Abstract
Statement of problem
Information concerning the effect of airborne-particle abrasion before zirconia sintering on its strength after aging is lacking.
Purpose
The purpose of this in vitro study was to determine the influence of airborne-particle abrasion moment (before or after zirconia sintering), particle size, and aging on the mechanical strength of a yttria-stabilized tetragonal zirconia polycrystalline (Y-TZP) ceramic.
Material and methods
Four hundred presintered zirconia disks were allocated to nonabraded (control) and abraded groups with 50 μm or 120 μm Al 2 O 3 particles before (50/BS or 120/BS) or after sintering (50/AS or 120/AS). The disks were aged by storing them in distilled water for 24 hours at 37 °C (24 h) (control); mechanical cycling (MC) (1×10 6 cycles; 2 Hz; 100 N); hydrothermal aging (HA) (134 °C; 0.2 MPa; 20 hours); or by both methods (MC+HA), totaling 20 groups (n=20). The specimens were subjected to biaxial flexural strength (BFS) testing. Fractographic analysis was performed to identify the fracture origin. Tetragonal to monoclinic transformation was determined by X-ray diffraction. The BFS data (MPa) were analyzed by 2-way ANOVA and the Games-Howell post hoc test (α=.05). Weibull statistics was also applied.
Results
The BFS and characteristic strength (σ0) of the 120/AS groups were significantly higher compared with the other abraded groups and statistically similar or higher compared with the respective nonabraded groups. In contrast, the 120/BS/24 h and all the 50/BS groups presented the lowest values. In all groups, the initial defect had its origin on the tensile stress side. Neither airborne-particle abrasion nor aging (except for the 50/AS/MC+HA) reduced the zirconia reliability compared with the respective controls. Abrading the zirconia in its presintered stage or after sintering increased the monoclinic phase content, and the sintering process eliminated this phase.
Conclusions
Airborne-particle abrasion before zirconia sintering yields an inferior performance compared with that after sintering under aging challenges. The BFS and σ0 were strongly determined by the particle size and airborne-particle abrasion moment, while aging mainly acted in the nonabraded zirconia or when this material was abraded before sintering.
While airborne-particle abrasion before zirconia sintering yields an inferior performance, abrading the zirconia surface after sintering using coarser particles improves biaxial flexural strength without compromising the reliability of the material even under aging challenges.
The use of yttria-stabilized tetragonal zirconia polycrystals (Y-TZPs) has increased because of their biocompatibility and excellent mechanical properties. However, as this material does not contain a glassy matrix, etching with hydrofluoric acid does not roughen the surface sufficiently to provide micromechanical interlocking for adhesive bonding. Furthermore, zirconia is a relatively inert material with low surface energy and wettability, which hinders its interaction with the materials used for cementation. For these reasons, different surface treatments have been proposed to improve the zirconia/resin cement bonding mechanism. Among them, airborne-particle abrasion has been widely applied, being a straightforward and effective procedure for increasing bond strength between resin cement and Y-TZP.
In addition to providing a microretentive surface and increasing the bonding area, airborne-particle abrasion cleans and activates the surface, which favors the wettability of the zirconia surface by the luting materials. In the short term, airborne-particle abrasion has been reported to increase the strength of Y-TZP. This is a result of the tetragonal to monoclinic transformation, which is accompanied by 3% to 4% volume expansion, leading to the formation of compressive stresses that shield the cracks and prevent their growth. In the long term, the abraded zirconia, which presents surface cracks and a monoclinic phase, still suffers cyclic fatigue in the humid environment of the oral cavity. Despite these apparently adverse conditions, encouraging strength results have been reported after hydrothermal aging and cyclic loading. A systematic review with meta-analysis concluded that airborne-particle abrasion improved the flexural strength of the Y-TZP, regardless of the parameters used and whether aging was performed. However, other studies have cast doubt on the safety of airborne-particle abrasion.
Zhang et al and Guess et al observed that flaws introduced by airborne-particle abrasion compromise the fatigue strength of zirconia. Ozcan et al and Guess et al reported significantly lower reliability in abraded than in as-sintered zirconia specimens. Furthermore, some authors have reported that Y-TZP is susceptible to low-temperature degradation caused by phase transformation in a humid environment and that during the degradation it develops microcracks, which could adversely affect the strength of the ceramic. This scenario might be worse for a Y-TZP ceramic that has already developed a monoclinic phase from airborne-particle abrasion. The main concern is that when coarser particles are used, they provide increased tetragonal to monoclinic transformation than smaller particles.
