Abstract
Objective
The purpose of this work was the study of the effect of food/oral simulating liquids on the dynamic mechanical thermal properties (viscoelastic properties) of current commercial dental light-cured resin composites characterized as nanohybrids. These nanohybrids were Grandio, Protofill-nano and Tetric EvoCeram.
Methods
The properties were determined under dry conditions (1 h at 37 °C after light-curing) and also after storage in dry air, distilled water, artificial saliva SAGF ® or ethanol/water solution (75 vol%) at 37 °C for up 1, 7, 30 or 90 days. Dynamic mechanical thermal analysis tests were performed on a Diamond Dynamic Mechanical Analyzer in bending mode. A frequency of 1 Hz and a temperature range of 25–185 °C were applied, while the heating rate of 2 °C/min was selected to cover mouth temperature and the materials’ likely T g .
Results
Storage modulus, loss modulus and tangent delta were plotted against temperature over this period. The T g of composites was obtained as the temperature indicated by tan δ peak. Moreover, the maximum height of tan δ peak, the width at the half of tan δ maximum and a parameter known as “ ζ ” parameter were determined. All composites analyzed 1 h after light-curing and 1 day in air or in food/oral simulating liquids showed two T g . All composites stored for 7, 30 or 90 days in any medium showed unique T g value. Also among the various properties studied the most sensible in the structural changes of composites seems to be the T g .
Significance
Storage of composites in dry air at 37 °C which is very close to their T g (40 °C) for 1 or 7 days caused post curing reactions, while storage for 30 or 90 days has no further effect on composites. Storage in water or artificial saliva 37 °C for 1 or 7 days caused post curing reactions, while storage for 30 or 90 days seems to cause plasticization effect affecting some parameters analogously. Storage in ethanol/water solution (75 vol%) 37 °C for 1 or 7 days caused also post curing reactions, while storage for 30 or 90 days caused plasticization and/or probable oxidation/hydrolysis of polymeric network.
1
Introduction
Hybrids composites in general were marketed in early 1980s and the ongoing effort for smaller particles with greater resistance resulted in nanohybrids in 2000s. Nanohybrid composites were developed from microhybrid composites by the use of nanofillers, a step which led to a significant increase in the filler content of the materials and a considerable improvement in their physical properties . In that way the inorganic net is enhanced by tiny spheres which occupy the free space among the larger microparticles giving out a compact material. Very promising clinical data for these nanohybrid composites have become available for the demanding posterior region as well .
The food/oral simulating liquids have been recommended by the Bureau of Food, FDA. Some of them do exist in the mouth, others can simulate its conditions (ingredients, pH, viscosity). Their use enables us to see a fast wear of the materials in short time and furthermore to take in consideration processes like chemical affinity, elution or bonding. It goes without saying that physical/mechanical properties are influenced profoundly by the presence of the FSLs . The major influence regards the organic phase of the composites which can be affected chemically, since pure fillers are rather inert toward solvents and solutions. There are two main environments than one would like to imitate: the usual mouth condition (water, saliva) and the presence of foods/drinks (oils, alcohol, sugar, salt, etc.) . Apparently, the received food remains only for a while into the mouth where it is masticated. Long-time stays result in intensive erosions, something that we use to reveal the causes of the phenomena.
Recently we studied the dynamic mechanical thermal properties of two nanofilled resin composites after curing and after immersion in some food/oral simulating liquids for a certain time . Dental composites exhibit viscoelastic behavior due to the presence of polymer matrix, which is a viscoelastic material. Dynamic tests such as dynamic mechanical analysis (DMA) on the other hand are particular well suited for viscoelastic materials, since they determine both the elastic and viscous response of material. Also dynamic tests better mimic the cyclic masticatory loading to which dental composites are clinically subjected . The viscoelastic properties of several contemporary dental resins have been studied by using DMA .
This work aimed at the study of dynamic mechanical thermal properties of three current commercial dental light-cured resin nanohybrids. The nanohybrids studied are Grandio (GR), Protofill-nano (PR) and Tetric EvoCeram (TEC). They have different structural characteristics (content and structure of organic matrix and filler) and it was found interesting to study the effect of these characteristics on dynamic mechanical properties after photo-polymerization and after storage in some food/oral simulating liquids.
