Abstract
Objective
To determine the nanohardness and elastic moduli of two bulk-fill resin based composites (RBCs) at increasing depths from the surface and increasing distances laterally from the center after light curing.
Methods
Two bulk-fill dental RBCs: Tetric EvoCeram Bulk Fill (TECBF) and Filtek Bulk Fill Flowable (FBFF) were light cured in a metal mold with a 6 mm diameter and a 10 mm long semi-circular notch. The RBCs were photo-polymerized for 10 s using a light emitting diode (LED) Bluephase Style curing light, with the original light probe that lacked the homogenizer. This light has two blue light and one violet light LED emitters. By changing the probe orientation over the mold, the light output from only two LEDs reached the RBC. Measurements were made using: (i) the light from one violet and one blue LED, and (ii) the light from the two blue LEDs. Five specimens of each RBC were made using each LED orientation (total 20 specimens). Specimens were then stored in the dark at 37 °C for 24 h. Fifty indents were made using an Agilent G200 nanoindentor down to 4 mm from the surface and 2.5 mm right and left of the centerline. The results were analyzed ( alpha = 0.05) using multiple paired-sample t -tests, ANOVA, Bonferroni post-hoc tests, and Pearson correlations.
Results
The elastic modulus and nanohardness varied according to the depth and the distance from the centerline. For TECBF, no significant difference was found between the spatial variations in the elastic modulus or hardness values when violet-blue or blue-blue LEDs were used. For FBFF, the elastic modulus and nanohardness on the side exposed to the violet emitter were significantly less than the side exposed to the blue emitter. A strong correlation between nanohardness and elastic modulus was found in all groups ( r 2 = 0.9512–0.9712).
Significance
Resin polymerization was not uniform throughout the RBC. The nanohardness and elastic modulus across two RBC materials were found to decline differently according to the orientation of the violet and blue light LED emitters within the curing light.
1
Introduction
When traditional resin based composite (RBC) materials are placed and light cured in 5 mm thick bulk increments, they exhibit lower levels of hardness at the surface that is furthest away from the light source . This occurs because increases in the RBC thickness cause an exponential decrease in the amount of light available to photo-cure the resin . With the recent introduction of bulk-fill RBCs, a greater depth of cure may be achieved. It is claimed that some brands of bulk-fill RBCs can be polymerized in up to 4-millimeter thick increments when light cured for 20 seconds using a high powered broad-spectrum curing light . This increase in depth of cure is achieved by using specific polymerization modulators, by improving the translucency, or by using more potent initiator systems . For example, the fillers in Tetric EvoCeram Bulk Fill (Ivoclar Vivadent, Schaan, Liechtenstein) are smaller in size and thus may increase the translucency of this RBC and enable improved polymerization at greater depths. In addition, Tetric EvoCeram Bulk Fill uses a Type I germanium based initiator system that has a higher photocuring activity than traditional initiators . These Type I photoinitiators undergo a unimolecular reaction upon irradiation and, consequently, they are more reactive to light compared to camphorquinone (CQ) and require a lower radiant exposure to adequately photo-cure the RBC . The depth of cure values may also be based upon the maximum length of hard RBC remaining when measured using the ISO 4049 test , even though it has been reported that the distribution of resin curing in opaque molds can be substantially lower at or near the mold walls compared to the center of the specimen .
Optimum photoinitiator efficiency occurs at specific wavelengths that correspond to the peak absorptivity of the photoinitiator used within the RBC . Light-emitting diodes (LEDs) contain a p–n junction diode that emits light when a voltage is applied. Electrons move across the depletion region and combine with a hole on the other side to create an electron–hole pair and the electron releases its energy in the form of an emitted photon of light. The emission spectrum from LEDs is neither monochromatic like a laser, nor is it broad spectrum as from a tungsten lamp. Instead the wavelengths of light emitted from LEDs are determined by the specific bandgap of the semiconductor and the full width half maximum (FWHM) emission spectrum is typically only ±10 nm around the peak spectral emission from the LED. Consequently many dental LED-light curing units (LCUs) have an emission peak in the 450–470 nm wavelength range, with virtually no emissions below 420 nm or above 510 nm . Some manufacturers have introduced broad-spectrum LED units that contain multiple LED chips each delivering their own narrow emission spectrum. This produces a light source that delivers two or more distinct wavelength bands . These types of LCUs have been referred to as dual peak, broad-spectrum, or “polywave ® lights”. The ability of these broad-spectrum LCUs to deliver a uniform irradiance across the light emitting tips at all emitted wavelengths has recently been investigated. The results showed that the irradiance distribution and emission spectrum across the light tip can be inhomogeneous from these broad-spectrum LCUs and this can affect the resin polymerization .
