Abstract
Objectives
1-ethyl-3-(3-dimethylaminopropyl) carbodiimide hydrochloride (EDC) has been shown to cross-link dentin type I collagen. Increased cross-linking usually elevates the glass transition temperature of polymers. The aim of this study was to evaluate the cross-linking reaction promoted by EDC in different aqueous concentrations by measuring the thermal denaturation temperature ( T d ) of human dentin collagen.
Methods
The T d of dehydrated collagen and of insoluble dentin matrix collagen immersed in 0.5 M or 1 M EDC aqueous solution for different treatment times was obtained using a Differential Scanning Calorimeter (DSC). Specimens were also analyzed by Energy Dispersive X-Ray Spectroscopy.
Results
EDC-treated dentin collagen showed a significantly higher T d than the untreated specimens when immersed in either 0.5 M EDC or 1 M EDC for 10 min or longer ( p < 0.05). EDC-treated dentin collagen showed an increase of sulfur and chloride, not detectable in EDC-untreated dentin specimens. Conversely, the relative amount of carbon, nitrogen and oxygen was not modified by treatments.
Significance
EDC-treated dentin collagen showed a higher T d than the untreated control at all tested concentrations and immersion times. A higher T d can be considered an indirect indicator of a more resistant and highly cross-linked collagen network. More data are needed to confirm these results.
1
Introduction
Dentin adhesive systems have greatly improved in the last decades. However, the degradation of the collagen matrix at the resin–dentin interface after resin bonding is still a potential drawback that adversely affects the longevity of the bonded interface . Acid-etching procedures used in etch-and-rinse adhesive techniques expose the dentin collagen matrix and activate endogenous matrix-metalloproteinases (MMPs) , which are usually inactive in mineralized dentin and bone . In addition to MMPs, mineralized dentin contains cysteine cathepsins (in particular cathepsin K) that is also involved in collagen degradation within the hybrid layer. MMPs and cathepsins act synergistically and gradually destroy the exposed collagen fibrils within the hybrid layer, when sub-optimally infiltrated by the adhesive resin . This degradation process may cause the loss of resin–dentin bond strength and failure of the adhesive interface .
Type I collagen represents the 90% of the dentin organic matrix, determining its biomechanical properties and functional integrity . Collagen is stabilized through the formation of native pyridinium cross-links , which reduce its solubility and impart high tensile properties to the molecule. Such intramolecular cross-links are formed over time during the maturation process of tissues or in response to a disease and strengthen aggregated forms of collagen fibrils . Lysine and hydroxylysine residues contained in collagen telopeptides are implicated in the cross-linking process . The oxidative deamination of lysine or hydroxylysine in telopeptides, followed by the formation of pyridinium bonds increase the number of covalent inter- and intramolecular collagen cross-links in natural cross-linking of collagen over time. Later modifications of the collagen matrix have important structural and mechanical consequences, and the degradation of cross-links can lead to severe loss of tissue properties . Collagen cross-linking is considered an effective approach to enhance the insolubility and resistance of the demineralized dentin matrix to endogenous enzymes and to obtain a stable hybrid layer with improved mechanical strength . It has been shown that the stability of collagen fibrils can be artificially increased by pretreatment of acid-etched dentin with cross-linking agents . Cross-linking collagen increases its resistance to collagenases by preventing unwinding of the triple elix . Various compounds have been studied . Glutaraldehyde and grape-seed extracts have been proposed as cross-linking agents, even though glutaraldehyde can be toxic . As an alternative, 0.1% riboflavin applied to acid-etched dentin followed by UVA light exposure for 2 min prior to resin-bonding was proposed to inhibit dentin MMPs and increase the hybrid layer stability by creating cross-links . In previous studies, the application of 0.1 or 1% riboflavin to demineralized dentin treated with UVA or a dental blue light doubled the ultimate tensile strength of collagen and reduced the amount of hydroxyproline release after bacterial collagenase challenge . Carbodiimide compounds represent the most popular and versatile agents for cross-linking the carboxylic moieties bonded to the side chains of collagen. In particular, 1-ethyl-3-[3-dimethylaminopropyl] carbodiimide hydrochloride (EDC), an imide-based zero-length cross-linking agent, has been proved to be effective for cross-linking dentin collagen and altering the three-dimensional structure of endogenous collagen-degrading enzymes ( Fig. 1 ). Thus, EDC is able to inhibit the enzymatic degradation of exposed collagen fibrils within the hybrid layer, thereby improving bond durability over time.

Thermal stability of type-I collagen in connective tissues and dentin has been previously investigated by differential scanning calorimetry (DSC), a sensitive tool for monitoring the thermal denaturation process of heated collagen. During heating of collagen, hydrogen bonds are destroyed and the thermally induced structural transitions of the collagen network can be monitored by DSC by noting the temperature associated with an endothermic DSC peak. Thermal energy is absorbed when collagen is converted to gelatin. This is analogous to the glass transition temperatures of synthetic peptides.
