Abstract
Objectives
Dental ceramic surfaces are modified with silane coupling agents, such as γ-methacryloxypropyl trimethoxy silane (γ-MPTS), to improve bond strength. For bonding between lithium disilicate glass ceramic and resin cement, the objective was to investigate if 8-methacryloxyoctyl trimethoxy silane (8-MOTS) could yield a similar performance as the widely used γ-MPTS.
Methods
One hundred and ten lithium disilicate glass ceramic specimens were randomly divided into 11 groups (n = 10) according to pretreatment regime. All specimens were pretreated with a different solution composed of one or a combination of these agents: 10 or 20 wt% silane coupling agent of γ-MPTS or 8-MOTS, followed by a hydrolysis solution of acetic acid or 10-methacryloyloxydecyl dihydrogen phosphate (10-MDP). Each pretreated surface was luted to a stainless steel rod of 3.6 mm diameter and 2.0 mm height with resin cement. Shear bond strength between ceramic and cement was measured after 24-h storage in 37 °C distilled water.
Results
8-MOTS produced the same bonding performance as γ-MPTS. Both silane coupling agents significantly increased the bond strength of resin cement, depending on their concentration. When activated by 10-MDP hydrolysis solution, 20 wt% concentration produced the highest values (γ-MPTS: 24.9 ± 5.1 MPa; 8-MOTS: 24.6 ± 7.4 MPa). Hydrolysis with acetic acid produced lower bond strengths than with 10-MDP.
Significance
Silane coupling pretreatment with 8-MOTS increased the initial bond strength between lithium disilicate glass ceramic and resin cement, rendering the same bonding effect as the conventional γ-MPTS.
1
Introduction
All-ceramic restorations have become increasingly popular with dentists and patients because of functional and aesthetic excellence. Not only do all-ceramic restorations circumvent issues pertaining to metal biocompatibility , they provide superior aesthetics . High glass content provides indisputable aesthetic appeal, while high crystalline content of alumina, lithium disilicate and zirconia provides high strength . In the case of lithium disilicate glass-ceramic, it contains 70% of lithium disilicate crystals in a glassy matrix, thus rendering them better than other ceramic materials with the desirable qualities of low refractive index, high translucency and enhanced strength . Coupled with their progressively improved mechanical properties, all-ceramic treatment options have expanded to include inlays, onlays, veneers and crowns.
Ceramic-resin cement bonding is a perennially critical concern because it has to withstand the harsh conditions of the oral environment as well as functional forces present in the oral cavity. The current practice of achieving effective, durable bonding is by two mechanisms: micro-mechanical attachment provided by acid etching or air abrasion, and chemical bonding by silane coupling agents. Silane coupling agent with the general formula R (CH 2 ) n Si (OR′) 3 has been widely used to increase bond strength , since mechanical attachment alone is not sufficient to withstand the intra-oral conditions for long-term use.
Organo-functional terminal (R) groups and hydrolysable groups (R′) are capable of achieving strong bonding with silica and resin . After the hydrolysable groups are hydrolysed by acid, reactive intermediate silanol groups (Si OH) are formed. Next, oxane bond (Si O Si) is formed between the silane agent and silica surface to create the chemical bonding between the ceramic and resin cement.
Instead of a monolayer of silane coupling agent being produced on the ceramic surface to bring about the optimal bond strength, a multilayer comprising three different structures is reported to be formed on the silane-treated surface . The outermost layer consists of small physisorbed oligomers, while the innermost layer consists of a regular three-dimensional network uniformly supported by and bonded to the ceramic surface. Between these two layers, there exist oligomers partially connected with a few siloxane bonds . Therefore, the multilayer is derived from the condensation reaction of silane coupling agent, hydrogen bonds of carbonyl groups, and van der Waals interactions of hydroxyl groups .
In silane coupling agents, the hydrocarbon chain ( (CH 2 ) n ) acts as a spacer between two functional groups. This hydrocarbon spacer influences myriad monomer properties, such as flexibility, solubility, wetting characteristic and hydrophobicity–hydrophilicity balance. Longer hydrocarbon chains, such as (CH 2 ) 3 in the widely used γ-methacryloxypropyl trimethoxy silane (γ-MPTS), produce greater hydrophobicity . Therefore, unlike short hydrocarbon chains, long hydrocarbon chains could offer the enhanced protection of self-condensation of silane coupling agents, thereby generating dimers, trimers and oligomers which have greater solubility.
On a glass surface, silane depositions with longer hydrocarbon chains offer higher density and stability than those with shorter hydrocarbon chains. In this study, we investigated and compared the chemical interaction potential of 8-methacryloxyoctyl trimethoxy silane (8-MOTS) with lithium disilicate glass-ceramic against that of γ-MPTS, where 8-MOTS has a longer hydrocarbon chain than γ-MPTS. The null hypothesis tested was that the bonding performance of 8-MOTS would not differ from that of γ-MPTS, which is conventionally used in dental practice.