The properties of a light-curing resin composite can be significantly influenced by the amount of radiant exposure received from the LCU . A strong relationship exists between the light curing protocol and the mechanical properties of the resin , and a positive correlation has been reported between the irradiance beam profile values from the LCU and the microhardness maps of the RBC surfaces . Hence, the shrinkage, hardness, elastic modulus, viscosity, etc. are also all related to the degree of conversion (DC) of the resin . For example, a higher radiant exposure at a given irradiance results in a higher DC and elastic modulus of the RBC . Among several techniques, measuring the hardness has proved to be the best indicator of the extent of polymerization of the RBC , and a strong positive correlation exists between the Knoop microhardness values and the DC within each brand of RBC . The hardness of RBCs has been used as an indirect method to assess the depth of cure with a value of 80% of hardness at the top surface considered as the borderline between sufficient and insufficient curing . The hardness and elastic modulus have also been correlated with the DC, depth of cure, and clinical behavior .
Several factors may affect the hardness of cured RBC such as the radiant exposure delivered to the RBC , composition and filler loading of the RBC , distance between the curing light tip and the RBC , and delayed finishing or polishing of the RBC specimens . A correlation between the hardness and elastic modulus has been suggested, and a hardness measurement has been used to estimate the modulus of RBCs . In a recent study, a new description for the depth of cure was proposed to be the depth at which the resin matrix switches from a glassy to a rubbery state . In this study, the resin modulus was obtained selecting the elastic modulus (E-mod) as the peak value corresponding to the resin after excluding the high modulus peak(s) that corresponded to the fillers. A rapid decrease in E-Mod was reported to reflect a marked change in the organic network of the RBC and the authors proposed that the true depth of cure is the point where the resin matrix switches from a glassy to a rubbery state . This work provides a useful philosophical perspective in understanding RBC properties as a function of curing, since many existing methodologies, used to determine depth of cure, fail to detect this transition; consequently previous literature may have overestimated the depth of cure in certain RBCs. However, accurate methods to determine the modulus of RBCs are required to validate and enhance the potential use of this approach. Recently, nanoindentation has evolved to permit continuous stiffness measurements (CSM) to be made. This method measures the hardness and elastic modulus of a material from the indentation load–displacement data recorded during a cycle of loading and unloading . The indenter tip component is conventionally made of diamond, formed into a sharp, symmetric shape such as the three-sided Berkovich pyramid. By adding a small sinusoidally varying signal on top of the signal that drives the indenter tip, modulus measurements can be obtained at any point along the loading curve of a given specimen. The benefits of this technique are well documented in the literature, however the small time constant associated with the CSM technique makes it especially useful to determine the modulus of polymeric materials .
In clinical situations, when using a broad-spectrum LED or polywave ® LCU, it is very likely that not all parts of the restoration will receive the same wavelengths of light. This cannot happen with LCUs that use filtered light from a halogen lamp as the light source . The effect of this phenomenon will depend on the RBC, the position of the LCU guide tip related to the RBC, the restoration size and shape, and the homogenization of the light from the different LEDs within the LCU. Two popular contemporary bulk-fill RBCs are Tetric EvoCeram Bulk Fill (TECBF, Ivoclar Vivadent, Liechtenstein) and Filtek Bulk Fill Flowable (FBFF, 3M ESPE, St. Paul, MN, USA). TECBF is a high viscosity RBC and the manufacturer recommends using a broad-spectrum polywave ® LED curing light to activate the alternative photoinitiators such as Lucirin TPO and Ivocerin that are used in this RBC . In contrast, FBFF is a low viscosity bulk-fill RBC that uses a ternary photoinitiator system comprised of camphorquinone (light absorber/sensitizer), ethyl-4-dimethyaminobenzoate (amine co-initiator/electron donor) and a diaryliodonium salt. FBFF does not require a broad-spectrum polywave ® LED curing light . The hypotheses of this study were that for these two types of RBC, the two different emission spectra produced by the two orientations of the light guide over the LED emitters (violet-blue) and (blue-blue) will: (1) exhibit similar patterns of nanohardness and elastic modulus measurements as the depth and distance from the center increase; and (2) that there would be a strong positive correlation between the hardness and elastic modulus values for each RBC.
2
Materials and methods
2.1
Resin based composite materials
To test the hypotheses, two bulk-fill dental RBCs were used; Tetric EvoCeram Bulk Fill (TECBF, Ivoclar Vivadent) and Filtek bulk fill flowable (FBFF, 3M ESPE). The material descriptions from the respective manufacturers are provided in Table 1 .