2
Materials and methods
2.1
Materials
Three commercially available light-cured nanohybrid composites were studied; TEC (Ivoclar-Vivadent, Schaan, Liechtenstein), GR (VOCO, Cuxhaven, Germany) and PR (Germany). Their specifications are listed in Table 1 .
Composite | Lot No. | Shade | Organic matrix | Filler | Total inorganic filler content |
---|---|---|---|---|---|
Grandio ( GR ) | 0809229 | A 3,5 | Bis-GMA, TEGDMA 13 wt% |
Silica: 20–60 nm (spherical) Barium-aluminaborosilicate: 0.1–2.5 μm mean 1000 nm |
87.0 wt%; 71.4 vol% |
Protofill-nano ( PR ) | 8W8354 | A 3 | Bis-GMA, TEGDMA, UDMA 18 wt% |
Strontium aluminum borosilicate: 0.6 μm SiO 2 nanoparticles: 20 nm |
81.9 wt% |
Tetric EvoCeram ( TEC ) | M29389 | A 3 | Bis-GMA, UDMA 17–18 wt% |
Barium glass, spherical mixed oxide, YbF 3 prepolymers (82–84 wt%) 40–3000 nm, mean 550 nm |
75–76 wt%; 53–55 vol% |
2.1.1
Artificial saliva
The SAGF ® medium was chosen as artificial saliva in this work and its composition is given in Table 2 . The pH of the SAGF medium was adjusted to 6.8, because this value was closer to that of the human saliva in the mouth. The artificial saliva and the samples were sterilized together to avoid colonization of microorganisms. The use of the SAGF liquid required special care because the solution was super-saturated in carbon dioxide with regard to the air. As a result it tended to lose CO 2 gas, which led to pH increase. Before each experiment the pH of solution was controlled and in case of rise drops of CH 3 COOH solution were added. All the above reactants were used as received.
Components | Concentration (mg L −1 ) |
---|---|
NaCl | 125.6 |
KCl | 963.9 |
KSCN | 189.2 |
KH 2 PO 4 | 654.5 |
Urea | 200.0 |
NaSO 4 ·10H 2 O | 763.2 |
NH 4 Cl | 178.0 |
CaCl 2 ·2H 2 O | 227.8 |
NaHCO 3 | 630.8 |
2.2
Preparation of specimens
For DMA tests, bar specimens of rectangular geometry were prepared by filling a Teflon mold (2 mm × 2 mm × 40 mm, as recommended by DMA manufacturer’s instructions) with unpolymerized material, taking care to minimize entrapped air. The upper and lower surface of the mold was overlaid with glass slides covered with a mylar sheet (thickness 0.05 mm) to avoid adhesion with the uncured material. The completed assembly was held together with spring clips and irradiated by overlapping, using a XL 3000 dental photocuring unit (3 M Company, St Paul, USA). This source consisted of a 75 W tungsten halogen lamp, which emits radiation between 420 and 500 nm and lighting power at 200 mW/cm 2 measured by Hilux curing light meter (Benlioglu Dental INC, Serial No. 9080935). This unit was used without the light guide in direct contact with the glass slides. The samples were irradiated for 60 s on each side applying overlaps. The assembly was dismantled, the composite was carefully removed by flexing the mold and the specimens were then slightly scratched to remove the material excess.
2.3
Dynamic mechanical analysis (DMA)
DMA is a powerful technique where a sinusoidal stress is applied and the resultant strain is measured. The properties measured under this oscillating loading are storage or Young modulus ( E ′), loss or viscous modulus ( E ″) and tan δ (ratio of loss to storage modulus). The storage modulus (the in-phase component of the modulus) represents the stiffness of a viscoelastic material and is proportional to the energy stored during a loading cycle. The loss modulus (the out of phase component) is related to the amount of energy lost due to viscous flow. The tan δ is also the tangent of the phase angle and is called damping. Damping is a dimensionless property and also a measure of how well the material can disperse (absorb or emit) energy throughout its mass. A high tan δ indicates high molecular mobility, while a low tan δ indicates less mobility into the material. As temperature increases and the material approaches the rubbery state, the tan δ value and the molecular mobility increases. The tan δ value goes up to a maximum as the polymer undergoes the transition from the glassy to the rubbery state. The glass transition temperature ( T g ) can be determined as the position of the maximum on the tan δ vs. temperature plot.
