Abstract
A static bond-strength test is often regarded as clinically less relevant, since such abrupt loading of the adhesive-tooth bond clinically never occurs. Therefore, dynamic fatigue testing is often claimed to better predict the clinical effectiveness of adhesives.
Objectives
To measure the micro-tensile fatigue resistance (μTFR) of adhesives bonded to dentin, and to compare their μTFR to their micro-tensile bond strength (μTBS).
Methods
The bonding effectiveness (including fracture analysis) of three adhesives (OptiBond FL, Kerr: 3-step etch-and-rinse adhesive or 3-E&Ra; Clearfil SE, Kuraray: 2-step self-etch adhesive or 2-SEa; G-Bond, GC: 1-step self-etch adhesive or 1-SEa) was measured by means of both a dynamic μTFR and a static μTBS approach. Preparation and test set-up of the micro-specimens were identical for both tests. In fatigue, specimens were tested with a wide range of selected loads at 2 Hz and at 10 Hz until failure, or until 10 4 cycles were reached. At 2 Hz, the μTFR was also measured after 3-month water storage. The μTFR was determined using a logistic regression model. Two-way ANOVA and Tukey HSD multiple comparisons test were used to determine statistical differences in μTBS.
Results
The 1-SEa recorded significantly lower values in μTFR at 10 Hz and in μTBS than the 2-SEa and 3-E&Ra. The 1-SEa and the 2-SEa performed significantly lower in μTFR than the 3-E&Ra, when tested at 2 Hz after 3-month water storage. Fatigue testing at 2 Hz after 1-week water storage did not reveal any differences in μTFR between the three adhesives.
Significance
The 3-E&Ra performed best in terms of bonding effectiveness, irrespective of the experimental condition or test used. The μTBS test proved once more to be a reliable laboratory test in ranking contemporary adhesives on their bonding effectiveness.
1
Introduction
When assessing the bonding effectiveness of adhesives in vitro , the ultimate goal of the selected methodology should be to provide data that can predict clinical performance, by preference also on the long term. Despite its limitations and lack of standardized test protocol, micro-tensile bond strength (μTBS) testing remains the most popular tool to assess the bonding effectiveness of contemporary dental adhesives . Under clinical circumstances, however, the adhesive interface of a restoration is hardly submitted to such acute tensile stresses; it is rather exposed to cyclic, sub-critical loadings produced during chewing . Nowadays, there definitely exists a need to supplement static bond-strength data with dynamic fatigue data in better simulation of clinical functioning . In this context, fatigue testing is supposed to provide more reliable results, and thus to better predict the in vivo performance of dental adhesives as more clinically relevant, dynamic loadings are applied . However, since these fatigue tests are much more labor-intensive than static bond-strength tests, so far only few researchers have employed fatigue testing to assess the bonding effectiveness of adhesives to tooth enamel and dentin . Doing so, different fatigue test protocols have been used, among which a macro/micro-shear test , a macro/micro-push-out test a micro-rotary fatigue test , and most recently also four-point bending approach . Unfortunately, despite an alleged need no standard for fatigue testing of adhesives today exists .
Class-V clinical trials remain the ultimate test to evaluate adhesives, although in vivo study designs still call for standardization as well . In non-carious Class-V restorations, not only temperature changes, but also synergistic abfraction forces are responsible for the production of sub-critical fatigue stresses that eventually may cause the restoration to de-bond . Degradation of adhesive interfaces in vivo also occurs by exposure of the hybrid layer to the humid oral environment and the consequent hydrolysis of its organic and resinous components .
As in such clinical trials, tensile stresses and water degradation are very detrimental to the bond survival, the purpose of this study was (1) to measure the micro-tensile fatigue resistance (μTFR) to dentin of three adhesives, representing the three major classes of contemporary adhesives, and (2) to compare the dynamically obtained results with the respective static μTBS determined for the same adhesives using the same micro-specimen preparation protocol. In addition, in order to determine the most relevant parameters for fatigue testing, the μTFR was determined at two different load frequencies and water storage periods. The null hypotheses tested were that there were no relative differences between μTFR and μTBS for the three adhesives tested, and that the load frequency and water storage co-variables did not affect the μTFR.
