Introduction
The objective of this study was to investigate the effect of low-level laser therapy (LLLT) on the rate of orthodontic tooth movement (OTM) into bone-grafted alveolar defects based on different healing states.
Methods
Ten male beagles were randomly allocated to 3 groups: group C, OTM alone as a control; group G, OTM into the grafted defects; group GL, OTM into the grafted defects with LLLT. The maxillary second premolars were protracted into the defects for 6 weeks, immediately (G-0 and GL-0) and at 2 weeks (G-2 and GL-2) after surgery. The defects were irradiated with a diode laser (dose, 4.5 J/cm 2 ) every other day for 2 weeks. The rates of OTM and alveolar bone apposition, and maturational states of the defects were analyzed by histomorphometry, microcomputed tomography, and histology.
Results
The total amounts of OTM and new bone apposition rates were decreased by LLLT, with increased bone mineral density and trabecular maturation in the defects. Group GL-2 had the slowest movement with root resorption in relation to less woven bone in the hypermatured defect.
Conclusions
LLLT significantly decreased the rate of OTM into the bone-grafted surgical defects by accelerating defect healing and maturation, particularly when the start of postoperative OTM was delayed.
Highlights
- •
We studied the effect of low-level laser therapy (LLLT) on orthodontic tooth movement.
- •
Tooth movement and new bone apposition rate decreased after LLLT.
- •
Bone mineral density and trabecular maturation in the defects increased after LLLT.
- •
LLLT accelerated defect healing and maturation.
Clinically, alveolar bone grafts have been applied to extend the range of orthodontic tooth movement (OTM) by overcoming anatomic limitations such as alveolar cleft, pathologic or traumatic alveolar defect, dehiscence or fenestration, and constricted alveolar ridge. In terms of enhancing the rate of OTM with successful defect regeneration after bone grafts, the timing of postsurgical orthodontic intervention is a critical factor, in addition to various surgical factors. Several studies have recommended 3 to 6 weeks as the minimal time to wait before initiating OTM, on the basis of graft stability and tissue regeneration. It was reported that the rates of OTM showed no significant differences between grafted and nongrafted defects when the force had been applied at 12 weeks after surgery, the fully matured stage. Another study found the fastest OTM in the group with the worst defect regeneration when OTM into the grafted alveolar cleft started at 8 weeks after surgery. The authors proposed that OTM earlier than 4 weeks after grafting might be a more beneficial choice, since most grafted materials are absorbed at 4 weeks after implantation, followed by obvious bone formation at 8 weeks. In our previous study, the fastest OTM into the surgical defect was seen in the immediate traction group with bone grafts and in the 2-week traction group without grafts. OTM was rather inhibited in the 12-week traction group compared with the controls and was accompanied by poor defect repair. It was suggested that the combined effect of defect healing potentials, osteoconduction by bone graft, and increased remodeling activities by OTM might influence the timing of optimal intervention after surgery.
Low-level laser therapy (LLLT) has been reported to enhance the healing potential of surgical defects filled with bone grafts. Weber et al found that LLLT led to a biostimulatory effect on the healing process of femoral defects grafted with autologous bone in rats. de Almeida et al suggested that LLLT favored the healing process; they showed that the quantity of new bone formation was similar to an autogenous bone graft in the rat’s calvarial defect. These effects include the stimulation of blood flow and angiogenesis, earlier onset of inflammatory responses, increased synthesis of collagen and growth factors, osteosynthesis by activation of osteoblasts and reduction in osteoclastic activity, and improvement in bone strength in relation to hypermineralization. However, most previous studies investigated these acceleratory effects on general bones such as long bones or calvaria that have different properties from the alveolar bone surrounding the teeth. Alveolar bone has a higher turnover rate even in the physiologic state, and its regenerative potential after injury could be improved when accompanied by OTM. There has been no study investigating the effect of LLLT on the healing of alveolar bone defects with OTM.