The bar-shaped specimens used for DMA measurements divided into groups of three samples each. The first group consisted of dry samples measured 1 h after curing. During this first hour they were placed in a desiccator at 37 ± 1 °C. The other groups consisted of samples which had been stored in dry air, distilled water, ethanol/water solution (75 vol%), or artificial saliva SAGF at 37 ± 1 °C for 1, 7, 30 or 90 days-time.
DMA tests were performed on a Diamond DMA dynamic mechanical analyzer (Perkin-Elmer) in bending mode. A frequency of 1 Hz was applied (approximately average chewing rate), bending force of 4000 mN and amplitude of 10 μm. A temperature range of 25–185 °C and a heating rate of 2 °C/min were selected to cover mouth temperature and the materials’ likely glass-transition temperature ( T g ). Storage modulus ( E ′), loss modulus ( E ″) and tangent delta (tan δ ) were plotted against temperature over this period. After the DMA run was completed, the sample was allowed to cool naturally at room temperature and the values of E ′, E ″ at 37 °C and the T g were noted. Then the process was repeated with the same specimen and the re-run values for E ′, E ″ and T g were determined as well. This method was used for each of the samples and the mean values were calculated.
2.4
Statistical analysis
The values reported in all following Tables represent mean values ± standard deviation of three replicates. One-way analysis of variance (ANOVA) test, followed by a Tukey’s test, for multiple comparisons between means to determinate significant differences was used at a significance level set at p ≤ 0.05, for analysis of the results (software package OriginPro 8, by OriginLab Corporation run on Microsoft Windows).
2
Materials and methods
2.1
Materials
Three commercially available light-cured nanohybrid composites were studied; TEC (Ivoclar-Vivadent, Schaan, Liechtenstein), GR (VOCO, Cuxhaven, Germany) and PR (Germany). Their specifications are listed in Table 1 .
Composite | Lot No. | Shade | Organic matrix | Filler | Total inorganic filler content |
---|---|---|---|---|---|
Grandio ( GR ) | 0809229 | A 3,5 | Bis-GMA, TEGDMA 13 wt% |
Silica: 20–60 nm (spherical) Barium-aluminaborosilicate: 0.1–2.5 μm mean 1000 nm |
87.0 wt%; 71.4 vol% |
Protofill-nano ( PR ) | 8W8354 | A 3 | Bis-GMA, TEGDMA, UDMA 18 wt% |
Strontium aluminum borosilicate: 0.6 μm SiO 2 nanoparticles: 20 nm |
81.9 wt% |
Tetric EvoCeram ( TEC ) | M29389 | A 3 | Bis-GMA, UDMA 17–18 wt% |
Barium glass, spherical mixed oxide, YbF 3 prepolymers (82–84 wt%) 40–3000 nm, mean 550 nm |
75–76 wt%; 53–55 vol% |
2.1.1
Artificial saliva
The SAGF ® medium was chosen as artificial saliva in this work and its composition is given in Table 2 . The pH of the SAGF medium was adjusted to 6.8, because this value was closer to that of the human saliva in the mouth. The artificial saliva and the samples were sterilized together to avoid colonization of microorganisms. The use of the SAGF liquid required special care because the solution was super-saturated in carbon dioxide with regard to the air. As a result it tended to lose CO 2 gas, which led to pH increase. Before each experiment the pH of solution was controlled and in case of rise drops of CH 3 COOH solution were added. All the above reactants were used as received.
Components | Concentration (mg L −1 ) |
---|---|
NaCl | 125.6 |
KCl | 963.9 |
KSCN | 189.2 |
KH 2 PO 4 | 654.5 |
Urea | 200.0 |
NaSO 4 ·10H 2 O | 763.2 |
NH 4 Cl | 178.0 |
CaCl 2 ·2H 2 O | 227.8 |
NaHCO 3 | 630.8 |
2.2
Preparation of specimens
For DMA tests, bar specimens of rectangular geometry were prepared by filling a Teflon mold (2 mm × 2 mm × 40 mm, as recommended by DMA manufacturer’s instructions) with unpolymerized material, taking care to minimize entrapped air. The upper and lower surface of the mold was overlaid with glass slides covered with a mylar sheet (thickness 0.05 mm) to avoid adhesion with the uncured material. The completed assembly was held together with spring clips and irradiated by overlapping, using a XL 3000 dental photocuring unit (3 M Company, St Paul, USA). This source consisted of a 75 W tungsten halogen lamp, which emits radiation between 420 and 500 nm and lighting power at 200 mW/cm 2 measured by Hilux curing light meter (Benlioglu Dental INC, Serial No. 9080935). This unit was used without the light guide in direct contact with the glass slides. The samples were irradiated for 60 s on each side applying overlaps. The assembly was dismantled, the composite was carefully removed by flexing the mold and the specimens were then slightly scratched to remove the material excess.