Material | Material Code | Viscosity | Manufacturer | Resin system | Filler | Filler wt %, vol % |
---|---|---|---|---|---|---|
Tetric EvoCeram Bulk-Fill | TECBF | High viscosity | Ivoclar Vivadent, Schaan, Liechtenstein | Dimethacrylate co-monomers | Barium aluminium silicate glass, ytterbium fluoride and mixed oxide | 80%, 61% |
Filtek Bulk-Fill Flowable | FBFF | Flowable | 3M ESPE, St. Paul, MN, USA | UDMA, Procrylat, Bis-EMA, Bis-GMA | Zirconia/silica and ytterbium trifluoride | 64.5%, 42.5% |
2.2
Specimen preparation:
Specimens were prepared using a custom-made stainless steel mold as illustrated in Fig. 1 .
One half of the split metal mold had a 6 mm diameter 10 mm long semi-circular notch that faced a 6 mm wide 0.2 mm deep indent in the opposing metal surface ( Fig. 1 A). This indent produced a consistent excess of RBC that was removed when the specimen was polished. The cover of the mold had a smooth flat surface. The mold was filled with RBC and a Mylar strip that fit into the indent was placed over the RBC and then both parts of the mold were screwed together ( Fig. 1 B). The mold was placed on a glass slide covered by a second Mylar strip, and additional RBC was packed into the mold. The top surface of the RBC was covered with a third Mylar strip and then compressed by a second glass slide. The Mylar strips were used to prevent oxygen inhibition of the RBC . The RBCs were photo-polymerized by using a polywave ® LED (Bluephase Style, Ivoclar Vivadent) for ten seconds with the tip of the light guide 0.5 mm from the top surface of the RBC. To deliver the two different emission spectra from the same LCU, the light source was oriented to allow only two LED emitters out of the three LEDs to face the RBC specimen. The third LED emitter was oriented to face the stainless steel mold ( Fig. 2 ), and thus no light from this chip reached the RBC. Two configurations of emitters were selected: either one violet and one blue emitter (violet-blue), or two blue emitters (blue-blue). The light source was held in a fixed position for all samples using a restraining clamp. To confirm that there was no light from the third LED emitter reaching the RBC, the light in these two positions was analyzed using a laser beam profiler camera as previously described . In brief, the LCU guide tip was placed in the above-described positions on one side of the mold and the beam was examined from the other side of the mold ( Fig. 2 ) using a laser beam profiler camera with a 50 mm focal length lens (USB-L070, Ophir-Spiricon, Logan, UT, USA). The resulting images were viewed using Beamgage version 5.11 software (Ophir-Spiricon).
A total of 20 RBC specimens were prepared, 10 specimens for each RBC. Specimens for each brand of resin composite were divided into two groups (5 specimens each) depending on the orientation of LED emitters. The nanoindenter requires a flat and smooth surface for specimen examination. Thus, the RBC specimens were polished using a sequence of felt cloths with 15, 9, 3, 1, and 0.5 μm embedded diamond, Al 2 O 3 and SiO 2 grains using copious amounts of water coolant to prevent any heating of the RBC. Each step was carried out for 3 mins with light pressure applied to the specimen against the felt cloths on the polishing machine. The RBC specimens were ultrasonically cleaned in distilled water for 3 mins and then stored in a dark container in air at 37 °C for 24 h.
2.3
Surface quality verification
To ensure that the preparation and polishing techniques were able to achieve acceptable standards for nanoindenter use, the surfaces of the TECBF and FBFF specimens were examined before indentation using an optical microscope. Some specimens were also measured after nanoindentation using a Hitachi S-4700 SEM (Hitachi High Technologies America, Inc., Pleasanton, CA). A representative image is shown in Fig. 3 .
2.4
Nano-indentation
Indentation tests were performed using an Agilent G200 nanoindentor (Agilent Technologies Canada Inc. Mississauga, ON) equipped with a continuous stiffness measurement (CSM) module. Indentation tests were performed at room temperature, using a calibrated and certified Berkovich diamond tip indenter with a tip radius < 20 ηm. The following criteria were used to standardize the indentation tests:
- 1.
The surface approach velocity for each indent was 10 ηm/s.
- 2.
The allowable drift rate was preset to 0.05 ηm/s.
- 3.
The surface detection (stiffness criteria) was pre-set to 200 ηm.
- 4.
The maximum depth limit per indent was 2000 ηm.
- 5.
The modulus was measured using the CSM module and calculated based on the load–displacement curve between an indentation depth of 400–1500 ηm.
Prior to use, the indenter was calibrated using a certified fused silica standard (Agilent Technologies Canada Inc. Mississauga, ON). A grid of 50 indents ( Fig. 4 ) was used to generate hardness and elastic modulus data for each specimen with five valid measurements recorded at each indent. Indents were placed along 5 lines down from the top surface of the specimen at 0.1 mm, 0.25 mm, 0.5 mm, and then at every 0.5 mm to a 4 mm depth from the surface. At each depth from the surface, five points were selected: at the centerline, then at 0.5 mm, and 2.5 mm laterally on each side of the centerline.