Thus, the aim of this study was to assess the effect of EDC on the thermal stability of dentin collagen. The null hypotheses tested were that (1) there is no difference in the thermal degradation resistance of dentin collagen after treatment with an EDC water solution; (2) the effect of EDC is unrelated to concentrations and exposure times.
2
Materials and methods
2.1
Dentinal collagen preparation
Dentin slabs (1 mm thick, 6 mm in diameter) were obtained from human third molars collected after patient’s informed consent was obtained under a protocol approved by the University of Trieste (Italy), stored in 0.5% chloramine in water at 4 °C and used within 1 month after extraction. Each specimen was immersed in 10% H 3 PO 4 solution for 24 h to completely demineralize its collagen, and then carefully rinsed in MilliQ water in agitation for at least other 24 h.
To verify the complete demineralization of dentin, the intensity of the phosphate mineral peaks was monitored by Fourier Transform Infrared (FTIR) spectroscopy (Nicolet 6700, Thermo Scientific, Milan, Italy). A preliminary FTIR spectrum of each specimen was acquired before the immersion in 10% H 3 PO 4 solution and correlated to a spectrum acquired after the demineralization process. Each dentin slab was placed on the diamond crystal of a horizontal attenuated total reflectance (ATR) stage positioned in the optical compartment of a FTIR spectrophotometer at room temperature. Infrared (IR) spectra were obtained between 4000 and 525 cm −1 at 6 cm −1 resolution. The complete dissolution of the mineral was verified by the disappearance of the most evident mineral peak (PO 4 3− at 1004 cm −1 ) on both sides of each disk. To ensure that all the depth of the specimens was demineralized, 5 additional dentin disks were perpendicularly cut and analyzed with the ATR-FTIR along the cut, i.e. the disk thickness.
2.1.1
Differential Scanning Calorimeter analysis (DSC)
The tested cross-linking agent was N -(3-dimethylaminopropyl)- N ʹ-ethylcarbodiimide hydrochloride, (EDC) (FM = 191.70 g/mol, CAS number 25952-53-8, Sigma–Aldrich,) in aqueous solution at different concentrations: Group 1 : demineralized dentin collagen treated with 0.5 M EDC; Group 2 : demineralized dentin collagen treated with 1 M EDC; Group 3 : untreated demineralized dentin control.
Each demineralized dentin collagen slab was radially sectioned in six parts and dried in anhydrous atmosphere with SiO 2 . One specimen was analyzed with a differential scanning calorimeter (DSC, Q10 TA Instruments, New Castle, DE, USA) without EDC treatment, to obtain the thermal denaturation temperature ( T d ) of untreated dehydrated collagen (control). The other five pieces of each slab were kept immersed in the assigned EDC solution for 10, 20, 30 or 60 min ( T 10 , T 20 , T 30 , T 60 respectively), and then rinsed for 15 min in demineralized water under agitation, dehydrated and analyzed with the DSC to obtain the T d of EDC-treated anhydrous collagen. Five repetitions for each test condition were made.
Data were statistically analyzed with a one-way ANOVA and Tukey’s post hoc test and t -test for repeated measures at α = 0.05.
2.2
Energy Dispersive X-Ray Spectroscopy (EDS)
Both control and EDC-treated specimens were analyzed to obtain detailed information of the elemental composition of both treated and untreated dentin using a field emission environmental Scanning Electron Microscope (FEI Quanta 200, FEI Hillsboro, Oregon, USA) equipped with EDAX X-ray energy dispersive spectrometer (EDAX Inc., Mahwah, NJ, USA).
Briefly, freshly EDC-treated and dehydrated dentin slabs were placed onto the microscope’s aluminum specimen stubs, previously covered with a conductive carbon adhesive disk (TAAB Ltd, Berks, England). The microscope was used in low vacuum mode at 30 kV accelerating voltage, at a magnification of 10,000×. The spectrometer unit was equipped with an ECON (Edax Carbon Oxygen Nitrogen) 6 Ultra-Thin Window X-ray detector, which is able to detect any chemical element over the atomic number 5 (boron) of the periodic table of elements. The analysis system was controlled by dedicated analysis software (Genesis, EDAX Inc., Mahwah, NJ, USA). Each specimen was measured three times with a time count of 100 s, and an amplification time of 51 μs and results were expressed as a percentage in relation to the total counts collected.
EDS results were analyzed with the Mann–Whitney U-test (level of significance, p < 0.05). The level of significance was adjusted according to the Bonferroni’s correction.
2
Materials and methods
2.1
Dentinal collagen preparation
Dentin slabs (1 mm thick, 6 mm in diameter) were obtained from human third molars collected after patient’s informed consent was obtained under a protocol approved by the University of Trieste (Italy), stored in 0.5% chloramine in water at 4 °C and used within 1 month after extraction. Each specimen was immersed in 10% H 3 PO 4 solution for 24 h to completely demineralize its collagen, and then carefully rinsed in MilliQ water in agitation for at least other 24 h.