2
Materials and methods
2.1
Micro-specimen preparation
Non-carious and non-restored third molars (gathered following informed consent approved by the Commission for Medical Ethics of the Catholic University of Leuven) were stored in 0.5% chloramine in water at 4 °C and used within 1 month after extraction. All teeth were mounted in gypsum blocks in order to facilitate manipulation, after which the occlusal third of the molar crowns was removed by means of a water-cooled slow-speed diamond saw (Isomet 1000, Buehler, Lake Bluff, IL, USA). Dentin surfaces were verified for absence of enamel and/or pulp tissue using a stereomicroscope (Wild M5A, Heerbrug, Switzerland). A standard smear layer was produced by removing a thin layer of the dentin surface using a water-cooled, high-speed medium-grit (100 μm) diamond bur (842, Komet, Lemgo, Germany) mounted in a custom-adapted MicroSpecimen Former (The University of Iowa, Iowa City, IA, USA). The teeth were randomly divided in three groups according to the adhesive tested: a 3-step etch-and-rinse adhesive (3-E&Ra: OptiBond FL, Kerr, Orange, CA, USA), a 2-step self-etch adhesive (2-SEa: Clearfil SE, Kuraray, Osaka, Japan), and a 1-step self-etch adhesive (1-SEa: G-Bond, GC, Tokyo, Japan). The bonding treatment was performed strictly according to the manufacturer’s instructions ( Table 1 ). After adhesive treatment, the surfaces were built up with the micro-hybrid resin composite Z100 (Shade A3; LOT 20050413 exp 2008-03; LOT 6AB exp 2009-03; 3M ESPE, St. Paul, MN, USA) in five layers to a height of 5–6 mm. Each layer was light-cured for 40 s using an Optilux 500 light-curing unit (Demetron/Kerr, Danbury, CT, USA) with a light output of not less than 550 mW/cm 2 . After storage of the teeth for 7 days in 0.5% chloramine at 37 °C ( Fig. 1 ), they were sectioned perpendicular to the bonding surface using a fully automatic saw (Accutom-50, Struers, Copenhagen, Denmark) into square specimens of 1 mm × 1 mm. The width and thickness of each specimen was measured to the nearest 0.01 mm using a digital caliper (Mitutoyo, Tokyo, Japan). For each micro-specimen, a bonding surface of about 1 mm 2 was obtained.
Adhesive | Composition a [lot number] | Application |
---|---|---|
OptiBond FL (Kerr, Orange, CA, USA) | Etching : 37.5% phosphoric acid, silica thickener [432588] | Etch for 15 s; rinse for 15 s; gently air dry for 5 s; scrub the surface for 15 s with primer; apply a thin coat of bonding agent and light cure for 30 s |
Primer : HEMA, GPDM, PAMM, ethanol, water, photo-initiator [449359] | ||
Adhesive : TEGDMA, UDMA, GPDM, HEMA, bis-GMA, filler, photo-initiator [452270] | ||
Clearfil SE Bond (Kuraray, Tokyo, Japan) | Self-etch primer : 10-MDP, HEMA, hydrophilic dimethacrylate, photo-initiator, water [00654A] | Apply primer for 20 s; gently air blow; apply bonding agent; light cure for 10 s |
Adhesive : 10-MDP, bis-GMA, HEMA, hydrophilic dimethacrylate, microfiller [00931A] | ||
G-Bond (GC, Tokyo, Japan) | 4-MET, DMA, Pam, photo-initiator, stabilizer, filler, acetone, water [0507271] | Apply adhesive; keep dentin wet for 10 s; strongly air dry; light cure for 10 s |
a Composition as provided by respective manufacturer: HEMA = hydroxyethylmethacrylate; GPDM = glycerol phosphate dimethacrylate; PAMM = phthalic acid monoethyl methacrylate; TEGDMA = triethylene glycol dimethacrylate; UDMA = urethane dimethacrylate; Bis-GMA = bisphenol-glycidyl methacrylate; 10-MDP = 10-methacryloyloxydecyl dihydrogen phosphate; 4-MET = 4-methacryloxyethyltrimetellitic acid; DMA = dimethacrylate; Pam = phosphoric acid monomer.
2.2
μTBS
The micro-specimens were fixed with cyanoacrylate glue (Model Repair II Blue, Dentsply-Sankin, Ohtawara, Japan) onto a notched BIOMAT jig . The μTBS of each micro-specimen was determined in a universal testing machine (Instron 5848 Micro Tester, High Wycombe, Bucks, UK) using a load cell of 500 N and a crosshead speed of 1 mm/min. The μTBS of each specimen was calculated in MPa, by dividing the imposed force (in N) at the time of fracture by its cross-sectional bond area (in mm 2 ). All specimens were maintained moist throughout the whole preparation and test procedure.