In several previous studies of microhardness/depth profiles, a depth corresponding to 80% of maximum hardness, representing a reduction of 20%, was considered as the borderline between sufficient and insufficient curing . In the present study, the depth where 50% of the maximum nanohardness occurred will be noted.
2.5
Statistical analysis
Five valid measurements of nanohardness and elastic modulus were recorded for each of the fifty selected points in the grid for each orientation of the blue-violet or blue-blue LEDs. The mean and standard deviation obtained at each point were used for the statistical analysis. To examine the effect of specimen orientation beneath the LEDs, multiple paired-sample t -tests were used to compare each value at the side (−2.5, −0.5, 0.5 and 2.5) with the corresponding value at the centerline at the same depth from the surface. The nanohardness and modulus values were compared separately.
One and two-way ANOVA followed by Bonferroni post-hoc analysis ( alpha = 0.05) were used to determine if significant changes occurred in the nanohardness and elastic modulus measurements as the depth from the surface increased. Results were compared separately for each RBC and light orientation (violet-blue and blue-blue). Similarly, comparisons were carried out for each point compared to a value that was 50% of the highest recorded nanohardness value for each RBC material. The coefficient of determination ( r 2 ) between nanohardness and elastic modulus for all four groups of RBC specimens was also reported.
2
Materials and methods
2.1
Resin based composite materials
To test the hypotheses, two bulk-fill dental RBCs were used; Tetric EvoCeram Bulk Fill (TECBF, Ivoclar Vivadent) and Filtek bulk fill flowable (FBFF, 3M ESPE). The material descriptions from the respective manufacturers are provided in Table 1 .
Material | Material Code | Viscosity | Manufacturer | Resin system | Filler | Filler wt %, vol % |
---|---|---|---|---|---|---|
Tetric EvoCeram Bulk-Fill | TECBF | High viscosity | Ivoclar Vivadent, Schaan, Liechtenstein | Dimethacrylate co-monomers | Barium aluminium silicate glass, ytterbium fluoride and mixed oxide | 80%, 61% |
Filtek Bulk-Fill Flowable | FBFF | Flowable | 3M ESPE, St. Paul, MN, USA | UDMA, Procrylat, Bis-EMA, Bis-GMA | Zirconia/silica and ytterbium trifluoride | 64.5%, 42.5% |
2.2
Specimen preparation:
Specimens were prepared using a custom-made stainless steel mold as illustrated in Fig. 1 .
One half of the split metal mold had a 6 mm diameter 10 mm long semi-circular notch that faced a 6 mm wide 0.2 mm deep indent in the opposing metal surface ( Fig. 1 A). This indent produced a consistent excess of RBC that was removed when the specimen was polished. The cover of the mold had a smooth flat surface. The mold was filled with RBC and a Mylar strip that fit into the indent was placed over the RBC and then both parts of the mold were screwed together ( Fig. 1 B). The mold was placed on a glass slide covered by a second Mylar strip, and additional RBC was packed into the mold. The top surface of the RBC was covered with a third Mylar strip and then compressed by a second glass slide. The Mylar strips were used to prevent oxygen inhibition of the RBC . The RBCs were photo-polymerized by using a polywave ® LED (Bluephase Style, Ivoclar Vivadent) for ten seconds with the tip of the light guide 0.5 mm from the top surface of the RBC. To deliver the two different emission spectra from the same LCU, the light source was oriented to allow only two LED emitters out of the three LEDs to face the RBC specimen. The third LED emitter was oriented to face the stainless steel mold ( Fig. 2 ), and thus no light from this chip reached the RBC. Two configurations of emitters were selected: either one violet and one blue emitter (violet-blue), or two blue emitters (blue-blue). The light source was held in a fixed position for all samples using a restraining clamp. To confirm that there was no light from the third LED emitter reaching the RBC, the light in these two positions was analyzed using a laser beam profiler camera as previously described . In brief, the LCU guide tip was placed in the above-described positions on one side of the mold and the beam was examined from the other side of the mold ( Fig. 2 ) using a laser beam profiler camera with a 50 mm focal length lens (USB-L070, Ophir-Spiricon, Logan, UT, USA). The resulting images were viewed using Beamgage version 5.11 software (Ophir-Spiricon).
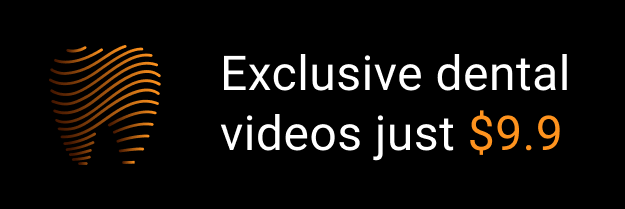