Faced with this uncertainty and considering the simplicity and benefits of airborne-particle abrasion, strategies to suppress any possible damage caused by this procedure are needed. One of them might be to airborne-particle abrade before zirconia sintering as the sintering process partially heals microcracks and eliminates flaws, and the monoclinic phase associated with airborne-particle abrasion is eliminated by reverse transformation during the subsequent sintering step. A reversal in the order of procedures (airborne-particle abrasion before sintering) could directly impact the mechanical strength of zirconia. In the short term, 2 studies reported that although the flexural strength was higher when airborne-particle abrasion was performed after zirconia sintering, abrading and then sintering presintered Y-TZP provided strength similar to that of as-sintered zirconia. The absence of a monoclinic phase content is an advantage which might positively affect the long-term mechanical properties of zirconia. However, to the best of the authors’ knowledge, published studies concerning the influence of the airborne-particle abrasion moment on the mechanical strength of Y-TZP ceramic under aging challenges are lacking.
The purpose of the present study was to evaluate the influence of the airborne-particle abrasion moment (before or after zirconia sintering), particle size, and aging on the mechanical strength of Y-TZP. The null hypotheses were that airborne-particle abrasion moment, particle size, and aging would not influence the strength of Y-TZP ceramic.
Material and methods
Four hundred disks (15-mm diameter × 1.5-mm thickness) were obtained by cutting presintered zirconia blocks (Lava Frame; 3M ESPE AG) with a diamond disk (Diamond Wafering Blade, Series 15LC nº 11-4276; Buehler Ltd) in a cutting machine (Isomet 1000; Buehler Ltd) under wet conditions. The disks were washed and finished with a ceramic polisher (Exa Cerapol 0361HP; Edenta AG) with a low-speed handpiece. Subsequently, both surfaces of the disks were polished under wet conditions using 600- and 1200-grit silicon carbide abrasive papers.
The specimens were allocated into 20 groups (n=20) as seen in Figure 1 . In the nonabraded groups (control), the zirconia was sintered followed by aging. In the abraded groups, airborne-particle abrasion was performed with 50-μm Al 2 O 3 particles or 120-μm Al 2 O 3 particles (Bio-Art Equipamentos Odontológicos Ltda) before (BS) or after (AS) zirconia sintering, and then, the specimens were submitted to aging.

Airborne-particle abrasion was performed for 15 seconds with a pressure of 0.05 MPa for airborne-particle abrasion before zirconia sintering and 0.28 MPa after zirconia sintering. All specimens were ultrasonically cleaned with 99% isopropanol for 3 minutes and allowed to dry at room temperature for 24 hours. The sintering process was performed according to the manufacturer’s recommendations (heating rate=20 °C/minute: 0 °C to 1000 °C; 10 °C/minute: 1000 °C to 1500 °C; holding time=2 hours, and cooling rate=15 °C/minute: 1500 °C to 800 °C; 20 °C/minute: 800 °C to 250 °C—oven opened at 250 °C). The dimensions of the specimens after sintering were 12 mm in diameter and 1.2 mm in thickness.
The specimens were submitted to 4 experimental conditions: storage in distilled water for 24 hours at 37 °C (24 h) (control); mechanical cycling (MC): specimens were submitted to 1×10 6 cycles of biaxial flexure on a piston and 3 balls arrangement (according to ISO 6872:2008) at a frequency of 2 Hz and load of 100 N in a universal cycling machine (Dispositivo Duplicador de Forças para Pistões Pneumáticos, Biocycle; Biopdi) under immersion in distilled water at 37 °C; hydrothermal aging (HA): specimens were autoclaved at 134 °C under a pressure of 0.2 MPa for 20 hours; MC followed by HA (MC + HA), according to the parameters described.
The specimens were submitted to biaxial flexural strength (BFS) testing according to ISO 687244 for ceramic materials. The test was performed in a mechanical testing machine (EMIC DL2000; Equipment and Systems Testing Ltd) with a 10-kN load cell at a crosshead speed of 1 mm/minute. The specimens were placed on 3 steel balls with a 2.5-mm diameter and arranged at an angle of 120 degrees between them on a 10-mm-diameter support circle. The load was applied to the center of the specimen with a flat punch of 1.4 mm in diameter. BFS values were calculated according to ISO 687 244 parameters.
The fractured specimens were analyzed with a stereomicroscope (Leica EM SCD-500; Leica Microsystems GmbH) to detect the origin of the fracture. This analysis was performed according to the principles of fractography of ceramics and glasses. Representative fracture specimens (n=2/group) were analyzed with scanning electron microscopy (JSM-7500F; JEOL Ltd). The analysis was performed with magnification ×40 and ×60 and acceleration voltage of 2.0 kV.
Weibull regression analysis based on the BFS data determined both the Weibull modulus (m) and the characteristic strength (σ0) of each group. The Weibull distribution is given by the formula: P =1−exp ([−σ/σ0]m), where P is the probability of failure, σ is the BFS, σ0 is the characteristic strength at the fracture probability of 63.21%, and m is the Weibull modulus.