2.3
μTFR
Preparation and test set-up of the micro-specimens were identical for both μTBS and μTFR. In fatigue, micro-specimens were tested under sinusoidal load control at 2 Hz and 10 Hz until failure or until 10 4 cycles were reached. For each adhesive, specimens were tested with a wide range of adjusted loads. At 2 Hz, micro-specimens were tested after 1 week and after 3 months of water storage. Specimens surviving μTFR were later also subjected to the μTBS test. One operator carried out all procedures to avoid operator variability.
2.4
Failure analysis and Feg-SEM examination
All specimens tested were semi-quantitatively analyzed for the mode of failure using a stereomicroscope at a magnification of 50× (Wild M5A, Heerbrug, Switzerland). Failures were recorded as either ‘cohesive in dentin’, ‘mixed failure’ (including interfacial failure and areas that failed partially ‘adhesively’ between tooth and resin, and/or partially ‘cohesively’ in resin or tooth), or ‘cohesive in resin’. Representative specimens were selected for fractographic examination by Feg-SEM (Philips XL30, Eindhoven, The Netherlands), and processed appropriately, including fixation in glutaraldehyde, dehydration in ascending concentrations of ethanol, and chemical drying following the protocol described in detail by Perdigão et al. .
2.5
Statistical analysis
After the data distribution was tested for deviations from a normal distribution, two-way analysis of variance (ANOVA) and Tukey HSD multiple comparisons test were used to determine statistical differences in μTBS. All data were analyzed at a significance level of 0.05. The distribution of the fatigue test data, the 25% and 75% quartiles, and the mean load at which 50% of the samples failed (μTFR), were determined (in MPa) using a logistic regression. Multiple logistic regressions were used to determine statistical differences in μTFR. All statistics were performed using the Statistica software package (Stat Soft, Tulsa, OK, USA).
2
Materials and methods
2.1
Micro-specimen preparation
Non-carious and non-restored third molars (gathered following informed consent approved by the Commission for Medical Ethics of the Catholic University of Leuven) were stored in 0.5% chloramine in water at 4 °C and used within 1 month after extraction. All teeth were mounted in gypsum blocks in order to facilitate manipulation, after which the occlusal third of the molar crowns was removed by means of a water-cooled slow-speed diamond saw (Isomet 1000, Buehler, Lake Bluff, IL, USA). Dentin surfaces were verified for absence of enamel and/or pulp tissue using a stereomicroscope (Wild M5A, Heerbrug, Switzerland). A standard smear layer was produced by removing a thin layer of the dentin surface using a water-cooled, high-speed medium-grit (100 μm) diamond bur (842, Komet, Lemgo, Germany) mounted in a custom-adapted MicroSpecimen Former (The University of Iowa, Iowa City, IA, USA). The teeth were randomly divided in three groups according to the adhesive tested: a 3-step etch-and-rinse adhesive (3-E&Ra: OptiBond FL, Kerr, Orange, CA, USA), a 2-step self-etch adhesive (2-SEa: Clearfil SE, Kuraray, Osaka, Japan), and a 1-step self-etch adhesive (1-SEa: G-Bond, GC, Tokyo, Japan). The bonding treatment was performed strictly according to the manufacturer’s instructions ( Table 1 ). After adhesive treatment, the surfaces were built up with the micro-hybrid resin composite Z100 (Shade A3; LOT 20050413 exp 2008-03; LOT 6AB exp 2009-03; 3M ESPE, St. Paul, MN, USA) in five layers to a height of 5–6 mm. Each layer was light-cured for 40 s using an Optilux 500 light-curing unit (Demetron/Kerr, Danbury, CT, USA) with a light output of not less than 550 mW/cm 2 . After storage of the teeth for 7 days in 0.5% chloramine at 37 °C ( Fig. 1 ), they were sectioned perpendicular to the bonding surface using a fully automatic saw (Accutom-50, Struers, Copenhagen, Denmark) into square specimens of 1 mm × 1 mm. The width and thickness of each specimen was measured to the nearest 0.01 mm using a digital caliper (Mitutoyo, Tokyo, Japan). For each micro-specimen, a bonding surface of about 1 mm 2 was obtained.
