Abstract
Objective
To evaluate the effect of two aging methods (mechanical cycling and autoclave) on the mechanical behavior of veneer and framework ceramic specimens with different configurations (monolithic, two and three-layers).
Methods
Three ceramics used as framework for fixed dental prostheses (YZ—Vita In-Ceram YZ; IZ—Vita In-Ceram Zirconia; AL—Vita In-Ceram AL) and two veneering porcelains (VM7 and VM9) were studied. Bar-shaped specimens were produced in three different designs: monolithic, two layers (porcelain–framework) and three layers (porcelain–framework–porcelain). Specimens were tested for three-point flexural strength at 1 MPa/s in 37 °C artificial saliva. Three different experimental conditions were evaluated (n = 10): control; mechanical cycling (2 Hz, 37 °C artificial saliva); and autoclave aging (134 °C, 2 bars, 5 h). Bi-layered specimens were tested in both conditions: with porcelain or framework ceramic under tension. Fracture surfaces were analyzed using stereomicroscope and scanning electron microscopy. Results were statistically analyzed using Kruskal–Wallis and Student-Newman–Keuls tests.
Results
Only for AL group, mechanical cycling and autoclave aging significantly decreased the flexural strength values in comparison to the control (p < 0.01). YZ, AL, VM7 and VM9 monolithic groups showed no strength degradation. For multi-layered specimens, when the porcelain layer was tested in tension (bi and tri-layers), the aging methods evaluated also had no effect on strength (p ≥ 0.05). Total and partial failure modes were identified.
Significance
Mechanical cycling and autoclave aging protocols had no effect on the flexural strength values and failure behavior of YZ and IZ ceramic structures. Yet, AL monolithic structures showed a significant decrease in flexural strength with any of the aging methods.
1
Introduction
An important challenge in the Dental Materials is to find a material that is able to successfully replace the metal framework in prosthetic restorations. Among the existent prosthetic systems, the metal-ceramic is still considered the gold standard for fixed dental prostheses (FDPs) as it exhibits success rates varying from 72 to 87% after 10 years, 69 to 74% after 15 years, and 53% after 30 years . A survival rate of 94.4% after 5 years and an annual failure rate of 1.2% were estimated for metal-ceramic FDPs showing a superior clinical behavior than all-ceramic FDPs .
The need for replacement of metal-ceramic restorations is mostly related to its inferior biocompatibility and low translucency in comparison to all-ceramic prostheses. In order to withstand the high stress concentration induced in posterior areas of the oral cavity, high-crystalline content alumina and zirconia-based ceramics were introduced in Dentistry. The production of FDPs with these ceramic systems involves manufacturing of a high-toughness ceramic framework that is later veneered with a glass-based ceramic to provide esthetics, resulting in a multi-layer all-ceramic restoration .
Ceramics such as polycrystalline alumina, alumina-based glass-infiltrated zirconia-reinforced ceramic (In-Ceram Zirconia) and yttrium oxide partially-stabilized tetragonal zirconia polycrystal (Y-TZP) are all indicated to produce frameworks for posterior multi-unit FDPs . Clinical studies showed relatively high success rates for these ceramic systems; however the follow-up periods are still relatively short, no longer than 6 years . A study that followed up three to five-unit Y-TZP FDPs for a longer period (up to 10 years) reported a survival rate of 65% for FDPs and 91.5% for zirconia frameworks . The most frequently technical failures reported in these studies were fracture of the framework (connector), veneer chipping and marginal discoloration .
Especially for Y-TZP, the scientific community showed some concern regarding its clinical prognosis due to the lack of long-term clinical studies with FDPs and high failure rates reported in the Orthopedic field for femoral heads . The superior mechanical behavior observed for Y-TZP is associated to the transformation toughening mechanism. A tetragonal to monoclinic zirconia phase transformation is induced around pre-existing microstructural flaws when stress concentration occurs at the crack tip. The phase transformation is followed by a volumetric expansion (3–5%) resulting in compressive stresses around the flaw, which increases the fracture toughness of the ceramic material. On the other hand, in the presence of humidity and low temperature, a spontaneous phase transformation may be triggered in the microstructure of Y-TZP. The crystal volumetric expansion results in localized stresses and micro-cracking in the material surface. These surface cracks allow water to further penetrate in the interior of the material, leading to additional phase transformation and resulting in the degradation of the mechanical properties (low temperature degradation, LTD) .