LLLT and dentoalveolar surgery have been separately investigated for accelerated tooth movement and alveolar remodeling activity through the regional acceleratory phenomenon. The combined effect of LLLT and surgical injury on the rate of OTM was investigated in our previous study to verify whether it would be synergistic. Interestingly, periodic laser irradiation after surgical cortical activation (corticision) around a moving tooth decreased the rate of OTM, possibly because of the accelerated healing of surgical sites induced by LLLT. It was postulated that the effect of LLLT on the remodeling activity of surgically injured bone surrounding the moving tooth might be different from that of sound alveolar bone. The healing process modulated by LLLT might depend on various conditions such as the type of surgical injury, the extent or intensity of the surgical damage, and the additional stimulation of defect healing.
So far, it has been questionable whether LLLT accelerates the rate of OTM into the grafted defect by stimulating paradental remodeling activities, or decelerates the rate of OTM by stimulating defect maturation dominantly. The objective of this study was to investigate the effect of LLLT on the rate of OTM into bone-grafted surgical alveolar defects based on different healing states.
Material and methods
Ten male beagle dogs (age, 18-24 months; weight, 10-13 kg) were housed in separate cages according to the guidelines of Kyung Hee Medical Center’s institutional animal care and use committee (approval number KHMC IACUC 11-007). Twenty maxillary second premolars were randomly allocated to 3 groups to receive the following treatments: group C, OTM alone as a baseline control (n = 4); group G, OTM into a grafted defect without LLLT (n = 8); and group GL, OTM into a grafted defect with LLLT (n = 8). The experimental groups were further divided into 2 subgroups (n = 4), based on the timing of orthodontic force application after surgery: immediate (groups G-0 and GL-0) and 2-weeks postoperative (groups G-2 and GL-2).
Under general anesthesia by intramuscular injection of Zoletil 50 (0.25 mg/kg; Virbac Laboratories, Carros, France), 4-wall, cuboidal, critical-sized defects of 5 (mesiodistal) × 5 (buccolingual) × 7 (vertical) mm that included the extraction sockets of the maxillary first premolars were created ( Fig 1 , A ). The distal wall of each defect was prepared so that 1.5 mm of interseptal bone remained along the mesial surface on the mesial root of the maxillary second premolar to prevent the immediate fracture of the interseptal wall during OTM. The defect was immediately filled with a 1:1 mixture of deproteinized bovine bone mineral (Bio-Oss; Geistlich Sons, Wolhusen, Switzerland) and demineralized bone matrix (OrthoBlast II; IsoTis, Irvine, Calif) ( Fig 1 , B ). The mucosa was adjusted and sutured. For postoperative care, anti-inflammatory analgesics (Ketopro; Uni Biotech Co, Chungnam, Korea) and antibiotics (Gentamycin; Komipharm International Co, Shiheung, Korea) were administered intramuscularly twice a day for 6 days, and a surgical dressing with 0.12% chlorhexidine gluconate rinse was applied daily.
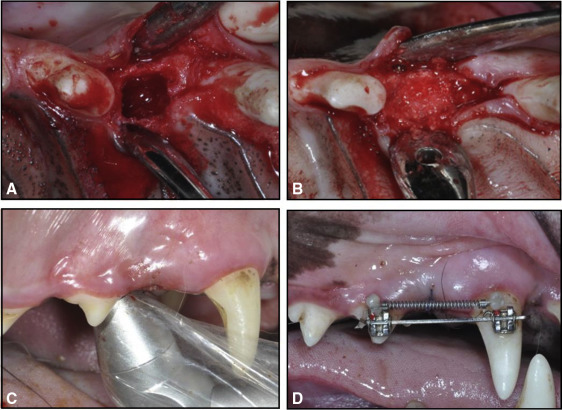
The animals of group GL had 8 sessions of LLLT every other day for 2 weeks from the day of surgery. A gallium-aluminum-arsenide diode laser (Laser Hand; MM Optics, São Carlos, Brazil) with a wavelength of 780 nm was used in the biostimulation mode. The preset mode of continuous waves at 70 mW output power, as suggested by the manufacturer, was delivered through a conventional tip with a diameter of 0.5 cm in direct contact with the tissues, producing a low intensity of 1.75 W per square centimeter. An energy dose (4.5 J/cm 2 ) was applied for 7 seconds to 4 points around the defect ( Fig 1 , C ).
