Abstract
Objective
To determine the effectiveness and efficiency of non-thermal, atmospheric plasmas for inducing polymerization of model dental self-etch adhesives.
Methods
The monomer mixtures used were bis-[2-(methacryloyloxy)ethyl] phosphate (2MP) and 2-hydroxyethyl methacrylate (HEMA), with mass ratios of 70/30, 50/50 and 30/70. Water was added to the above formulations: 10–30 wt%. These monomer/water mixtures were treated steadily for 40 s under a non-thermal atmospheric plasma brush working at temperatures from 32 to 35 °C. For comparison, photo-initiators were added to the above formulations for photo-polymerization studies, which were light-cured for 40 s. The degree of conversion (DC) of both the plasma- and light-cured samples was measured using FTIR spectroscopy with an attenuated total reflectance attachment.
Results
The non-thermal plasma brush was effective in inducing polymerization of the model self-etch adhesives. The presence of water did not negatively affect the DC of plasma-cured samples. Indeed, DC values slightly increased, with increasing water content in adhesives: from 58.3% to 68.7% when the water content increased from 10% to 30% in the adhesives with a 50/50 (2MP/HEMA) mass ratio. Conversion values of the plasma-cured groups were higher than those of light-cured samples with the same mass ratio and water content. Spectral differences between the plasma- and light-cured groups indicate subtle structural distinctions in the resultant polymer networks.
Significance
This research if the first to demonstrate that the non-thermal plasma brush induces polymerization of model adhesives under clinical settings by direct/indirect energy transfer. This device shows promise for polymerization of dental composite restorations having enhanced properties and performance.
1
Introduction
As a critical factor in improving the performance of dental composites, adhesive dentistry has greatly advanced, since Dr. Michael Buonocore introduced the acid-etching technique. Clearfil Bond System-F (Kuraray Co. Ltd., Tokyo, Japan) was the first commercially available etch-and-rinse system, introduced in 1978, which was applied using “dry bonding”. In 1992 , the concept of “wet-bonding” to a dentin surface was introduced. This technique avoids collagen collapse due to over-drying of demineralized dentin , but the protocol is extremely sensitive to moisture and clinical handling. Additionally, excessive water on the dentin surface may dilute hydrophilic monomers , which results in phase separation, poor degree of double-bond conversion, and lower bond strength. In order to simplify the protocols and reduce technique sensitivity, acidic monomers are employed as self-etch adhesives.
The self-etch adhesive systems including one-step and two-step are believed to be more user-friendly and less technique-sensitive , and demonstrate lower incidence of post-operative sensitivity, compared to the etch-and-rinse systems . However, some critical limitations still remain with self-etch adhesives, such as lower “immediate” bond strengths , increased interfacial nanoleakage , enhanced osmosis and phase-separation in HEMA-rich formulations , and continued demineralization of the surrounding hard tissue . One of the inherent problems with these light-cured, self-etch adhesives is the compromised polymerization initiating efficacy, which arises from the reaction of acidic monomers with amines used in the initiator systems (such as the camphorquinone/amine system in the most of current adhesives) . In addition, the presence of water dramatically decreases the degree of conversion and polymerization rate . The combination of compromised photopolymerization, presence of water, and the hydrophilic nature of self-etch adhesives would likely contribute to the above mentioned limitations.
Plasmas are partially ionized gases that contain highly reactive particles including electronically excited atoms, molecules, ionic and free radical species. Depending on their gas temperature range (300–10,000 K) , plasmas can be divided into thermal (high temperature) and non-thermal (low temperature or cold) categories. Non-thermal gas plasmas also contain the above reactive particles, while the gas phase remains near room temperature. Applications of thermal and non-thermal plasmas include: surface etching and cleaning, surface engineering and functionalization, deposition of organic and inorganic functional coatings, and sterilization of solid state biomaterials and medical devices . In dentistry, application of non-thermal plasma use has focused on killing of oral bacteria , dentin adhesion enhancement , and tooth bleaching .
It is also well known that plasmas induce polymerization . Polymers synthesized by plasma exposure demonstrate high cross-linking and high degrees of polymerization . However, plasmas used for these applications are created under a low pressure, high vacuum environment, which is not applicable to in vivo conditions. The need of reduced pressure or high vacuum for generating plasmas limits its biomedical applications. Plasmas created at one atmospheric pressure are usually of the high temperature nature, due to high gas density. Such high temperatures cannot be endured by living organisms, especially in the oral cavity. For example, the dental pulp is a very sensitive tissue: an intrapulpal temperature increase of 5.5 °C will result in 15% of pulp tissue necrosis . Recent development in atmospheric plasma technology has enabled generation of plasmas at a relatively low temperature. The patented non-thermal atmospheric plasma brush, developed in our plasma research center, can be ignited and sustained in an ambient atmosphere, at very low power consumption (several watts), with temperatures close to that of the human body. Previous studies indicate that this brush was highly effective in oral bacterial disinfection and interfacial bonding improvement of composite restorations.