Ceramics are also susceptible to another degradation phenomenon called subcritical crack growth (SCG), which is characterized by the stable growth under stress of pre-existing flaws until a critical size is reached, leading to catastrophic failure. SCG is influenced by the loading condition, pH, temperature fluctuations, composition of the immersion solution and, for Y-TZP, grain size and yttria concentration . Some loading types, such as the one applied cyclically during mastication at low intensity, trigger the SCG phenomenon in ceramic materials . Thus, clinically, ceramics fail under a stress level well below the ones reported in fast fracture in vitro tests. Therefore, laboratory tests should consider the configuration (multi-layer) of all-ceramic prosthesis and the fatigue process associated to cyclic loading, humidity and temperature variations so as to produce clinically relevant data. Aging methods could involve cyclic loading in a humid environment—with or without thermal cycling, storage in distilled water, and autoclaving .
Therefore, the objective of this study was to evaluate the effect of two aging methods (mechanical cycling and autoclave) on the flexural strength and failure mode of ceramic specimens with different configurations: monolithic and multilayer (two and three ceramic layers). The study hypothesis is that mechanical cycling and autoclave aging influence the flexural strength values and failure behavior of ceramic structures.
2
Materials and methods
Three ceramics used as framework materials for FDPs and two veneering porcelains were studied ( Table 1 ). Three bar-shaped specimen (2 mm × 4 mm × 16 mm) designs were produced:
- (1)
Monolithic (one material, either veneering porcelain or framework material);
- (2)
Two layers (1-mm thick framework ceramic and 1-mm thick of porcelain);
- (3)
Three layers (1-mm thick framework ceramic, veneered on all sides with a 0.5-mm thick porcelain).
Infrastructure (IS) a | IS composition | Veneer a | Veneer composition |
---|---|---|---|
(YZ) Vita In-Ceram YZ | Yttria partially stabilized tetragonal zirconia polycrystal | (VM9) Vita VM9 | Feldsphatic porcelain |
(IZ) Vita In-Ceram Zirconia | Alumina-based zirconia-reinforced glass infiltrated ceramic | (VM7) Vita VM7 | Feldsphatic porcelain |
(AL) Vita In-Ceram AL | Alumina polycrystal | (VM7) Vita VM7 | Feldsphatic porcelain |
YZ, IZ and AL bar-shaped specimens were obtained by cutting pre-sintered CAD-CAM blocks using a diamond disc in a precision cutting machine (Isomet 1000, Buehler, Lake Bluff, USA) at 275 rpm. YZ and AL specimens were sintered (1530 °C) in the Zyrcomat furnace (Vita Zahnfabrik, Germany). IZ material was infiltrated with glass (Zirconia Glass Powder, Vita Zahnfabrik, Germany) following the manufacturer instructions (1110 °C for 6 h) and the excess glass was removed with diamond burs. VM7 and VM9 specimens were fabricated by mixing the ceramic powder with distilled water to form a slurry that was poured into a metallic mold and condensed with manual vibration. The Keramat I furnace (Knebel, Porto Alegre, Brazil) was used to perform the porcelain sintering. Porcelain specimens were sintered according to the following cycle: pre-drying at 500 °C for 6 min, heating to 910 °C at a rate of 55 °C/min under vacuum, heating at 960 °C for 1 min and cooled down to room temperature (6 min).
Monolithic specimens were ground to their final dimensions (2 mm × 4 mm × 16 mm), and the 4-mm wide faces were polished to a 1 μm finish using a polishing machine (Ecomet 2, Buehler, Lake Bluff, USA). All edges were chamfered to a 0.1-mm wide chamfer, as recommended by the ISO 6872:2008 standard . For multi-layer structures (two- and three-layer specimens), the framework material was veneered with the porcelain recommended by the manufacturer ( Table 1 ). Framework bars were produced following the methodology described for monolithic specimens (above). For two-layer structures, the framework (1 mm × 4 mm × 16 mm) was veneered with a 1-mm thick layer of porcelain using a metallic mold. After sintering, ten specimens had the framework surface polished and ten specimens had the porcelain surface polished. For tri-layered structures, frameworks with the dimensions of 1 mm × 3 mm × 15 mm were produced and all surfaces were veneered with a 0.5 mm thickness of porcelain. One of the 4-mm wide faces was polished to a 1-μm finish. After polishing, all edges were chamfered . A bonding agent (Effect Bonder, Vita Zahnfabrik, Germany) was applied to the YZ specimen surfaces before veneering. All specimens were sintered according to the manufacturer’s instructions.