Adhesive | Composition a [lot number] | Application |
---|---|---|
OptiBond FL (Kerr, Orange, CA, USA) | Etching : 37.5% phosphoric acid, silica thickener [432588] | Etch for 15 s; rinse for 15 s; gently air dry for 5 s; scrub the surface for 15 s with primer; apply a thin coat of bonding agent and light cure for 30 s |
Primer : HEMA, GPDM, PAMM, ethanol, water, photo-initiator [449359] | ||
Adhesive : TEGDMA, UDMA, GPDM, HEMA, bis-GMA, filler, photo-initiator [452270] | ||
Clearfil SE Bond (Kuraray, Tokyo, Japan) | Self-etch primer : 10-MDP, HEMA, hydrophilic dimethacrylate, photo-initiator, water [00654A] | Apply primer for 20 s; gently air blow; apply bonding agent; light cure for 10 s |
Adhesive : 10-MDP, bis-GMA, HEMA, hydrophilic dimethacrylate, microfiller [00931A] | ||
G-Bond (GC, Tokyo, Japan) | 4-MET, DMA, Pam, photo-initiator, stabilizer, filler, acetone, water [0507271] | Apply adhesive; keep dentin wet for 10 s; strongly air dry; light cure for 10 s |
a Composition as provided by respective manufacturer: HEMA = hydroxyethylmethacrylate; GPDM = glycerol phosphate dimethacrylate; PAMM = phthalic acid monoethyl methacrylate; TEGDMA = triethylene glycol dimethacrylate; UDMA = urethane dimethacrylate; Bis-GMA = bisphenol-glycidyl methacrylate; 10-MDP = 10-methacryloyloxydecyl dihydrogen phosphate; 4-MET = 4-methacryloxyethyltrimetellitic acid; DMA = dimethacrylate; Pam = phosphoric acid monomer.
2.2
μTBS
The micro-specimens were fixed with cyanoacrylate glue (Model Repair II Blue, Dentsply-Sankin, Ohtawara, Japan) onto a notched BIOMAT jig . The μTBS of each micro-specimen was determined in a universal testing machine (Instron 5848 Micro Tester, High Wycombe, Bucks, UK) using a load cell of 500 N and a crosshead speed of 1 mm/min. The μTBS of each specimen was calculated in MPa, by dividing the imposed force (in N) at the time of fracture by its cross-sectional bond area (in mm 2 ). All specimens were maintained moist throughout the whole preparation and test procedure.
2.3
μTFR
Preparation and test set-up of the micro-specimens were identical for both μTBS and μTFR. In fatigue, micro-specimens were tested under sinusoidal load control at 2 Hz and 10 Hz until failure or until 10 4 cycles were reached. For each adhesive, specimens were tested with a wide range of adjusted loads. At 2 Hz, micro-specimens were tested after 1 week and after 3 months of water storage. Specimens surviving μTFR were later also subjected to the μTBS test. One operator carried out all procedures to avoid operator variability.
2.4
Failure analysis and Feg-SEM examination
All specimens tested were semi-quantitatively analyzed for the mode of failure using a stereomicroscope at a magnification of 50× (Wild M5A, Heerbrug, Switzerland). Failures were recorded as either ‘cohesive in dentin’, ‘mixed failure’ (including interfacial failure and areas that failed partially ‘adhesively’ between tooth and resin, and/or partially ‘cohesively’ in resin or tooth), or ‘cohesive in resin’. Representative specimens were selected for fractographic examination by Feg-SEM (Philips XL30, Eindhoven, The Netherlands), and processed appropriately, including fixation in glutaraldehyde, dehydration in ascending concentrations of ethanol, and chemical drying following the protocol described in detail by Perdigão et al. .
2.5
Statistical analysis
After the data distribution was tested for deviations from a normal distribution, two-way analysis of variance (ANOVA) and Tukey HSD multiple comparisons test were used to determine statistical differences in μTBS. All data were analyzed at a significance level of 0.05. The distribution of the fatigue test data, the 25% and 75% quartiles, and the mean load at which 50% of the samples failed (μTFR), were determined (in MPa) using a logistic regression. Multiple logistic regressions were used to determine statistical differences in μTFR. All statistics were performed using the Statistica software package (Stat Soft, Tulsa, OK, USA).
