Abstract
Objectives
The temperature variations during the veneering firing cycles of a zirconia dental ceramic can negatively affect its mechanical properties. A possible synergistic effect of both heat-treatment and aging while exposed to the oral environment could result to catastrophic failure. The aim of the present study was to investigate the effect of heat treatment followed during veneering and in vitro aging on the mechanical and microstructural properties of zirconia dental ceramics.
Methods
Three specimens from each of two zirconia blocks (Ivoclar IPS e.max ZirCAD (IV) and Wieland ZENO Zr (WI)) were cut by CAD/CAM technology, fully sintered and polished. Each one was cut in four equal parts. One part was used as control (C), one was heat-treated (H), one was aged (A) (134 °C, 2 bar, 10 h) and one was heat-treated and subsequently aged (HA). The mechanical properties (nano-hardness ( H ) and elastic modulus ( E* )) were investigated by nano-indentation tests while the surface characterization was carried out with XRD, FTIR and SEM.
Results
Different treatments on IV and WI samples resulted in a reduction of both H and E* values, however the differences were not statistically significant ( p > 0.05). The combination of treatments imposes an overall effect ( p < 0.001), enhancing the influence on both H and E* values. This reduction in mechanical properties was followed by an increase of monoclinic content. Greater variations in both H and E* values were recorded for WI samples.
Significance
The clinical performance of zirconia dental ceramics may be affected during firing and aging resulting in increased probability of failure.
1
Introduction
Y-TZP zirconia ceramics have attracted the interest of dental technology in manufacturing core materials for fixed all-ceramic restorations due to their excellent mechanical properties (i.e. bending strength and toughness) . The high strength of Y-TZP zirconia ceramics is attributed to a toughening mechanism related to the transformation of the tetragonal phase ( t ), to the monoclinic ( m ) phase (natural equilibrium) . This t→m transformation may be triggered by an applied stress and is associated with a net 4 vol% expansion due to the larger volume occupied by the monoclinic phase compared to the tetragonal one . In case of a pre-existing crack the volume expansion associated to the t→m transformation, enables the closure of the crack and diminishes its propagation. This crack propagation prevention minimizes the risk of catastrophic failure of the material due to fracture and is called the “transformation toughening mechanism” .
The main drawback of Y-TZP zirconia ceramics is their sensitivity to low temperature degradation (LTD) – aging that leads to exaggerated t→m transformation and a degradation that starts on the surface and propagates into the depth of the material, diminishing its mechanical properties . This is of particular interest for dental zirconia ceramics, as their degradation in the oral environment due to the exposure to oral fluids and mechanical stress over prolonged periods of time cannot be overlooked. Although minimally exposed to the oral environment, the margins of zirconia restorations allow a continuous contact of zirconia core with saliva or other fluids that may start and progressively lead to the degradation of the material. Furthermore, there is growing popularity of monolithic zirconia restorations where much larger areas of zirconia are in contact with the oral environment.The majority of the models proposed to explain the spontaneous t→m transformation taking place during LTD-aging, are either based on the formation of zirconium hydroxides (Zr-OH) or yttrium hydroxides (Y(OH) 3) or Y(O)OH) due to the diffusion of water through the material, promoting phase transition with local stress concentration or variation of the yttrium/zirconium ratio. According to the most recent proposed model oxygen anions are responsible for the transformation nucleation and therefore for the LTD. Due to volume changes the transformed grains cause microcracks and the material becomes degraded.
Currently, accelerated tests at intermediate temperatures (100–300 °C) are the only basis for the estimation of the transformation rate and, hence, of the product lifetime , although a lot of controversy exists about the validity of the extrapolated predictions . According to Chevalier aging of zirconia with 1 h of autoclave treatment at 134 °C and 2 bar pressure results in a significant t→m transformation that has theoretically the same effect as 3–4 years in vivo, while the ISO standard imposes a maximum of 25 wt% of monoclinic zirconia to be present after an accelerated aging test conducted for 5 h at 134 °C and 2 bar . However Lughi and Sergo state that these accelerating in vitro tests provide only a rough estimate and any extrapolation could lead to a significant error in estimating body temperature lifetimes.