To verify the complete demineralization of dentin, the intensity of the phosphate mineral peaks was monitored by Fourier Transform Infrared (FTIR) spectroscopy (Nicolet 6700, Thermo Scientific, Milan, Italy). A preliminary FTIR spectrum of each specimen was acquired before the immersion in 10% H 3 PO 4 solution and correlated to a spectrum acquired after the demineralization process. Each dentin slab was placed on the diamond crystal of a horizontal attenuated total reflectance (ATR) stage positioned in the optical compartment of a FTIR spectrophotometer at room temperature. Infrared (IR) spectra were obtained between 4000 and 525 cm −1 at 6 cm −1 resolution. The complete dissolution of the mineral was verified by the disappearance of the most evident mineral peak (PO 4 3− at 1004 cm −1 ) on both sides of each disk. To ensure that all the depth of the specimens was demineralized, 5 additional dentin disks were perpendicularly cut and analyzed with the ATR-FTIR along the cut, i.e. the disk thickness.
2.1.1
Differential Scanning Calorimeter analysis (DSC)
The tested cross-linking agent was N -(3-dimethylaminopropyl)- N ʹ-ethylcarbodiimide hydrochloride, (EDC) (FM = 191.70 g/mol, CAS number 25952-53-8, Sigma–Aldrich,) in aqueous solution at different concentrations: Group 1 : demineralized dentin collagen treated with 0.5 M EDC; Group 2 : demineralized dentin collagen treated with 1 M EDC; Group 3 : untreated demineralized dentin control.
Each demineralized dentin collagen slab was radially sectioned in six parts and dried in anhydrous atmosphere with SiO 2 . One specimen was analyzed with a differential scanning calorimeter (DSC, Q10 TA Instruments, New Castle, DE, USA) without EDC treatment, to obtain the thermal denaturation temperature ( T d ) of untreated dehydrated collagen (control). The other five pieces of each slab were kept immersed in the assigned EDC solution for 10, 20, 30 or 60 min ( T 10 , T 20 , T 30 , T 60 respectively), and then rinsed for 15 min in demineralized water under agitation, dehydrated and analyzed with the DSC to obtain the T d of EDC-treated anhydrous collagen. Five repetitions for each test condition were made.
Data were statistically analyzed with a one-way ANOVA and Tukey’s post hoc test and t -test for repeated measures at α = 0.05.
2.2
Energy Dispersive X-Ray Spectroscopy (EDS)
Both control and EDC-treated specimens were analyzed to obtain detailed information of the elemental composition of both treated and untreated dentin using a field emission environmental Scanning Electron Microscope (FEI Quanta 200, FEI Hillsboro, Oregon, USA) equipped with EDAX X-ray energy dispersive spectrometer (EDAX Inc., Mahwah, NJ, USA).
Briefly, freshly EDC-treated and dehydrated dentin slabs were placed onto the microscope’s aluminum specimen stubs, previously covered with a conductive carbon adhesive disk (TAAB Ltd, Berks, England). The microscope was used in low vacuum mode at 30 kV accelerating voltage, at a magnification of 10,000×. The spectrometer unit was equipped with an ECON (Edax Carbon Oxygen Nitrogen) 6 Ultra-Thin Window X-ray detector, which is able to detect any chemical element over the atomic number 5 (boron) of the periodic table of elements. The analysis system was controlled by dedicated analysis software (Genesis, EDAX Inc., Mahwah, NJ, USA). Each specimen was measured three times with a time count of 100 s, and an amplification time of 51 μs and results were expressed as a percentage in relation to the total counts collected.
EDS results were analyzed with the Mann–Whitney U-test (level of significance, p < 0.05). The level of significance was adjusted according to the Bonferroni’s correction.
3
Results
3.1
Differential Scanning Calorimeter analysis
Representative DSC thermograms of the tested specimens are shown in Fig. 2 . Endothermal peaks associated with the helix-coil transition due to thermal disruption of hydrogen bonds, which stabilize the collagen triple helix, were detectable in all specimens.

VIDEdental - Online dental courses
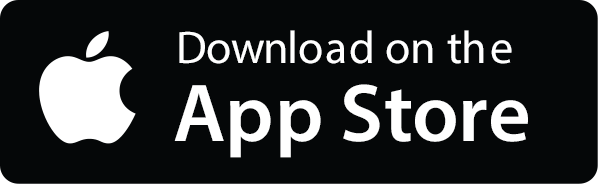
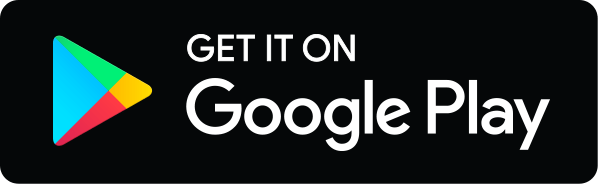