2
Materials and methods
One hundred and ten lithium disilicate glass ceramic specimens (IPS e.max CAD, Ivoclar Vivadent AG, Schaan, Liechtenstein; Table 1 ) were randomly divided into 11 groups (10 specimens per group) according to surface treatment method. Before surface treatment, all ceramic surfaces were polished with abrasive paper (Silicon Carbide abrasive paper, #2000, Struers A/S, Rodovre, Denmark) under water irrigation. Polished ceramic surface was treated with a silane coupling agent of either γ-MPTS or 8-MOTS in two concentrations (10 or 20 wt%) in ethanol ( Fig. 1 , Table 2 ). Silane coupling solutions were activated by a hydrolysis solution of either 2 wt% 10-methacryloyloxydecyl dihydrogen phosphate (10-MDP) or 2 wt% acetic acid (AA). Two other controls of non silane-treated specimens applied with 10-MDP or AA alone were also prepared, as well as a negative control subjected not to any surface treatment.
Materials | Ingredients (in % by weight) | Manufacture |
---|---|---|
Litium discilicate ceramic | IPS e.max CAD; SiO 2 (57.0–80.0), Li 2 O (11.0–19.0), K 2 O (0.0–13.0), P 2 O 5 (0.0–11.0), ZrO 2 (0.0–8.0), ZnO (0.0–8.0), Al 2 O 3 (0.0–5.0), MgO (0.0–5.0), Colouring oxides (0.0–8.0) | Ivoclar Vivadent AG (Schaan, Liechtenstein) |
Silane solution | γ-MPTS (10.0 or 20.0) in ethanol | Shin-Etsu Chemical (Tokyo, Japan) |
8-MOTS (10.0 or 20.0) in ethanol | ||
10-MDP solution | 10-Methacryloyloxydecyl dihydrogen phosphate (2.0) in ethanol | Kuraray Noritake Dental (Tokyo, Japan) |
Acetic acid solution | Acetic acid (2.0), ethanol (70.0), water (28.0) | Wako Pure Chemical Industries (Osaka, Japan) |
Resin cement | Clearfil Esthetic Cement Universal; A paste: Bis-GMA, TEGDMA, Hydrophobic aromatic dimethacrylate, silanated barium glass, colloidal silica, acceletors |
Kuraray Noritake Dental (Tokyo, Japan) |
B paste: Bis-GMA, TEGDMA, hydrophobic aromatic and aliphatic dimethacrylates, silanated silica, silanated barium glass, colloidal silica, CQ, initiators, accelerators, pigments |

γ-MPTS (10%) | γ-MPTS (20%) | 8-MOTS (10%) | 8-MOTS (20%) | NA | |
---|---|---|---|---|---|
AA | 11.58 (6.0) b | 11.86 (8.2) b | 13.01 (5.6) bc | 9.09 (5.9) ab | 1.43 (0.8) a |
10-MDP | 20.15 (4.7) cd | 24.93 (5.1) d | 20.73 (6.3) cd | 24.58 (7.4) d | 3.24 (2.4) a |
NA | 2.28 (1.4) a |
2.1
Fourier transform infrared spectroscopy (FTIR) analysis
Silane coupling agent activated by hydrolysis solution was evaluated using FTIR (IRAffinity-1S, Shimadzu, Japan) in transmission mode. Immediately after mixing with a hydrolysis solution, each silane coupling agent of either two concentrations (10 or 20 wt%) was placed on a KBr plate. An even film was obtained with a second KBr plate placed on top. Peak analysis was done at 2835 cm −1 to reveal the carbon hydrogen stretching vibrations.
2.2
Bonding procedure
After a gentle blow-dry with oil-free air, a stainless steel rod (3.6 mm diameter, 2.0 mm height) was luted onto pretreated ceramic surface using an auto-mixed, dual-cure, and non 10-MDP-containing adhesive resin cement (Clearfil SA Cement, Kuraray Noritake Dental, Tokyo, Japan; Table 1 ). Cementation was carried out using a Teflon jig mold, where a 10-N load was applied and maintained for 3 min. Bonded specimens were light-cured for 20 s on each side from two opposite sides. Each adhesive surface of stainless steel rod was air abraded with 50-μm aluminum oxide particles and then applied a metal primer (Alloy primer, Kuraray Noritake Dental, Tokyo, Japan) in order not to occur the adhesive failure between stainless steal rod and resin cement.
2.3
Shear bond strength test
All bonded specimens were immersed in distilled water (37 ± 2 °C) for 24 h before shear bond strength test. Each specimen was placed in a shear test fixture, and shear bond strength was measured using a universal testing machine (Autograph AG-X, Shimadzu, Kyoto, Japan). Stress at failure was automatically calculated and recorded as the shear bond strength using an accessory software.
After debonding, the fractured surfaces of ceramic specimens were examined under a light microscope. Failure caused by shear fracture was classified into one of the following three types: (1) adhesive failure between resin cement and ceramic; (2) cohesive failure within resin cement; or (3) mixed-mode failure (adhesive–cohesive).