Thus, the purpose of the current study is to examine the effectiveness of the non-thermal, atmospheric-pressure, plasma brush for inducing polymerization of one-step, model self-etch adhesives, without inclusion of photoinitiators. Conventional photo-activated polymerization of the model resin system was used as control. The research hypothesis tested was that the extent of monomer conversion of the model resin system would be significantly greater when using the plasma brush than when the same system was conventionally light-cured. In addition, it was hypothesized that the presence of water would not have a significant impact on resin conversion when using the plasma brush, but would cause a significant decrease in conversion in the photo-initiated systems.
2
Materials and methods
2.1
Model self-etch adhesive preparation
The monomer mixtures used in this study consisted of bis-[2-(methacryloyloxy)ethyl] phosphate (2MP) (Sigma–Aldrich, Milwaukee, WI, USA) and 2-hydroxyethyl methacrylate (HEMA) (Acros Organics, Morris Plain, NJ, USA). Resins were mixed in a mass ratio (2MP:HEMA) of 30:70, 50:50, 70:30, respectively. The chemical composition of the model resin system is similar to that of a commercial, self-etch adhesive (Clearfil Liner Bond 2V, Kuraray America, Inc., New York, NY, USA, based on the MSDS sheet). For comparison, photoinitiators, such as camphorquinone (CQ) and ethyl 4-dimethylaminobenzoate (EDMAB), were added to above formulations to provide photo-activation capability. Photoinitiator concentration (for each component) was maintained at 0.022 mmol/g of the monomer mixture, according to previous results . The photo-initiators were all obtained from Sigma–Aldrich and used without any further purification.
Deuterium oxide (D 2 O, Cambridge Isotope Laboratories, Inc., Andover, MA), at concentrations of 10, 20, or 30 wt %, was used to make the self-etch adhesive solutions. Using D 2 O instead of H 2 O avoided the interference in the IR absorption of H 2 O with that of C C double bonds in adhesives. Sufficient shaking and sonication were required to yield well-mixed solutions.
2.2
Non-thermal, atmospheric plasma brush
The non-thermal, atmospheric plasma brush employed in this study was designed by the Plasma Research Center at University of Missouri and Los Alamos National Laboratory. The detailed information about this device can be found in previous publications . Compressed argon gas (ultra high purity) was used as the plasma gas supply. A mass flow controller (MKS Instruments Inc., Andover, MA) was introduced to adjust the argon gas flow rate. A glow discharge by the direct current power source (Model 1556C, Power Designs Inc., Westbury, NY) was ignited between the two electrodes in a Teflon chamber. Fig. 1 shows a photograph of the plasma brush in the atmospheric environment. Plasma temperatures were characterized by a type K thermocouple and infrared imaging (TH 5104, Mikron Infrared Inc., Oakland, NJ).
2.3
FTIR spectroscopy
The infrared (IR) spectra of monomers, plasma-cured and light-cured adhesives were obtained using a Fourier transform infrared spectrometer (Spectrum One, Perkin-Elmer, Waltham, MA, USA) equipped with a universal attenuated total reflectance (ATR) attachment (Perkin-Elmer) at a resolution of 4 cm −1 . 128 scans were used and averaged to obtain a single spectrum. The ATR crystal was diamond with a transmission range between 650 and 4000 cm −1 .
2.3.1
Spectra of model adhesives before curing
A small volume of the adhesive/water mixture was cast on the diamond crystal top-plate of the ATR accessory, covered with a clear, polyester film (Mylar, 22 mm × 22 mm × 0.25 mm, Fisher Scientific, Pittsburgh, PA, USA). Spectra of the mixtures without any curing/treatment were obtained, and acted to supply absorption parameters of the specimens in the uncured state, which were used for calculation of the degree of conversion described below.
2.3.2
Spectra of plasma-cured adhesives
Glass slides were employed as the smooth solid substrates for the plasma-cured specimens. Each glass slide was uniformly treated with the non-thermal plasma brush for 60 s before applying one of the photoinitiator-free model adhesives onto it, which made it possible for the adhesive to spread evenly on the surface. The adhesive/water mixtures were cast on the glass slides to form very thin liquid films, and were then treated/cured using the non-thermal, plasma brush for 40 s steadily (as schematically shown in Fig. 2 ). The glass slides with plasma-cured adhesives were turned over and pressed against the horizontal face of the ATR diamond crystal where total internal reflection took place. The spectra of these plasma-cured adhesives were recorded. Six separate replications for each adhesive formulation were conducted.
2.3.3
Spectra of light-cured adhesives
The model adhesives containing photoinitiators were cast on the diamond crystal top-plate, covered with a clear, polyester film, and exposed for 40 s to a conventional dental light polymerization unit (Spectrum Light, Dentsply, Milford, DE, USA). The irradiance of the light (550 mW/cm 2 ) was measured using a visible curing light meter (CURE RITE, EFOS Inc., Williamsville, NY, USA), having a digital display. The spectra of the light-cured adhesives were obtained. Three separate replications for each adhesive formulation were conducted.