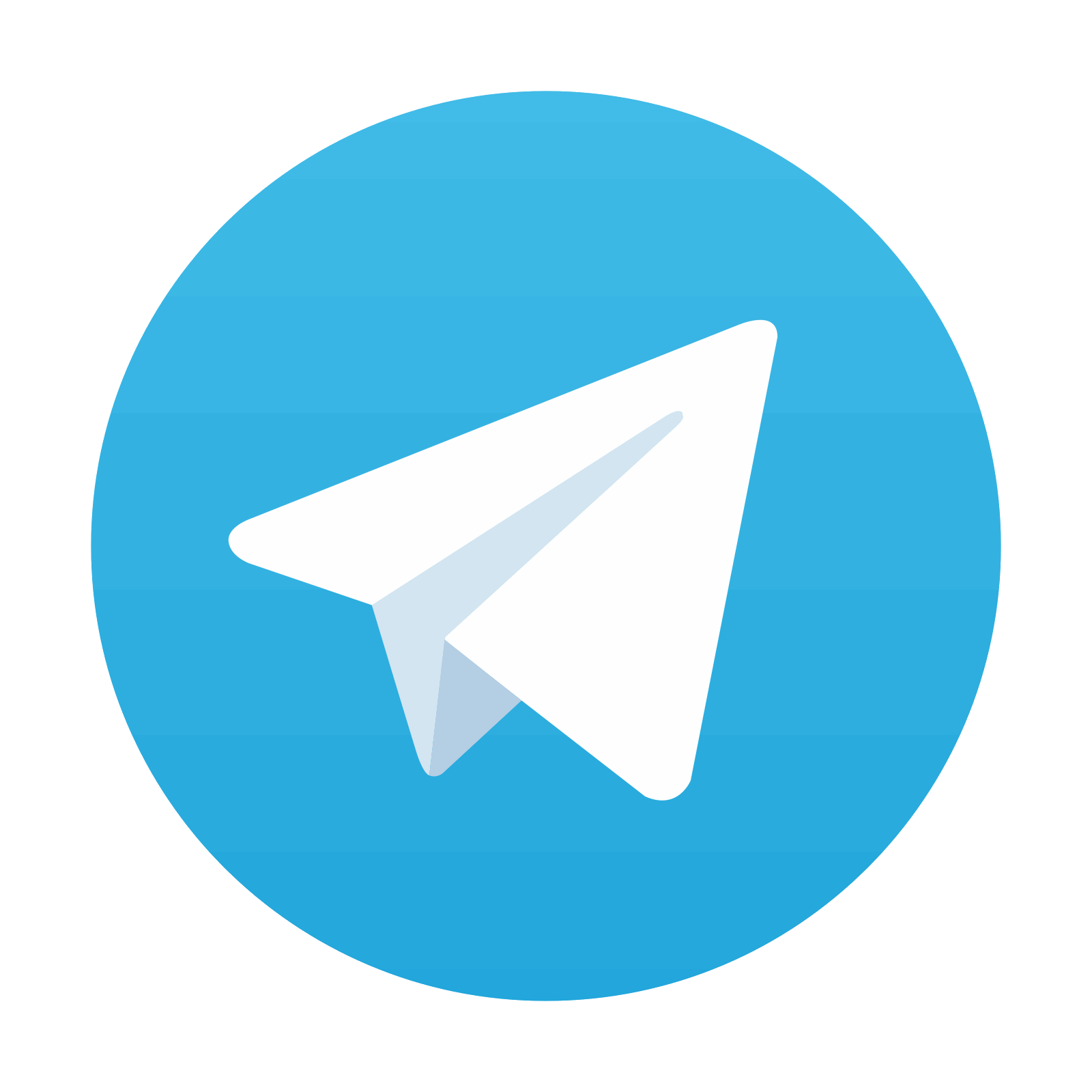
Stay updated, free dental videos. Join our Telegram channel

VIDEdental - Online dental courses
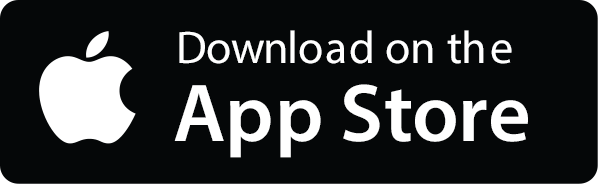
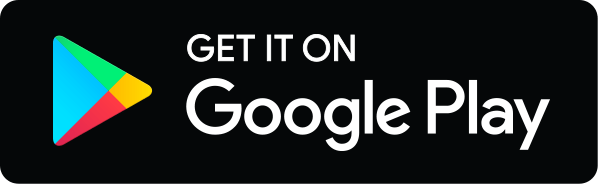