Brackets (Tomy, Tokyo, Japan) were bonded on the buccal surfaces of the maxillary second premolars and canines using Super-bond C&B (Sun Medical, Shiga, Japan). Sectional stainless steel wires (0.017 × 0.025 in) were passively inserted into the 2 brackets for minimizing unwanted movement, such as tipping or rotation. For mesial movement of the second premolar, a nickel-titanium closed-coil spring (light force; 3M Unitek, Monrovia, Calif) was activated with a 100-g force using the canine as the anchorage tooth ( Fig 1 , D ). Throughout the 6 weeks, the force applied was checked and readjusted once a week in all groups. All animals were killed at 6 weeks after OTM initiation by direct injection of Zoletil 50 into the heart.
The amount of OTM was measured on precisely fabricated stone models and recorded weekly. It was calculated as the difference of the distances measured from the mesial cervix of the second premolar to the mesial cervix of the first molar. All measurements were repeated twice by 1 expert investigator (S.-J.K.) at 2-week intervals. The average and weekly rates of movement were calculated and compared.
Microcomputed tomography images were obtained not only to describe the type of OTM, but also to quantitatively analyze the defects’ maturational states. Alveolar bone specimens (N = 20), including the second premolar and the defect, were harvested and fixed immediately using 10% formalin for 48 hours. Images were taken from the fixed blocks with settings of 130 kV(p) source voltage, 30 μA electricity, and 44.7 μm image pixel size. The volume of interest in the defect was defined as 3.5 × 2.8 × 2.5 mm 3 to be consistently confined to the core defect and the regenerative tissues. Three representative longitudinal sections were taken from each block (total n = 3 × 4 = 12/group) and used for the measurement of microstructural morphometric parameters including bone mineral density, percent bone volume, percent surface volume, trabecular thickness, trabecular number, and trabecular separation using SkyScan 1173 (Bruker, Kontich, Belgium).
To determine both the anabolic modeling rate on the tension side of the moved tooth and the regeneration rate of the defect, sequential fluorescent labels were measured under a fluorescence microscope (BH-2; Olympus Optical, Tokyo, Japan) with an ultraviolet filter (l¼ 515 nm) in nondecalcified specimens. All animals had been injected intramuscularly with oxytetracycline hydrochloride (Fluka Chemie AG, Buchs, Switzerland; yellow orange, 30 mg/kg) at 24 hours before OTM; alizarin red (Fluka Chemie AG; red, 30 mg/kg) at 3 weeks; and calcein (Fluka Chemie AG; green, 10 mg/kg) at 6 weeks after OTM. Photomicrographs were obtained using a digital charge-coupled device camera (PS30C; Kappa Optronics, Gleichen, Germany). Along the outline of the labeled bone traced from the microphotographs, 10 sites were randomly selected in the upper half of the roots for linear measurements of newly formed mineralized bone (n = 10/group), and the mean values were used (Metreo version 2.5; Kappa Optronics) to support indirectly the assessment of the rate of OTM.
Half of the tissue blocks were decalcified with 10% ethylene diaminetetraacetic acid (EDTA-2Na, pH 7.4) at 48°C for 30 days and dehydrated in ethyl alcohol of gradually increasing concentrations from 70% to 100%. After the samples were embedded in paraffin, they were sectioned sagittally into 6-μm-thick sections. They were stained with hematoxylin and eosin and were examined under a light microscope for evaluation of the alveolar bone modeling around the moved tooth and the healing state of the surgical defect. The proportion (%) of different tissue components in the defect was measured in the specimen stained with Masson trichrome using a Tomoro Scope Eye (version 3.5.197; JNOpTIC, Seoul, Korea).