2.3
Dynamic mechanical analysis (DMA)
DMA is a powerful technique where a sinusoidal stress is applied and the resultant strain is measured. The properties measured under this oscillating loading are storage or Young modulus ( E ′), loss or viscous modulus ( E ″) and tan δ (ratio of loss to storage modulus). The storage modulus (the in-phase component of the modulus) represents the stiffness of a viscoelastic material and is proportional to the energy stored during a loading cycle. The loss modulus (the out of phase component) is related to the amount of energy lost due to viscous flow. The tan δ is also the tangent of the phase angle and is called damping. Damping is a dimensionless property and also a measure of how well the material can disperse (absorb or emit) energy throughout its mass. A high tan δ indicates high molecular mobility, while a low tan δ indicates less mobility into the material. As temperature increases and the material approaches the rubbery state, the tan δ value and the molecular mobility increases. The tan δ value goes up to a maximum as the polymer undergoes the transition from the glassy to the rubbery state. The glass transition temperature ( T g ) can be determined as the position of the maximum on the tan δ vs. temperature plot.
The bar-shaped specimens used for DMA measurements divided into groups of three samples each. The first group consisted of dry samples measured 1 h after curing. During this first hour they were placed in a desiccator at 37 ± 1 °C. The other groups consisted of samples which had been stored in dry air, distilled water, ethanol/water solution (75 vol%), or artificial saliva SAGF at 37 ± 1 °C for 1, 7, 30 or 90 days-time.
DMA tests were performed on a Diamond DMA dynamic mechanical analyzer (Perkin-Elmer) in bending mode. A frequency of 1 Hz was applied (approximately average chewing rate), bending force of 4000 mN and amplitude of 10 μm. A temperature range of 25–185 °C and a heating rate of 2 °C/min were selected to cover mouth temperature and the materials’ likely glass-transition temperature ( T g ). Storage modulus ( E ′), loss modulus ( E ″) and tangent delta (tan δ ) were plotted against temperature over this period. After the DMA run was completed, the sample was allowed to cool naturally at room temperature and the values of E ′, E ″ at 37 °C and the T g were noted. Then the process was repeated with the same specimen and the re-run values for E ′, E ″ and T g were determined as well. This method was used for each of the samples and the mean values were calculated.
2.4
Statistical analysis
The values reported in all following Tables represent mean values ± standard deviation of three replicates. One-way analysis of variance (ANOVA) test, followed by a Tukey’s test, for multiple comparisons between means to determinate significant differences was used at a significance level set at p ≤ 0.05, for analysis of the results (software package OriginPro 8, by OriginLab Corporation run on Microsoft Windows).
3
Results
In Fig. 1 (a–c) the storage modulus, loss modulus and tan δ , versus temperature curves are demonstrated correspondingly for TEC material, as representative. The sample has been kept for 1 h at 37 °C before the record. After the first scan the specimen was cooled down to 25 °C and a second scan was recorded from 25 to 185 °C. The curves obtained from the second run are shown also in Fig. 1 (a–c). The double decrease of elastic modulus observed during the 1st run became a single decrease and the values of elastic modulus recorded during the 2nd run were higher than the corresponding ones recorded during the first run. The values of viscous modulus in the second run remained almost the same. The tan δ peak at low temperature was disappeared, while that at higher temperature remained also the same. These differences in the storage modulus and tan δ curves during the 1st and 2nd run confirmed the fact that additional curing reaction took place during the first run. When light-curing at room temperature is over, unreacted vinyl bonds are left behind. The lifetime of residual free radical at room temperature could reach several days or months, depending on crosslinking density and storage conditions . The existence of the residual groups indicates that further reactions may take place during any kind of heating, during a thermo-mechanical test or storing isothermally even at low temperatures (e.g. body temperature).
