Introduction
This study aimed to evaluate the craniofacial effects of microimplant assisted rapid palatal expansion (MARPE) on bone-anchored maxillary protraction (BAMP) through a finite element analysis.
Methods
A 3-dimensional finite element model of the skull with associated sutures was created from the computed tomography image of a 12-year-old male patient. Two protraction protocols: BAMP without MARPE (protocol 1) and BAMP with MARPE (protocol 2), were analyzed using Ansys software (Ansys, Canonsburg, Pa). Stress distribution in the sutures and displacement pattern of craniofacial structures were analyzed in the 2 protocols using finite element analysis.
Results
Both protocols produced changes in craniofacial structures in all the 3 planes. Displacement of the maxilla was more pronounced in protocol 2 in all directions with mild clockwise rotation. Protocol 1 displayed a translatory movement of the maxilla without any rotation and mild constriction in the anterior region. In protocol 2, an expansion of the maxilla, which increased in the superoinferior direction, was also observed. Von Mises stress in circummaxillary sutures was significantly more in protocol 2, indicating an increased displacement of craniomaxillary structures.
Conclusions
The use of MARPE during BAMP enhanced maxillary protraction and reduced the counterclockwise rotation tendency of the maxilla. Hence, it may be inferred that incorporation of MARPE during BAMP protocol may prove beneficial in the treatment of patients with skeletal Class III malocclusion with open bite tendency or hyperdivergent growth pattern.
Highlights
- •
MARPE enhances maxillary protraction during BAMP
- •
MARPE reduces the counterclockwise rotation tendency of the maxilla.
- •
Incorporation of MARPE during BAMP proves beneficial in hyperdivergent growth patterns.
For several years, the cornerstone of early skeletal Class III malocclusion treatment has been the orthopedic face mask (FM), anchored from the maxillary teeth with or without rapid maxillary expansion (RME). The objective of this treatment modality is to enhance the growth at the sutures by applying additional force to separate them. However, along with the forward displacement of the maxilla, this therapy results in certain unwanted side effects such as extrusion and mesialization of the maxillary molars, proclination of maxillary incisors, retroclination of mandibular incisors, clockwise rotation of the mandible, and increased vertical dimension of the face. The use of titanium miniplates as anchorage for applying bone-borne orthopedic forces has revolutionized Class III treatment by enhancing midfacial growth without the attendant side effects of FM therapy. This is accomplished by applying reverse-pull forces from maxillary implants instead of maxillary teeth.
Transverse maxillary deficiency with unilateral or bilateral posterior crossbite is a common finding in patients with Class III malocclusion, which needs to be corrected. Maxillary protraction therapy is thus very often accompanied by palatal expansion. Moreover, the palatal expansion also disarticulates the sutures and initiates a cellular response which enhances maxillary protraction. However, being a tooth-borne appliance, this could lead to buccal tipping and dehiscence of maxillary posterior teeth. In recent years, the incorporation of microimplants for expansion has helped in alleviating undesirable dental side effects, resulting in significantly better outcomes. Such microimplant assisted rapid palatal expanders (MARPE), when combined with FM, reported further enhancement in the forward movement of the maxilla in a short period. The skeletal expansion results in effective disarticulation of the circummaxillary sutures, which is postulated to be a key factor in efficient and effective maxillary protraction.
Another method of intercepting developing skeletal Class III malocclusion is to apply bilateral Class III elastic traction from implants placed in the maxillary infrazygomatic region to implants placed in the mandible, mesial to the canines. This method, called bone-anchored maxillary protraction (BAMP), is a protocol reported to produce significantly larger maxillary advancement than conventional RME and FM therapy displaying better vertical control without adverse effects on the dentition. , However, clinical outcomes of incorporating MARPE into BAMP have not been studied so far. It is possible that skeletal expansion will enhance the positive outcomes of BAMP protocol as well.
The finite element model (FEM) analysis is a noninvasive method of analyzing clinical situations virtually and assessing the displacement and stress distribution pattern in different clinical protocols. This study aimed to evaluate the stress distribution and displacement pattern when transverse forces are applied using MARPE during BAMP protocol and also compare the skeletal and dentofacial effects of BAMP with and without MARPE. The null-hypothesis generated is that there would be no change in the displacement and stress distribution pattern in craniofacial structures and sutures after BAMP with or without MARPE.
Material and methods
A FEM was constructed from the spiral computed tomographic images of the skull of a 12-year-old growing person obtained from the Department of Radiology, Government Medical College. The scan parameters are tube voltage 120 kV, 360 mA, matrix size of 512 × 512, and a slice thickness of 0.3 mm and voxel 0.463 × 0.463 × 0.300. The scan data were saved as digital imaging and communications in medicine (DICOM) files. The raw DICOM data from the computerized tomography (CT) scan were imported into Mimics software (version 8.11, Materialise Interactive Medical Image Control System; Materialise, Leuven, Belgium), in which the DICOM images of the scan were further processed to extract maxilla, mandible, teeth, periodontal ligament, and other soft tissues and then converting it into stereolithographic file format. The data in stereolithographic files were exported to Rapidform software to convert it into a geometric model (lines and surfaces) in Initial Graphics Exchange Specification format.