Forty two experimental groups were evaluated (n = 10) for flexural strength. Specimens were classified according to the number of layers (one, two or three layers) and flexural test configuration (porcelain or framework in tension) ( Table 2 ). In addition, three different aging conditions were evaluated:
- (1)
Control: Fast fracture test in a three-point flexural strength (σ) design;
- (2)
Mechanical cycling (M): Mechanical aging in a chewing simulator. Aging was performed in artificial saliva at 37 °C and the load was applied using a three-point flexure fixture, at 2 Hz frequency. Different load amplitude and number of cycles were chosen for the different materials and specimen configuration considering fast fracture and fatigue data obtained in previous studies . Yet, the aging profile should properly simulate the oral environment and induce degradation to the material in a reasonable period of time, without causing fracture during the process. Therefore, the following aging profiles were used: 80 N load and 10 5 cycles for monolithic framework specimens; 45 N load and 10 5 cycles for bi-layered specimens tested with the framework in tension; 45 N load and 10 4 cycles for monolithic porcelain specimens, bi-layered specimens tested with the porcelain in tension, and tri-layered specimens. After mechanical aging, specimens were tested in fast fracture;
- (3)
Autoclave aging (A): Accelerated aging was performed in autoclave (Phoenix AB 25, São Carlos, Brazil), at 134 °C, under 2 bars of pressure for 5 h (corresponding to 21 years at 37 °C in the human body ). Five 1-h cycles were performed to complete the aging process. After autoclave aging, specimens were tested in fast fracture.
Material | Monolithic | Two layers | Three layers | |
---|---|---|---|---|
Porcelain in tension | Infrastructure in tension | |||
YZ | YZ | YZ-PT | YZ-IT | YZ-TRI |
IZ | IZ | IZ-PT | IZ-IT | IZ-TRI |
AL | AL | AL-PT | AL-IT | AL-TRI |
VM7 | VM7 | |||
VM9 | VM9 |
The fast fracture mechanical test was performed according to the ISO 6872:2008 standard with a three-point flexure fixture with 2-mm diameter rollers and 12.0 mm span . The fixture was immersed in 37 °C artificial saliva with the following composition: 100 mL of KH 2 PO 4 (2.5 mM); 100 mL of Na 2 HPO 4 (2.4 mM); 100 mL of KHCO 3 (1.50 mM); 100 mL of NaCl (1.0 mM); 100 mL of MgCl 2 (0.15 mM); 100 mL of CaCl 2 (1.5 mM); and 6 mL of citric acid (0.002 mM) . The flexural strength (σ f ) was determined using a universal testing machine (Sintech 5G, MTS, São Paulo, Brazil) at a constant stress rate of 1 MPa/s. Three-point flexural strength (σ f ) values for monolithic, bi- and tri-layered specimens were calculated using equations and Young’s modulus (E) and Poisson’s ratio (ν) values reported previously .
Fracture surfaces were examined using stereomicroscope (Olympus SZ61, Olympus, Japan) and scanning electron microscope (SEM) (Stereoscan 440, LEO Electron Microscopy Ltd., Cambridge, England) to determine the mode of failure based on the fracture origin and fractographic principles . Data for σ f were analyzed using Kruskal–Wallis one-way analysis of variance on ranks and Student-Newman–Keuls method for multiple comparisons (α = 0.05).
2
Materials and methods
Three ceramics used as framework materials for FDPs and two veneering porcelains were studied ( Table 1 ). Three bar-shaped specimen (2 mm × 4 mm × 16 mm) designs were produced:
- (1)
Monolithic (one material, either veneering porcelain or framework material);
- (2)
Two layers (1-mm thick framework ceramic and 1-mm thick of porcelain);
- (3)
Three layers (1-mm thick framework ceramic, veneered on all sides with a 0.5-mm thick porcelain).
