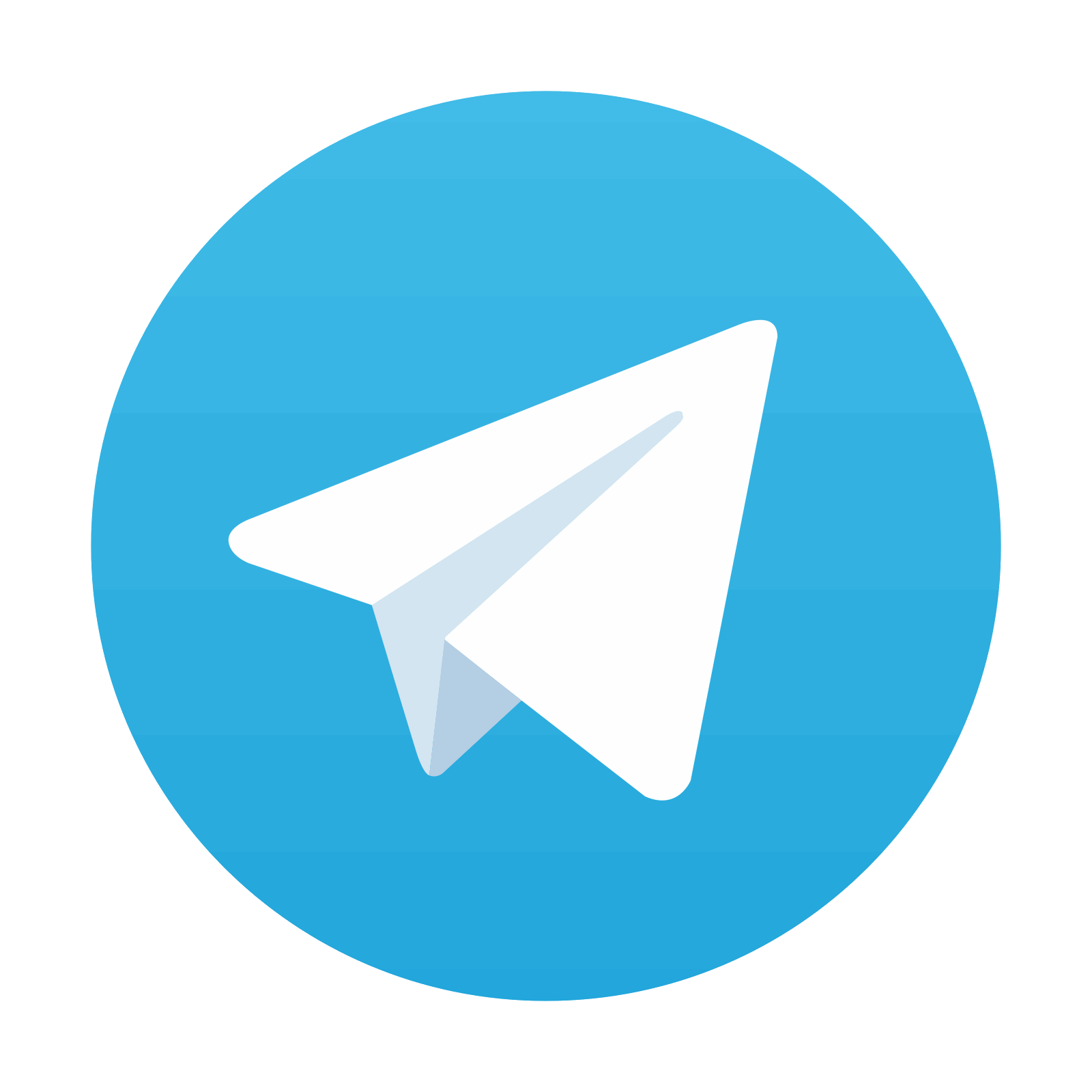
Stay updated, free dental videos. Join our Telegram channel

VIDEdental - Online dental courses
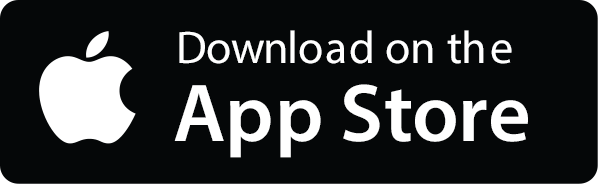
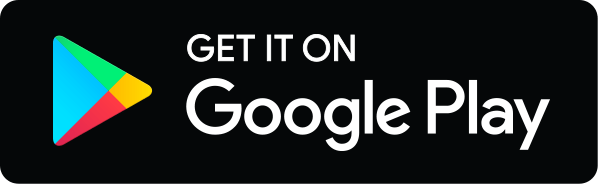