The expander, miniscrews, and miniplates were modeled as computer-aided design geometry using computer-aided 3-dimensional interactive application software (Dassault Systèmes, Paris, France) and positioned according to the situation in the live patient by using the CT images as positioning aids. The material properties were assigned into the geometric models according to the information provided by the manufacturer. The combined geometric model is further imported into Hypermesh software (version 13.0, Altair Hypermesh; Altair Engineering, Inc, Detroit, Mich) to convert into FEMs. The geometric models undergo a process called meshing, in which these models are divided into a finite number of elements. These elements are connected at a finite number of points called nodes . The meshing condition is shown in Figure 1 . In this study, 10 node solid tetrahedral elements were used. Two FEMs were established in this study: one showing maxillary protraction using BAMP without MARPE and the other with MARPE. Both models contained 465,091 elements and 101,247 nodes each.

The mechanical properties of the structures such as bone, teeth, and miniscrews in this model were obtained from experimental data from previous studies ( Table I ). These mechanical properties were found to be isotropic and linear elastic. The thickness of the cortical bone was modeled according to Farnsworth et al ; the thickness of the periodontal ligament was 0.2 mm, and the sutures were 0.5 mm.
Material | Young’s modulus (N/mm 2 ) | Poissons’s ratio |
---|---|---|
Cortical bone | 1.34 × 10 4 | 0.30 |
Cancellous bone | 0.78 × 10 4 | 0.30 |
Miniplate | 10.3 × 10 4 | 0.33 |
Miniscrew | 10.3 × 10 4 | 0.33 |
Suture | 68.65 | 0.40 |
Tooth | 2.07 × 10 4 | 0.30 |
Periodontal ligament | 0.68 | 0.49 |
Nodes along with the foramen magnum and center of the forehead were fully constrained by all degrees of freedom with zero rotation and zero displacements.
Two FEM models were created to simulate the 2 protocols ( Fig 2 ). Protocol 1 simulates BAMP in which 2 miniplates are placed in the infrazygomatic crest in the maxilla and 2 in the mandible between the canine and lateral incisor on either side. Fixation was done using titanium screws (2 for each maxillary implant and 3 for mandibular). The length of the screw was 5 mm and diameter 2.3 mm. Elastics were engaged from the miniplates in the infrazygomatic crest to that in the mandible using 250 g of protraction force. In protocol 2, maxillary skeletal expansion was also incorporated along with BAMP. This was done using MARPE, which is a bone-borne palatal expander having 4 microscrews. The microscrews were inserted into the palate 3 mm lateral to the midpalatine suture. These microscrews were attached to the expansion screw, and the arms of the expansion screw were designed to be soldered to the bands of the first molars and first premolars. Here, along with 250 g of protraction force, 800 g of palatal expansion force per side was applied bilaterally. Forces were directed transversely at the site of insertion of microscrews at the apex of the palate, 3 mm lateral to midpalatal suture.

The analysis was done using Ansys software (version 12.1; Ansys, Canonsburg, Pa), in which the nodal and element solutions were plotted for both protocols. Stress distribution and displacement of selected landmarks in the maxillofacial bone were analyzed. Displacements of various craniofacial structures were evaluated along the x-, y-, and z-coordinates. The x-coordinate indicates transverse direction, y-coordinate indicates anteroposterior direction, and z-coordinate shows vertical direction. Positive values in the x-axis indicate movements to the right side, and negative values show movements to the left. In the y-axis, positive values show backward movement, and negative values indicate forward movement. In the z-axis, upward movements are indicated by positive values, and negative values show the downward movement of the maxillary complex. The landmarks to assess the displacement pattern of maxillofacial bones are given in Table II .
Area of examination | Selected landmarks |
---|---|
Maxilla | Frontal process of the maxilla |
Nasion | |
Inferior part of nasal bone | |
ANS | |
PNS | |
Point A | |
Frontal process of the zygoma | |
Maxillary process of zygoma | |
Temporal process of the zygoma | |
Maxillary central incisor tip | |
Maxillary dentition | Maxillary first molar’s palatal cusp tip in the occlusal plane |
Results
A comparison of the displacement pattern in x, y, and z-planes is shown in Figure 3 , and the amount of displacement of the selected landmarks in both the protraction protocols is shown in Table III . Protocol 1 in the sagittal plane shows that all points in the nasomaxillary complex moved forward except the superior point of the nasal bone, superior border of the frontomaxillary process, and the superior end of zygomaticofrontal process. The ANS moved anteriorly and inferiorly by 0.31 mm and 0.21 mm, respectively. The PNS also moved anteriorly and inferiorly by 0.32 mm and 0.21 mm, respectively, indicating the absence of rotation of the maxilla. A very mild constriction of the anterior maxilla in the transverse plane is observed. The zygomatic bone moved anteriorly, laterally, and inferiorly. The lateral walls of the nasal cavity showed significant expansion. The inferior border of the nasal bone moved anteriorly, with the superior end showing a posterior movement. Point A and the tip of the central incisor moved anteriorly by 0.32 mm and 0.32 mm, respectively.
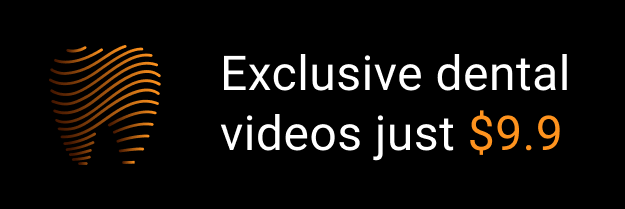