To achieve highly aesthetic zirconia restorations, zirconia core is veneered with a feldspathic porcelain coating and is subjected to firing at high temperatures (750–900 °C) followed by subsequent cooling. This process takes place at least once, but usually it takes two to five firing cycles in order to obtain an aesthetically acceptable restoration . The fracture strength , microhardness and flexural strength are significantly reduced after veneering firing cycles. According to Oilo et al. this effect is predominantly observed after the first firing cycle while subsequent cycles do not cause a further deterioration of properties. The reduced mechanical properties of zirconia ceramics after heat-treatment have been attributed to residual compressive stresses due to milling and various processing steps – such as grinding and sandblasting – that are released during the heat treatment, as well as to the t→m transformation and the alteration of the grain size that take place during the firing cycles of the veneering process . This degradation of mechanical properties can further reduce the strength of the material after exposure to the oral environment, so a possible synergistic effect of both heat-treatment and aging could result to a probably irreversible premature failure.
The aim of the present work was to investigate the effect of heat treatment followed during veneering and the in vitro aging on critical mechanical and microstructural properties at the nano-scale of cold isostatic-pressed zirconia ceramics for dental restorations. The research hypotheses investigated were:
- 1.
the heat treatment does not affect the nano-hardness ( H ) and the elastic ( E* ) constant of the zirconia ceramic cores;
- 2.
the in vitro aging does not affect the nano-hardness ( H ) and the elastic ( E* ) constant of the zirconia ceramic cores;
- 3.
the combination of heat-treatment and subsequent in vitro aging does not affect the nano-hardness ( H ) and the elastic ( E* ) constant of the zirconia ceramic cores.
2
Materials and methods
Three bar-shaped specimens (28 mm × 4 mm × 2 mm) milled from two zirconia blocks [Ivoclar IPS e.max ZirCAD (LOT: PX0075), and Wieland ZENO Zr (LOT:20090728-07)] by the CAD/CAM technology were sintered to full density, polished with diamond pastes of 3 and 1 μm under running water until a mirror-like surface was achieved, and cut with a diamond bur under water cooling into four equal parts each (7 mm × 4 mm × 2 mm). One part was used as control (designated as C), one was heat treated (designated as H), one was aged (designated as A) [steam 121 °C/2 bar/10 h, Kavo autoclave sterilizer 2100 (KavoDental, Biberach/Riss, Germany)] and the last was heat-treated and subsequently aged (designated as HA). The applied heat-treatment corresponded to four firing cycles of the veneering porcelain as shown in Table 1 according to manufacturers’ instructions.
Firing cycle | 1st shoulder | 1st dentin | 2nd dentin | Glazing | ||||
---|---|---|---|---|---|---|---|---|
IV | WI | IV | WI | IV | WI | IV | WI | |
B (standby temperature) | 403 °C | 575 | 403 °C | 575 | 403 °C | 575 | 403 °C | 575 |
S (pre-heating temperature time) | 4ʹ | 8 | 4ʹ | 9 | 4ʹ | 8 | 6ʹ | 5 |
T ↗ (heating rate) | 50 °C/min | 45 | 50 °C/min | 45 | 50 °C/min | 45 | 60 °C/min | 45 |
T (final temperature) | 800 °C | 980 | 750 °C | 900 | 750 °C | 890 | 725 °C | 880 |
H (holding time at T ) | 1ʹ | 1 | 1ʹ | 2 | 1ʹ | 1 | 1ʹ | 1 |
V 1 (vacuum in) | 450 °C | 575 | 450 °C | 575 | 450 °C | 575 | 450 °C | – |
V 2 (vacuum out) | 799 °C | 980 | 749 °C | 900 | 749 °C | 890 | 724 °C | – |
2.1
Nano-mechanical properties evaluation
The H and E* values were assessed by means of a Hysitron Ubi-1 TriboLab modular nano-indentation instrument (Tribolab, Hysitron Incorporated, Minneapolis). A pyramidal diamond Berkovich indenter with a total included angle of 142.3°, and a tip with a radius of curvature of approximately 120 nm, has been utilized. In all cases, a trapezoidal loading–unloading profile was used, with 5 s loading and unloading segments, including a 2 s holding segment. The H and E* values were calculated from the experimental unload–displacement curves using the Oliver and Pharr model .
Nano-indentations in all samples were performed with constant load of 4 mN. This load was selected after tests with varying loads performed on the untreated samples, based on the aspect that the produced indentation should have a contact depth higher than one half of the tip’s radius of curvature. This is necessary, in order to have proper onset of plasticity. The contact depth, in all indentation tests, was kept between 80 and 110 nm. The eight different groups of materials were produced in triplicates and each sample was tested in two different locations, where ten indentations were made in every location, thus making a total of 20 indentation prints in every sample and a total of 60 in each different material. Every experimental point in Fig. 2 a and b is the mean value of 60 indentations.