X-ray diffraction (XRD) analysis was performed under some baseline conditions of the Y-TZP ceramic to improve the interpretation of the results. The XRD data (n=1) for the specimens analyzed were collected in the PANalytical diffractometer (Empyrean), from 20 to 110 degrees (2θ), continuous scanning, with copper radiation (λk α 1=1.5405 Å, λk α 2=1.5444 Å, Ik α 1/Ik α 2=0.5), slits of divergence=0.5 mm and reception=0.30 mm, and tube power given by 40 kV×40 mA. For the Rietveld method refinements, the General Structure Analysis System program was used with the EXPGUI interface. The crystal structure parameter used as basis of the Inorganic Crystal Structure Database code was 66781 and 23928 (ZrO 2 , tetragonal, called t-ZrO 2 (I) and t-ZrO 2 (II), respectively), 18190 (ZrO 2 , monoclinic, called m-ZrO 2 ), and 53998 (ZrO 2 , cubic, called c-ZrO 2 ). The function used to adjust the profile was the Thompson-Cox-Hastings pseudo-Voigt. The asymmetry of reflection due to axial divergence was corrected by the model described by Finger et al. The anisotropy was corrected by the phenomenological model for microdeformation described by Stephens, and the quantification of crystalline phases was determined as described by Hill and Howard.
After analyzing the assumptions of parametric tests (Shapiro-Wilk P >.05 and Levene test P <.001), BFS data were analyzed by 2-way ANOVA followed by the Games-Howell post hoc test for multiple comparisons. The independent variables were airborne-particle abrasion (nonabrasion, 50/BS, 50/AS, 120/BS, and 120/AS) and aging conditions (24 h, MC, HA, and MC +HA). Statistical analysis was performed with statistical software (IBM SPSS Statistics, v22.0; IBM Corp) (α=.05).
Results