Infrastructure (IS) a | IS composition | Veneer a | Veneer composition |
---|---|---|---|
(YZ) Vita In-Ceram YZ | Yttria partially stabilized tetragonal zirconia polycrystal | (VM9) Vita VM9 | Feldsphatic porcelain |
(IZ) Vita In-Ceram Zirconia | Alumina-based zirconia-reinforced glass infiltrated ceramic | (VM7) Vita VM7 | Feldsphatic porcelain |
(AL) Vita In-Ceram AL | Alumina polycrystal | (VM7) Vita VM7 | Feldsphatic porcelain |
YZ, IZ and AL bar-shaped specimens were obtained by cutting pre-sintered CAD-CAM blocks using a diamond disc in a precision cutting machine (Isomet 1000, Buehler, Lake Bluff, USA) at 275 rpm. YZ and AL specimens were sintered (1530 °C) in the Zyrcomat furnace (Vita Zahnfabrik, Germany). IZ material was infiltrated with glass (Zirconia Glass Powder, Vita Zahnfabrik, Germany) following the manufacturer instructions (1110 °C for 6 h) and the excess glass was removed with diamond burs. VM7 and VM9 specimens were fabricated by mixing the ceramic powder with distilled water to form a slurry that was poured into a metallic mold and condensed with manual vibration. The Keramat I furnace (Knebel, Porto Alegre, Brazil) was used to perform the porcelain sintering. Porcelain specimens were sintered according to the following cycle: pre-drying at 500 °C for 6 min, heating to 910 °C at a rate of 55 °C/min under vacuum, heating at 960 °C for 1 min and cooled down to room temperature (6 min).
Monolithic specimens were ground to their final dimensions (2 mm × 4 mm × 16 mm), and the 4-mm wide faces were polished to a 1 μm finish using a polishing machine (Ecomet 2, Buehler, Lake Bluff, USA). All edges were chamfered to a 0.1-mm wide chamfer, as recommended by the ISO 6872:2008 standard . For multi-layer structures (two- and three-layer specimens), the framework material was veneered with the porcelain recommended by the manufacturer ( Table 1 ). Framework bars were produced following the methodology described for monolithic specimens (above). For two-layer structures, the framework (1 mm × 4 mm × 16 mm) was veneered with a 1-mm thick layer of porcelain using a metallic mold. After sintering, ten specimens had the framework surface polished and ten specimens had the porcelain surface polished. For tri-layered structures, frameworks with the dimensions of 1 mm × 3 mm × 15 mm were produced and all surfaces were veneered with a 0.5 mm thickness of porcelain. One of the 4-mm wide faces was polished to a 1-μm finish. After polishing, all edges were chamfered . A bonding agent (Effect Bonder, Vita Zahnfabrik, Germany) was applied to the YZ specimen surfaces before veneering. All specimens were sintered according to the manufacturer’s instructions.
Forty two experimental groups were evaluated (n = 10) for flexural strength. Specimens were classified according to the number of layers (one, two or three layers) and flexural test configuration (porcelain or framework in tension) ( Table 2 ). In addition, three different aging conditions were evaluated:
- (1)
Control: Fast fracture test in a three-point flexural strength (σ) design;
- (2)
Mechanical cycling (M): Mechanical aging in a chewing simulator. Aging was performed in artificial saliva at 37 °C and the load was applied using a three-point flexure fixture, at 2 Hz frequency. Different load amplitude and number of cycles were chosen for the different materials and specimen configuration considering fast fracture and fatigue data obtained in previous studies . Yet, the aging profile should properly simulate the oral environment and induce degradation to the material in a reasonable period of time, without causing fracture during the process. Therefore, the following aging profiles were used: 80 N load and 10 5 cycles for monolithic framework specimens; 45 N load and 10 5 cycles for bi-layered specimens tested with the framework in tension; 45 N load and 10 4 cycles for monolithic porcelain specimens, bi-layered specimens tested with the porcelain in tension, and tri-layered specimens. After mechanical aging, specimens were tested in fast fracture;
- (3)
Autoclave aging (A): Accelerated aging was performed in autoclave (Phoenix AB 25, São Carlos, Brazil), at 134 °C, under 2 bars of pressure for 5 h (corresponding to 21 years at 37 °C in the human body ). Five 1-h cycles were performed to complete the aging process. After autoclave aging, specimens were tested in fast fracture.
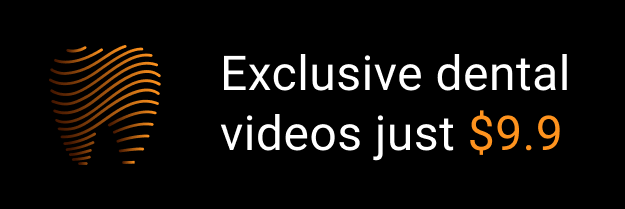