In order to reach equilibrium with the thermal mass inside the cabinet, all samples were placed into the acoustic and thermal enclosure of the nano-indenter apparatus for several hours prior to testing. Before performing each array of indents, the stability of the nano-indentation instrument was checked by measuring a fused-quartz reference sample. In all cases, the measured values of H and E* of the fused-quartz reference sample were in good agreement with the values reported by the manufacturer, i.e. 9.25 GPa and 69.6 GPa, respectively.
2.2
Statistical analysis
Descriptive statistics were calculated by means of min, max, median, mean and standard deviation. The assumption of normality was tested with the Shapiro–Wilk test and it was not rejected. Levene’s test for equality of variances was used to test the homogeneity of variances assumption which also was not rejected. One-way ANOVA was performed to investigate statistically significant differences between groups of specimens, while pair wise comparisons were conducted with the Bonferroni multiple comparison tests for the adjustment of the Type I error. Two independent samples t test were performed to investigate statistically significant differences between the two zirconia ceramics. The analysis was performed with the SPSS 15.0 software and the statistical significance was set for p < 0.05 .
2.3
Surface characterization
X-ray diffraction (XRD) surface analysis was carried out for the determination of the amount of m-ZrO 2 transformation using a Philips diffractometer (PW1710; Philips, Eindhoven and Almelo, The Netherlands) with Ni-filtered CuKα radiation. The counting parameters of the XRD study were: step size: 0.05° 2 θ , start angle: 5°, end angle: 53° and scan speed: 1, 2° 2 θ /min. The monoclinic phase fraction Xm was calculated according to the Garvie and Nicholson method. Fourier Transform Infrared Spectroscopy (FTIR) was used to qualitatively evaluate the m-ZrO 2 transformation. The FTIR reflectance spectra of the specimens were obtained in the spectral area of MIR and FIR (4000–120 cm −1 ), using a Brüker FTIR extended spectrometer, model IFS113v with a resolution of 2 cm −1 . Scanning electron microscopy-Energy dispersive spectroscopy (SEM-EDS) analysis was performed on the surfaces of carbon-coated specimens (J.S.M. 840A; JEOL, Tokyo, Japan) in order to observe any differences concerning surface morphology or elemental composition owed to the various treatments applied. SEM microphotographs of arbitrarily selected specimens were obtained and backscattered microphotographs were collected. Chemical analyses were performed with 20 kV accelerating voltage and 0.4 mA probe current. Pure Co was used as an optimizing element.
2
Materials and methods
Three bar-shaped specimens (28 mm × 4 mm × 2 mm) milled from two zirconia blocks [Ivoclar IPS e.max ZirCAD (LOT: PX0075), and Wieland ZENO Zr (LOT:20090728-07)] by the CAD/CAM technology were sintered to full density, polished with diamond pastes of 3 and 1 μm under running water until a mirror-like surface was achieved, and cut with a diamond bur under water cooling into four equal parts each (7 mm × 4 mm × 2 mm). One part was used as control (designated as C), one was heat treated (designated as H), one was aged (designated as A) [steam 121 °C/2 bar/10 h, Kavo autoclave sterilizer 2100 (KavoDental, Biberach/Riss, Germany)] and the last was heat-treated and subsequently aged (designated as HA). The applied heat-treatment corresponded to four firing cycles of the veneering porcelain as shown in Table 1 according to manufacturers’ instructions.