Shear bond strength data of each group of lithium disilicate glass ceramic specimens were statistically compared using one-way analysis of variance (ANOVA) and Tukey HSD post hoc test within 5% error limits ( p < 0.05) using the Statistical Package for the Social Sciences (IBM SPSS Statistics).
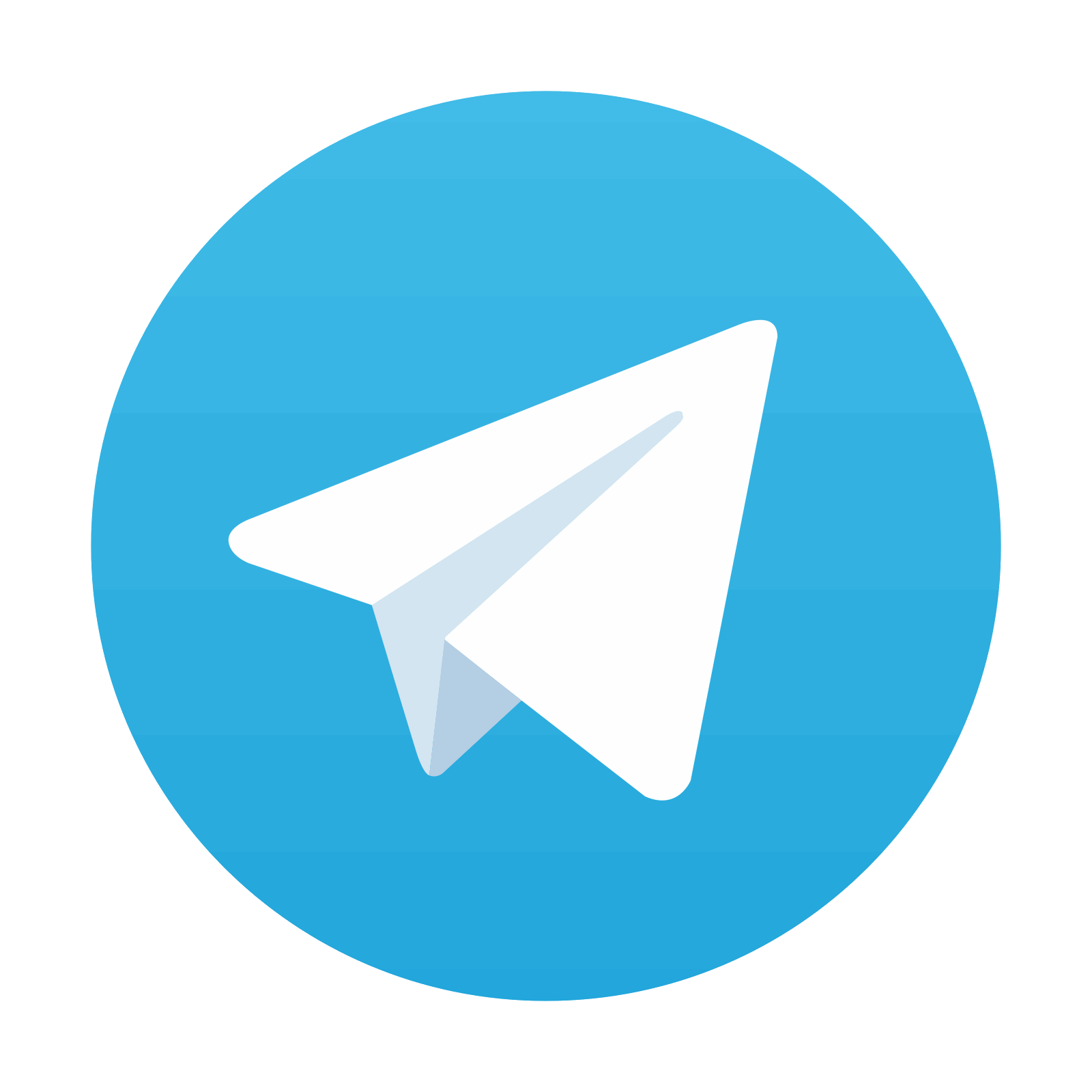
Stay updated, free dental videos. Join our Telegram channel

VIDEdental - Online dental courses
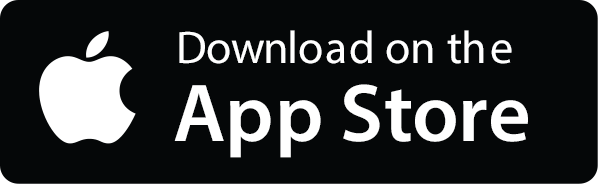
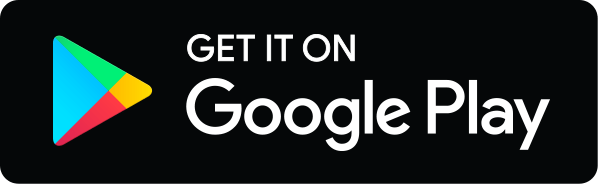