Each of the two maxima in the tan δ versus temperature curves corresponds to one of the two drops in the storage modulus curves and represents the two T g values for the dry samples. The first T g is the apparent one of the light-cured specimen, which still contains unreacted methacrylate C C bonds within the vitrified network, whereas the second T g is due to the specimen cured during the DMA test, as said before. Two T g values were also observed in the tan δ curve of homopolymer resins and in commercial light-cured composites. In all these cases the two T g values were attributed to the additional thermal curing occurring.
It is reported that the increase of filler particles in the composite’s composition introduces a broadening of the tan δ curve . It has been also shown that the width of tan δ curve is directly associated with the heterogeneity of polymer network formed by photopolymerization of dimethacrylates . A more homogeneous network presents a narrower tan δ peak. The heterogeneity of a polymer network can be evaluated quantitatively, by examining the width of the tan δ peak at half of its height.
The structural heterogeneity of a polymer network formed by photopolymerization of dimethacrylates can be expressed qualitatively by the so called ‘ ζ ’ parameter. The values of storage modulus of the material drop uniformly with the temperature increase, until they reach a plateau (rubbery region) ( Fig. 2 ). The inverse ratio of the modulus in the rubbery region (rubbery modulus) to temperature at which the modulus was measured was called ‘ ζ ’ parameter and was generally considered to be inversely correlated to the crosslink density of the polymer network. Higher ‘ ζ ’ values correspond to lower crosslink density .
Table 3 summarizes the initial behavior of the composites, shortly after curing. The effect of composites storage in dry air, distilled water, artificial saliva SAGF or ethanol/water solution (75 vol%), at 37 ± 1 °C for up 1, 7, 30 or 90 days on their viscoelastic properties is shown correspondingly in Tables 4–7 .
Property | GR | PR | TEC |
---|---|---|---|
E ′ (GPa) at 37 °C | 10.99 ± 2.78 | 5.31 ± 0.07 | 6.30 ± 0.32 |
E ″ (GPa) at 37 °C | 0.95 ± 0.15 | 0.86 ± 0.01 | 0.68 ± 0.06 |
tan δ (×10 3 ) at 37 °C | 92 ± 3 | 161 ± 1 | 110 ± 8 |
T g (°C) | 40.5 ± 1.5 a | 41.7 ± 0.3 a | 40.1 ± 2.1 a |
137.0 ± 4.9 a | 134.9 ± 1.6 a | 113.9 ± 9.6 | |
Δ T a (°C) | 30.5 ± 2.0 a | 27.6 ± 0.9 | 30.4 ± 6.1 a |
44.7 ± 4.0 | 32.8 ± 0.6 a | 30.4 ± 1.7 a | |
h max (×10 3 ) b | 111 ± 5 | 168 ± 5 | 217 ± 9 |
92 ± 5 | 135 ± 1 | 176 ± 8 | |
“ ζ ” parameter (×10 7 ) (K/Pa) c | 0.87 ± 0.03 | 2.34 ± 0.10 | 10.45 ± 0.19 |
a Width of the tan δ curve at half peak maximum.