2.4
Calculation of the degree of conversion
Two characteristic bands 1637 cm −1 (stretching of methacrylate double bond C C) and 1715 cm −1 (stretching of carbonyl group C O) were employed to calculate the DC of polymerization induced by both the light and non-thermal plasma curing. The intensities of these two bands were calculated based on band height methodology, and the changes of the band ratios profile at 1637 cm −1 and 1715 cm −1 were monitored. The DC was calculated by the following equation:
DC ( % ) = 1 − Absorbanc e 1637 cm − 1 sample / Absorbanc e 1715 cm − 1 sample Absorbanc e 1637 cm − 1 monomer / Absorbanc e 1715 cm − 1 monomer × 100
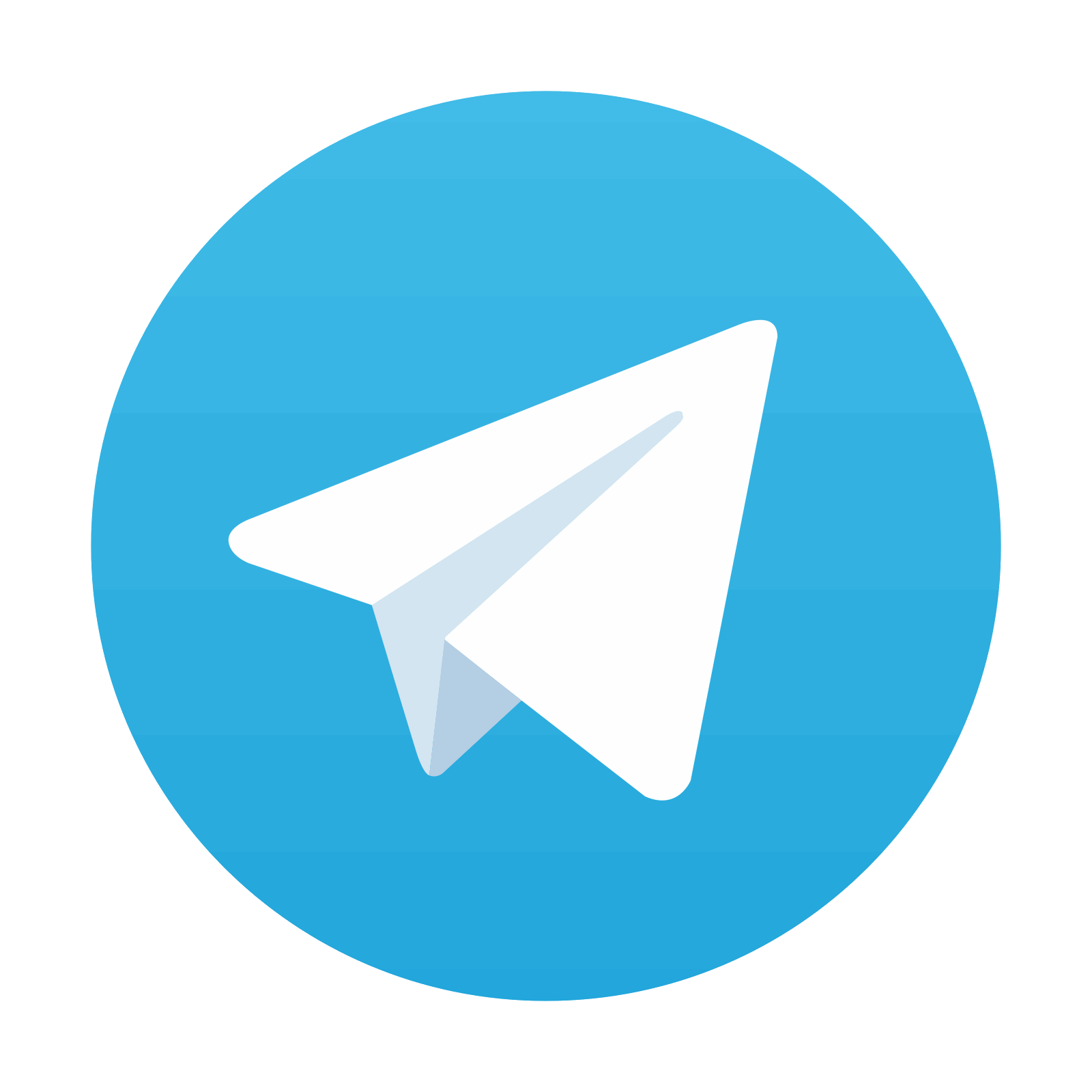
Stay updated, free dental videos. Join our Telegram channel

VIDEdental - Online dental courses
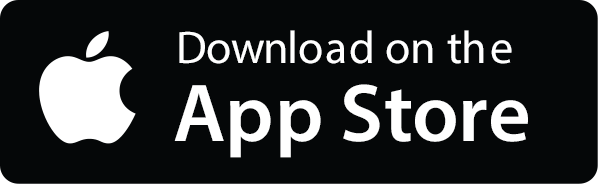
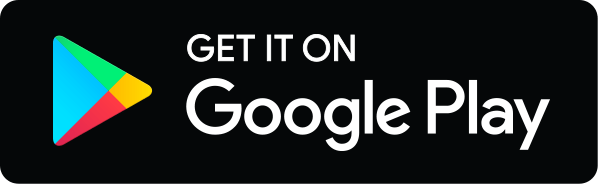