Statistical analysis
Intraobserver differences for each variable, evaluated with paired t tests, showed no significance ( P >0.05). Accordingly, the mean of the data sets was used for further description. The normality of data distribution was assessed by the Shapiro-Wilk test, and the homogeneity of the variances was checked by the Levene test. One-way analysis of variance (ANOVA) and Tukey post hoc tests were performed for intergroup comparisons of the mean accumulated distances of OTM, mean apposition of newly mineralized bone on the tension side, and 6 microstructural parameters.
Results
The mean accumulated distances ( Fig 2 , A ) and the weekly rates of OTM for 6 weeks ( Fig 2 , B ) were significantly lower in the laser-irradiated groups than in the nonirradiated groups. Group GL-0 showed significantly less movement (1.59 ± 0.23 mm) than did group G-0 (3.44 ± 1.25 mm), maintaining a higher level of movement than did group C (1.28 ± 0.13 mm). Group GL-2 exhibited less movement (1.06 ± 0.35 mm) than did group G-2 (2.42 ± 0.84 mm), thus having values farther below group C ( Table I ). There were remarkable differences in the weekly rates between the irradiated and nonirradiated groups during the first 3 weeks.
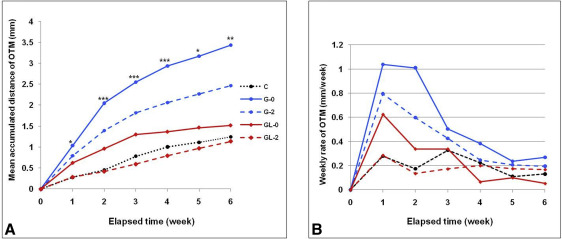
Elapsed time (wk) | No LLLT | LLLT | P value | Multiple comparison | |||
---|---|---|---|---|---|---|---|
Group C (a) | Group G-0 (b) | Group G-2 (c) | Group GL-0 (d) | Group GL-2 (e) | |||
1 | 0.25 ± 0.10 | 1.04 ± 0.20 | 0.80 ± 0.14 | 0.65 ± 0.13 | 0.26 ± 0.09 | 0.037 ∗ | b > c, d > a, e |
2 | 0.46 ± 0.06 | 2.05 ± 0.18 | 1.39 ± 0.14 | 0.97 ± 0.11 | 0.35 ± 0.06 | 0.000 ‡ | b > c > d > a, e |
3 | 0.66 ± 0.08 | 2.55 ± 0.16 | 1.82 ± 0.12 | 1.26 ± 0.18 | 0.46 ± 0.08 | 0.000 ‡ | b > c > d > a, e |
4 | 1.04 ± 0.09 | 2.93 ± 0.15 | 1.91 ± 0.12 | 1.36 ± 0.14 | 0.73 ± 0.15 | 0.000 ‡ | b > c > d > a, e |
5 | 1.16 ± 0.14 | 3.14 ± 0.18 | 2.15 ± 0.47 | 1.50 ± 0.15 | 0.93 ± 0.29 | 0.013 ∗ | b > c > d, a, e |
6 | 1.28 ± 0.13 | 3.44 ± 1.25 | 2.42 ± 0.84 | 1.59 ± 0.23 | 1.06 ± 0.35 | 0.006 † | b > c > d > a, e |
We measured the rate of alveolar modeling on the triple fluorochrome-stained images. The mean apposition rates of newly mineralized bone on the tension side of the moved tooth ( Fig 3 ) corresponded to the mean values of OTM. LLLT significantly decreased the mean apposition rates during alveolar modeling for 6 weeks, indicated by the distance between the yellow and green labels, consistent with the decreased rate of OTM. Instead of thin demarcated lines with long intervals as seen in group G, thick and diffuse bands with short or no gaps were found in group GL. Group GL-0 had lower apposition rates especially in the first 3 weeks (from yellow to red labels) compared with group G-0. Group GL-2 showed lower rates than did group G-2 in both the first and the last 3 weeks (from yellow to red, and from red to green labels), and was comparable with the rate of group C. In addition to cortical surface modeling, intracancellous osteonal remodeling was remarkably observed in the irradiated groups.