Composites | E ′ (GPa) | E ″ (GPa) | tan δ (×10 3 ) | T g (°C) | Δ T (°C) | h max (×10 3 ) | ζ ×10 7 (K/Pa) | |
---|---|---|---|---|---|---|---|---|
GR | 1 h/air | 10.99 ± 2.78 a | 0.95 ± 0.15 a | 92 ± 3 a | 40.5 ± 1.5 a | 30.5 ± 2.0 a | 111 ± 5 a | 1.07 ± 0.03 a |
137.0 ± 4.9 | 44.7 ± 4.0 | 92 ± 5 | ||||||
1d | 11.44 ± 0.81 a | 0.74 ± 0.01 b | 65 ± 3 b | 79.9 ± 0.0 b | 24.5 ± 3.4 a | 113 ± 4 a | 1.04 ± 0.00 a | |
136.2 + 3.7 | 43.5 ± 4.0 | 92 ± 5 | ||||||
7d | 11.90 ± 2.00 a | 0.77 ± 0.09 b | 65 ± 7 b | 124.4 ± 0.4 c | 106.9 ± 2.8 b | 119 ± 9 a | 1.10 ± 0.01 a | |
30d | 10.30 ± 2.57 a | 0.67 ± 0.05 c | 68 ± 3 b | 125.9 ± 0.1 c | 94.1 ± 3.1 b | 118 ± 8 a | 1.43 ± 0.44 a | |
90d | 10.95 ± 2.38 a | 0.63 ± 0.13 c | 57 ± 3 b | 126.0 ± 1.4 c | 101.5 ± 3.5 b | 108 ± 6 a | 1.17 ± 0.09 a | |
PR | 1 h/air | 5.31 ± 0.07 a | 0.86 ± 0.01 a | 161 ± 1 a | 41.7 ± 0.3 a | 27.6 ± 0.9 a | 168 ± 5 a | 3.24 ± 0.10 a |
134.9 ± 1.6 | 32.8 ± 0.6 | 135 ± 1 | ||||||
1d | 5.38 ± 0.17 a | 0.58 ± 0.11 b | 108 ± 10 b | 78.7 ± 1.0 b | 21.2 ± 0.9 a | 174 ± 3 a | 3.96 ± 0.80 a | |
128.1 ± 3.9 | 20.4 ± 3.7 | 176 ± 6 | ||||||
7d | 6.25 ± 0.58 b | 0.57 ± 0.04 b | 94 ± 3 b | 111.5 ± 1.8 c | 54.9 ± 5.4 b | 184 ± 9 b | 3.15 ± 0.39 a | |