Firing cycle | 1st shoulder | 1st dentin | 2nd dentin | Glazing | ||||
---|---|---|---|---|---|---|---|---|
IV | WI | IV | WI | IV | WI | IV | WI | |
B (standby temperature) | 403 °C | 575 | 403 °C | 575 | 403 °C | 575 | 403 °C | 575 |
S (pre-heating temperature time) | 4ʹ | 8 | 4ʹ | 9 | 4ʹ | 8 | 6ʹ | 5 |
T ↗ (heating rate) | 50 °C/min | 45 | 50 °C/min | 45 | 50 °C/min | 45 | 60 °C/min | 45 |
T (final temperature) | 800 °C | 980 | 750 °C | 900 | 750 °C | 890 | 725 °C | 880 |
H (holding time at T ) | 1ʹ | 1 | 1ʹ | 2 | 1ʹ | 1 | 1ʹ | 1 |
V 1 (vacuum in) | 450 °C | 575 | 450 °C | 575 | 450 °C | 575 | 450 °C | – |
V 2 (vacuum out) | 799 °C | 980 | 749 °C | 900 | 749 °C | 890 | 724 °C | – |
2.1
Nano-mechanical properties evaluation
The H and E* values were assessed by means of a Hysitron Ubi-1 TriboLab modular nano-indentation instrument (Tribolab, Hysitron Incorporated, Minneapolis). A pyramidal diamond Berkovich indenter with a total included angle of 142.3°, and a tip with a radius of curvature of approximately 120 nm, has been utilized. In all cases, a trapezoidal loading–unloading profile was used, with 5 s loading and unloading segments, including a 2 s holding segment. The H and E* values were calculated from the experimental unload–displacement curves using the Oliver and Pharr model .
Nano-indentations in all samples were performed with constant load of 4 mN. This load was selected after tests with varying loads performed on the untreated samples, based on the aspect that the produced indentation should have a contact depth higher than one half of the tip’s radius of curvature. This is necessary, in order to have proper onset of plasticity. The contact depth, in all indentation tests, was kept between 80 and 110 nm. The eight different groups of materials were produced in triplicates and each sample was tested in two different locations, where ten indentations were made in every location, thus making a total of 20 indentation prints in every sample and a total of 60 in each different material. Every experimental point in Fig. 2 a and b is the mean value of 60 indentations.
In order to reach equilibrium with the thermal mass inside the cabinet, all samples were placed into the acoustic and thermal enclosure of the nano-indenter apparatus for several hours prior to testing. Before performing each array of indents, the stability of the nano-indentation instrument was checked by measuring a fused-quartz reference sample. In all cases, the measured values of H and E* of the fused-quartz reference sample were in good agreement with the values reported by the manufacturer, i.e. 9.25 GPa and 69.6 GPa, respectively.
2.2
Statistical analysis
Descriptive statistics were calculated by means of min, max, median, mean and standard deviation. The assumption of normality was tested with the Shapiro–Wilk test and it was not rejected. Levene’s test for equality of variances was used to test the homogeneity of variances assumption which also was not rejected. One-way ANOVA was performed to investigate statistically significant differences between groups of specimens, while pair wise comparisons were conducted with the Bonferroni multiple comparison tests for the adjustment of the Type I error. Two independent samples t test were performed to investigate statistically significant differences between the two zirconia ceramics. The analysis was performed with the SPSS 15.0 software and the statistical significance was set for p < 0.05 .
2.3
Surface characterization
X-ray diffraction (XRD) surface analysis was carried out for the determination of the amount of m-ZrO 2 transformation using a Philips diffractometer (PW1710; Philips, Eindhoven and Almelo, The Netherlands) with Ni-filtered CuKα radiation. The counting parameters of the XRD study were: step size: 0.05° 2 θ , start angle: 5°, end angle: 53° and scan speed: 1, 2° 2 θ /min. The monoclinic phase fraction Xm was calculated according to the Garvie and Nicholson method. Fourier Transform Infrared Spectroscopy (FTIR) was used to qualitatively evaluate the m-ZrO 2 transformation. The FTIR reflectance spectra of the specimens were obtained in the spectral area of MIR and FIR (4000–120 cm −1 ), using a Brüker FTIR extended spectrometer, model IFS113v with a resolution of 2 cm −1 . Scanning electron microscopy-Energy dispersive spectroscopy (SEM-EDS) analysis was performed on the surfaces of carbon-coated specimens (J.S.M. 840A; JEOL, Tokyo, Japan) in order to observe any differences concerning surface morphology or elemental composition owed to the various treatments applied. SEM microphotographs of arbitrarily selected specimens were obtained and backscattered microphotographs were collected. Chemical analyses were performed with 20 kV accelerating voltage and 0.4 mA probe current. Pure Co was used as an optimizing element.