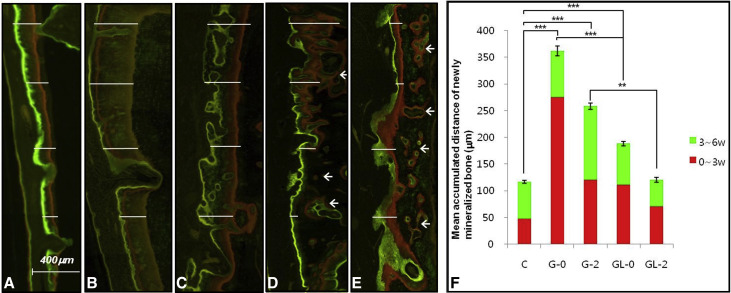
For the morphometric analysis of the microcomputed tomography images, the representative images described the defect healing states as well as the types of OTM ( Fig 4 , A-D ). The types of OTM in group GL were crown tipping without remarkable root movement, unlike translations that were seen in group G. As a result of morphometric analyses on 6 microstructures of the regenerate bones ( Table II ), group GL had significantly higher bone mineral density ( P <0.001), trabecular thickness ( P <0.05), and trabecular number ( P <0.05) than did group G. No intragroup differences based on the timing of the OTM were detected. Bone volume ratio ( P >0.05) and surface ratio ( P >0.05) showed no significant differences between the groups.
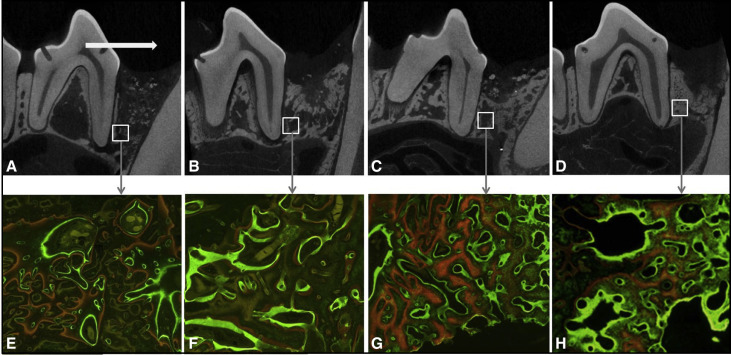
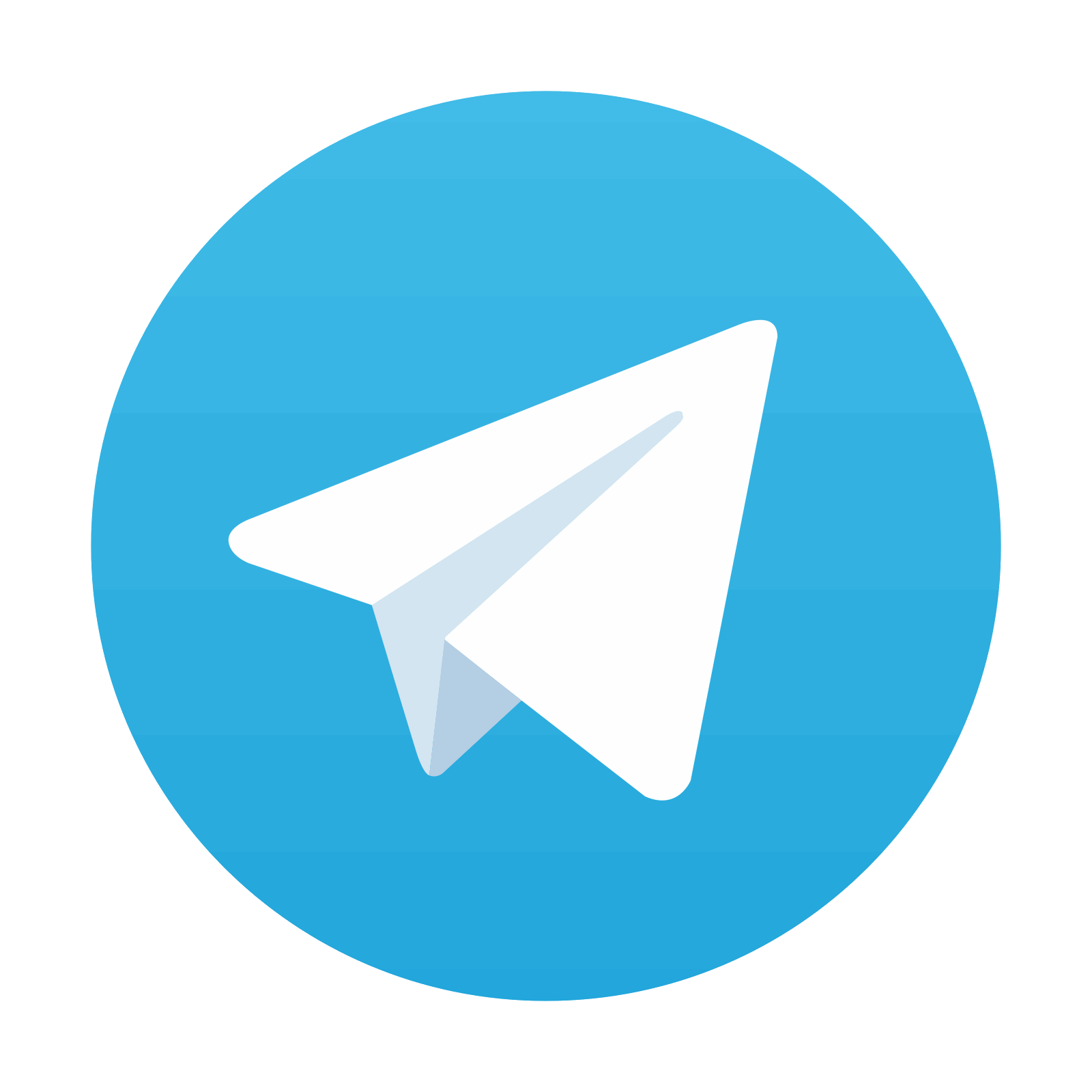
Stay updated, free dental videos. Join our Telegram channel

VIDEdental - Online dental courses
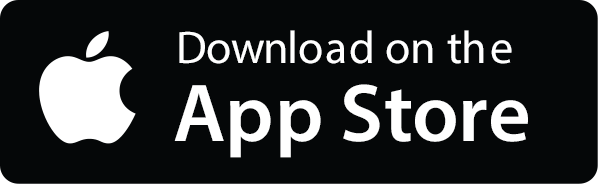
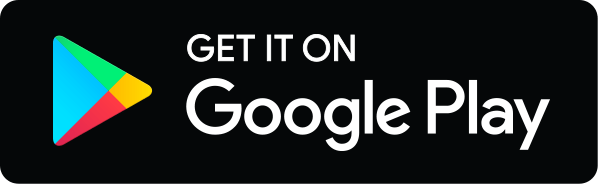
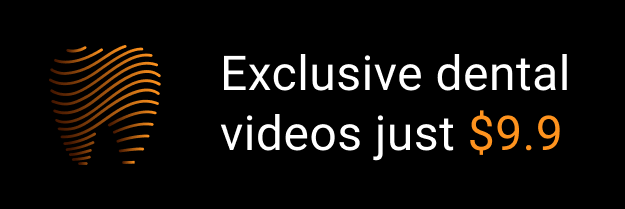