30d | 6.48 ± 0.94 b | 0.56 ± 0.10 b | 79 ± 5 c | 108.2 ± 1.2 c | 57.7 ± 0.3 b | 183 ± 1 b | 3.23 ± 0.10 a | |
90d | 6.49 ± 0.00 b | 0.56 ± 0.17 b | 82 ± 7 c | 105.3 ± 0.9 c | 55.6 ± 1.1 b | 180 ± 3 b | 3.90 ± 0.09 a | |
TEC | 1 h/air | 6.30 ± 0.32 a | 0.68 ± 0.06 a | 110 ± 8 a | 40.1 ± 2.1 a | 30.4 ± 6.1 a | 217 ± 9 a | 5.45 ± 0.19 a |
113.9 ± 9.6 | 30.4 ± 1.7 | 176 ± 8 | ||||||
1d | 8.11 ± 0.63 b | 0.59 ± 0.07 a,b | 73 ± 5 b | 84.8 ± 2.8 b | 23.3 ± 3.0 a | 213 ± 6 a | 4.26 ± 0.09 a | |
112.2 ± 0.6 | 21.1 ± 1.4 | 177 ± 0 | ||||||
7d | 7.90 ± 1.05 b | 0.51 ± 0.07 b | 64 ± 5 b | 92.5 ± 2.2 c | 60.5 ± 3.5 b | 211 ± 5 a | 4.84 ± 0.07 a | |
30d | 7.97 ± 0.78 b | 0.49 ± 0.2 b | 64 ± 10 b | 95.5 ± 0.4 c | 51.3 ± 1.4 b | 222 ± 4 a | 5.33 ± 0.62 a | |
90d | 7.73 ± 1.09 b | 0.50 ± 0.04 b | 67 ± 10 b | 96.0 ± 0.1 c | 52.7 ± 3.1 b | 212 ± 5 a | 5.09 ± 0.53 a |
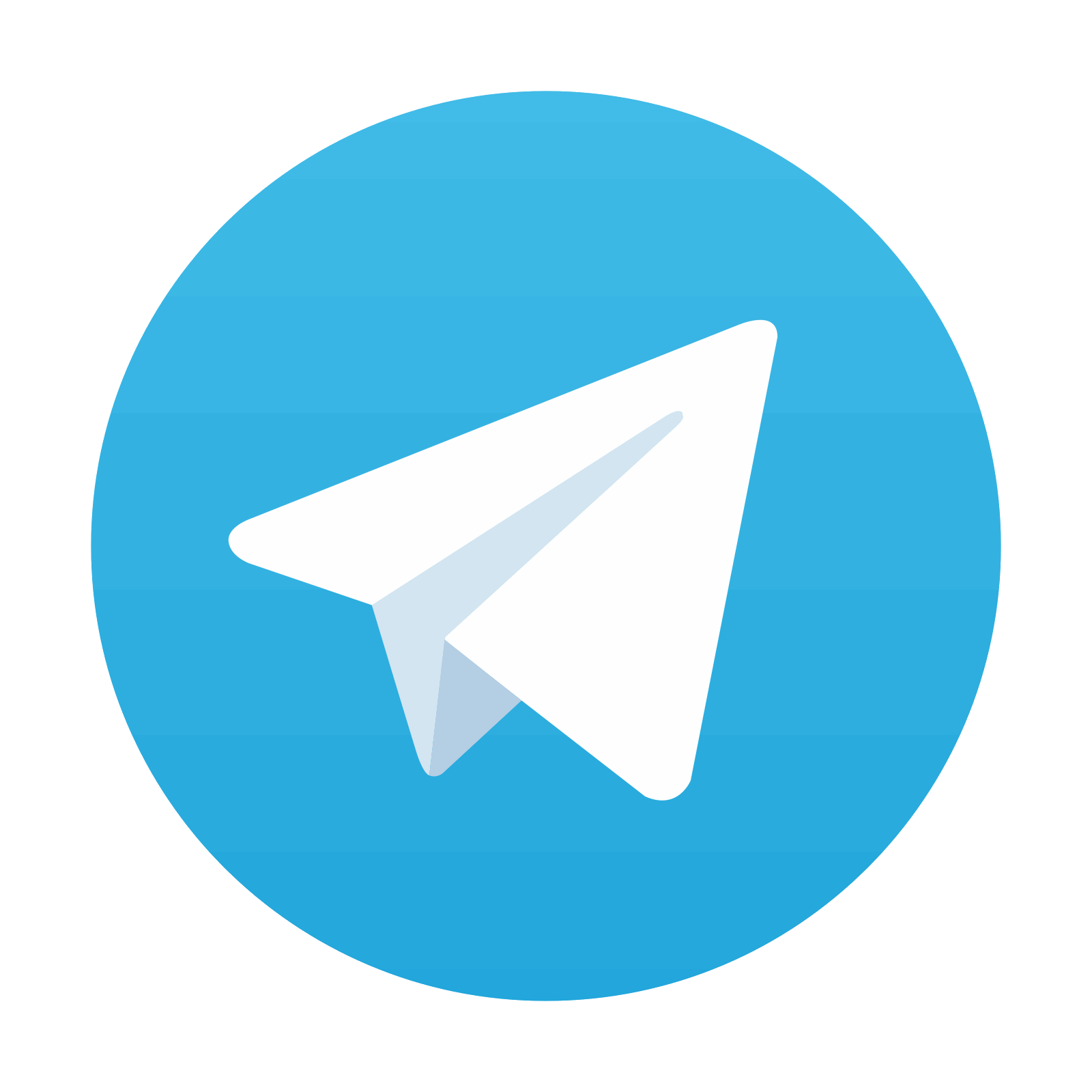
Stay updated, free dental videos. Join our Telegram channel

VIDEdental - Online dental courses
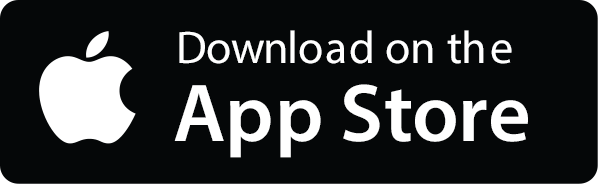
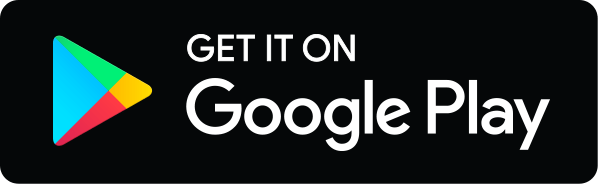
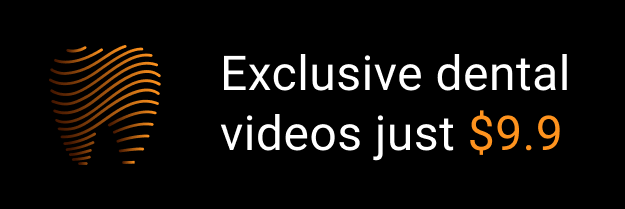