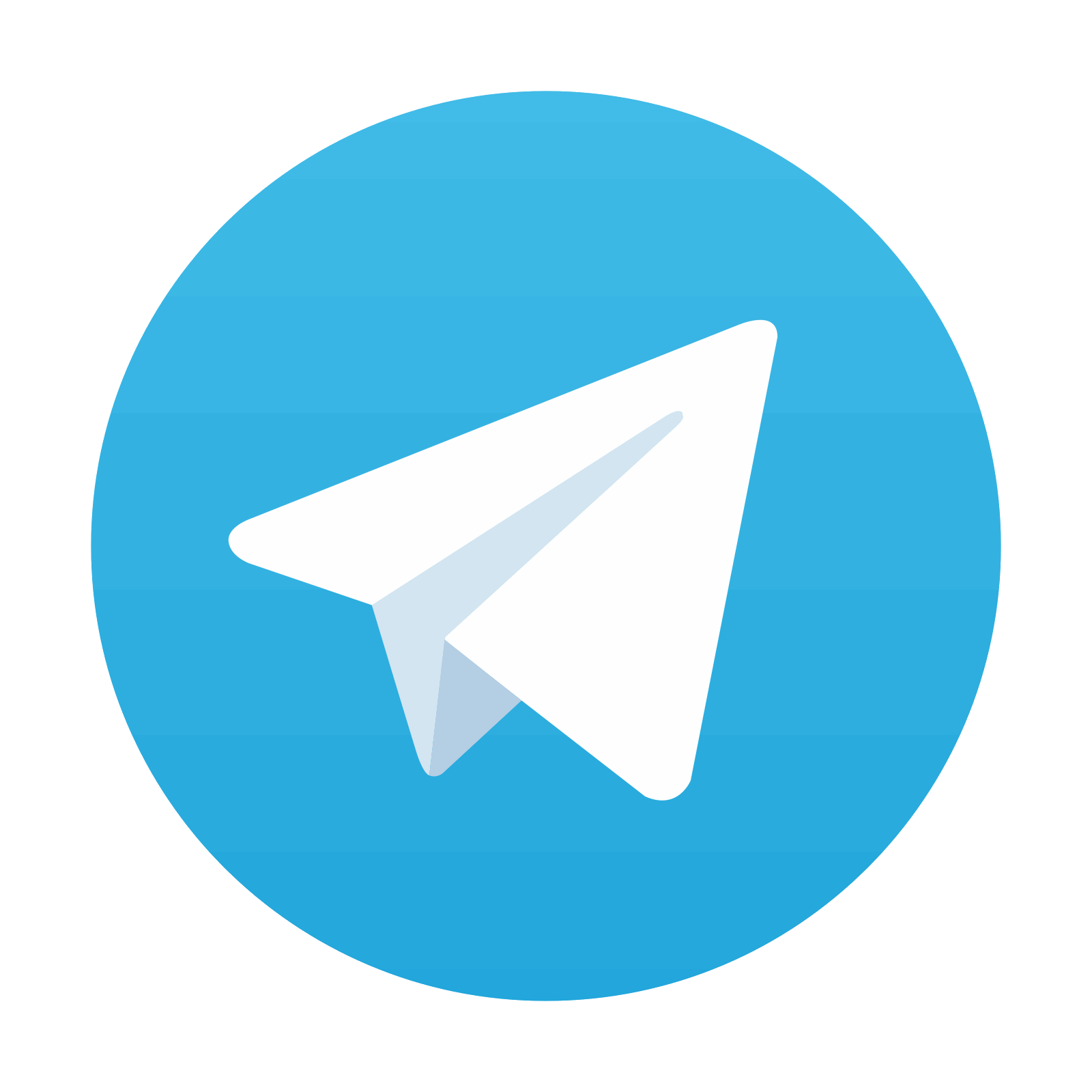
Stay updated, free dental videos. Join our Telegram channel

VIDEdental - Online dental courses
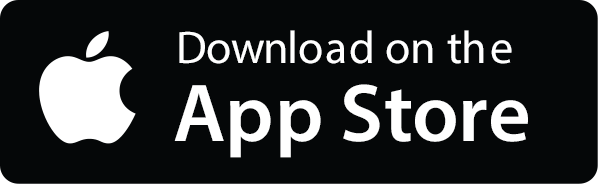
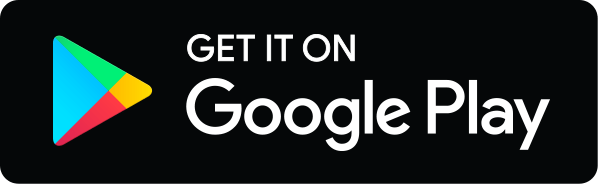