According to the results of the 2-way ANOVA ( Table 1 ), the interaction among the 2 factors was significant ( P <.001). Table 2 shows the BFS mean values (MPa), standard deviations, and statistical results. The groups can be allocated into 3 larger groups according to the BFS. The 120/AS groups achieved the highest BFS values. The nonabraded, 50/AS, and 120/BS groups (except for the 120/BS/24 h group) exhibited an intermediate position. Finally, the 120/BS/24 h group and the 50/BS groups presented the lowest BFS values. Compared with the 24-h control groups, aging significantly increased the BFS of the nonabraded groups and those abraded before sintering, with no influence on the groups abraded after zirconia sintering (except for the 50/AS/HA group).
Source of Variation | SS | df | MS | F | P | η 2 p |
---|---|---|---|---|---|---|
Abrasion | 17390720.716 | 4 | 4347680.179 | 323.689 | <.001 | .773 |
Aging | 1409344.562 | 3 | 469781.521 | 34.976 | <.001 | .216 |
Abrasion×aging | 1047887.115 | 12 | 87323.926 | 6.501 | <.001 | .170 |
Error | 5104033.693 | 380 | 13431.668 | |||
Total | 494844224.908 | 400 |
120/AS/HA | 1438.92 ±132.16 | A |
120/AS/MC | 1409.78 ±152.96 | A |
120/AS/24 h | 1408.62 ±229.20 | AB |
120/AS/MC+HA | 1386.94 ±153.80 | AB |
Nonabraded/HA | 1224.91 ±111.14 | BC |
Nonabraded/MC+HA | 1211.67 ±153.63 | BC |
50/AS/HA | 1181.13 ±75.21 | CD |
Nonabraded/MC | 1170.63 ±125.37 | CDE |
120/BS/HA | 1110.22 ±113.50 | CDEF |
50/AS/MC | 1099.88 ±85.63 | DEF |
50/AS/24 h | 1080.15 ±75.85 | EF |
120/BS/MC | 1030.59 ±104.97 | F |
50/AS/MC+HA | 1029.46 ±102.58 | F |
120/BS/MC+HA | 1008.60 ±133.89 | F |
Nonabraded/24 h | 992.94 ±117.73 | F |
50/BS/HA | 879.40 ±49.30 | G |
50/BS/MC+HA | 805.90 ±44.97 | H |
120/BS/24 h | 798.94 ±71.94 | H |
50/BS/24 h | 715.46 ±42.99 | I |
50/BS/MC | 692.83 ±59.02 | I |
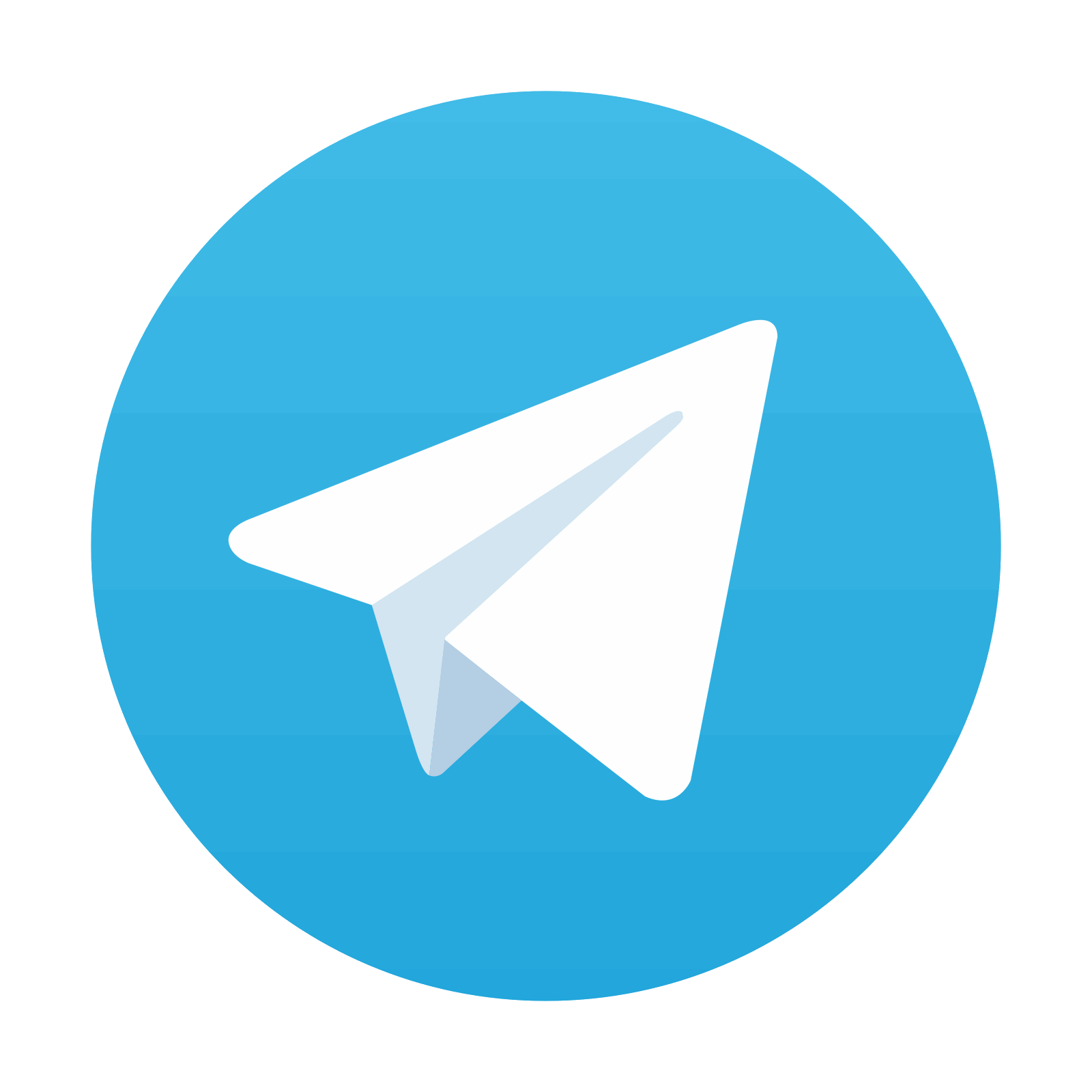
Stay updated, free dental videos. Join our Telegram channel

VIDEdental - Online dental courses
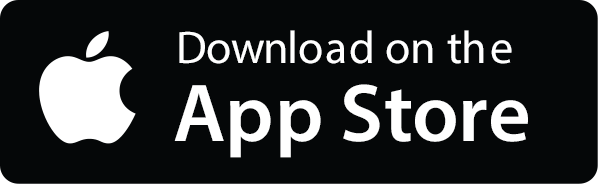
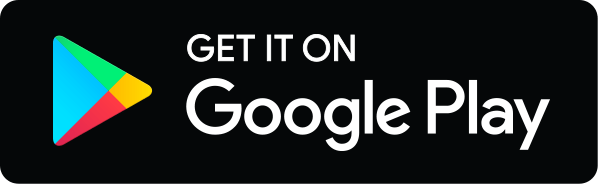
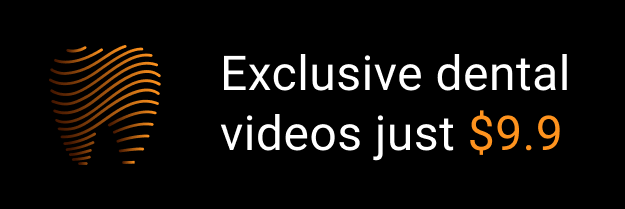