12
Digital Integration of Implant Surgery Workflow
Jeremy Kernitsky and Massimo Di Battista
Basic Concepts in Digital Dentistry
The origins of digital dentistry can be traced to almost 40 years ago, when the first dental restoration was milled using computer aided-design (CAD) and computer aided-manufacturing (CAM) by the first commercial CAD/CAM system: CEREC (Dentsply, Sirona) (Mörmann 2006). Since then, many advancements have been made in digital dentistry, but most of the basic principles stand the test of time. Most workflows in digital dentistry start from a process known as digitization, which consists of transporting diagnostic information into the digital world through various modalities. For instance, bone structures can be digitized by obtaining a cone-beam computed tomography (CBCT) providing a Digital Imaging and Communications in Medicine (DICOM) file, while teeth and soft tissue can be digitized by a variety of optical scanners to create a jaw replica in the form of a stereolithography (STL) file.
The digitized files have different functions and properties during the CAD-CAM process. For example, a DICOM file is a tridimensional volume rendering of an area of interest and its resolution is determined by the voxel size (Bankman 2000). This tridimensional visualization is nothing but a hologram and does not contain digital information on the surface geometry of the bone. In other words, this means that the boundaries of the imaged anatomical structures are not registered in the digital world. This type of imaging study (DICOM) is useful for diagnostic purposes, such as bone volume measurement and screening for pathology. However, due to the lack of surfaces in this type of file, it cannot be modified or integrated into a digital workflow without first identifying and extracting surfaces through segmentation.
An STL file, which is another type of 3D file, represents a surface and can be modified. Due to their versatility and the fact that they can be modified, STL files are used extensively in digital dentistry.
Segmentation is a process that allows obtaining an STL file from the original DICOM file. It is generally defined as the identification of all the voxels (or pixels) that belong to the object of interest or its boundary. Among the many existing segmentation methods, the preferred one is called “threshold segmentation.” As implied by the name, threshold segmentation involves separating the object of interest (bone and teeth) from the rest of the volume (soft tissues and background) utilizing a cutoff radio-opacity value: the threshold (Bankman 2000). Some digital implant software will not let users set a specific threshold value, instead providing them with predetermined settings corresponding to different thresholds (e.g., bone, soft tissue, endo, etc.), while other software will let the user decide of the threshold themselves. Figure 12.1 represents different thresholds of the same CBCT.

Figure 12.1 CBCT Thresholding. Different thresholds are applied to the same CBCT dataset. (A) represents a too low thresholds; therefore, soft tissue and artifacts are visible. (B) represents an adequate threshold; the surface created represents mostly hard tissue. (C) represents a too high threshold; major parts of the hard tissue are missing.
Another important concept is superposition, which is defined as the process of placing an object over another. In the context of implant dentistry this is mostly done to superimpose an intraoral scan (or a scan of an impression) to the CBCT. This process is also referred to as “merging.” This is beneficial because intraoral scans have a better resolution and fewer artifacts than CBCT images, especially for dental structure. The image of the dentition provided by a CBCT is not accurate enough to fabricate a surgical template that will precisely fit on teeth. By superimposing both files, the clinician gets the best of both worlds, high accuracy and anatomy visualization. Merging the scans also adds diagnostic value because it allows for more accurate visualization of the soft-tissue thickness. Unless the CBCT was acquired with cheek retractors in place (Januário et al. 2008), the facial soft tissue of the patient will most likely be contacting the cheeks at time of acquisition and will therefore be indistinguishable from them on the resulting imaging study. When the intraoral scan is overlayed atop the CBCT, the thickness of the soft tissue becomes evident (Figure 12.3).

Figure 12.3 Superimposition of intraoral scan and CBCT. (A)The STL surface of the intraoral scan (green) superimposed over the surface extracted from the CBCT (gray). (B) The outline of the intraoral scan (green) backwardly superimposed to the CBCT (grayscale). The tight adaptation of the outline around the tooth indicates a successful merge. Note that the soft tissue thickness is perceivable (distance between green line and bone).
The STL files cannot be directly superimposed to the DICOM files; a surface must be extracted from the DICOM first. Most modern implant planning software will do this automatically for the clinician. Once the STL of the intraoral scan has been superimposed to the surface extracted from the DICOM file, the intraoral scan will be backwardly superimposed to the DICOM. In order to minimize the introduction of error in the treatment plan, it is primordial to ensure that the merging of the two surfaces is done accurately. The most common way of doing this for dentate patients is by selecting and matching multiple reference points on the DICOM and the intraoral scan as shown in Figure 12.2. The relation of both scans can then be compared by evaluating the adaptation of the outline of the intraoral scan to the anatomical structure of the CBCT as shown in Figure 12.3.

Figure 12.2 The merging procedure. Reference points are highlighted on the STL surface of the intraoral scan (right) and a reconstructed panoramic image (left). The software then uses an algorithm to match both and gives the user the option to verify the merge. While the concept remains the same, other software systems have different ways of doing this, for example matching points to a 3D reconstruction of the CBCT instead of a panoramic image. To ensure a successful merge, it is recommended to have points on both sides of the arch.
Once the CBCT dataset is merged with the STL of the intraoral structures, the clinician can start planning for the implant treatment plan. Having the CBCT and the intraoral scan allows all the steps of implant planning, such as the digital wax-up, the surgical planning, and the design and manufacture of a surgical guide.
Surgical Planning: From Analog Static to Computer Generated Guides, Dynamic Surgery (Navigation and Robotic Placement)
Digital Diagnostic Wax-up
One of the most notable paradigm shifts in modern implant dentistry is the “restoratively driven” concept. This is a treatment philosophy based around the fact that the only purpose of dental implants is to support a restoration, therefore the implant position should be mainly driven by the restorative plan and not the anatomy of the patient (Orentlicher et al. 2012). The surgical-restorative team must start with the end result in mind, i.e., the planned restoration, and the fixture(s) should be placed in the ideal position according to this plan.
From that perspective, the first step of implant planning, after patient assessment and interview, should be the diagnostic wax-up. Early protocols involved mounting casts, carving a physical wax-up, and subsequently scan it to merge with the CBCT (Kourtis et al. 2012). Later, fully digital protocols where a tooth is selected from a premade tooth bank and added onto the STL file of the intraoral scans were described (Lee et al. 2012). Although this method had the advantage of being fully digital, the fact that teeth were from predetermined shapes meant that occlusion was not accounted for in the planning.
With modern advances in computer-assisted design and manufacturing (CAD/CAM), the planning, design, and manufacture of dental restoration are now routinely in most dental offices and educational institutions (Mörmann 2006). The technology used for these can be applied for the planification of ideal restorations for implant therapy. Dano et al. (2018) describes a fully digital workflow for restorative design and implant planning, as shown in Figure 12.4. This technique has the advantage of both being fully digital and taking occlusion in consideration.

Figure 12.4 From diagnostic wax-up to implant placement. (A) The intraoral scan with the approximate position of the CEJ traced. The digital wax-up is then computed and shown in (B). The implant planning based on these restorations is depicted in (C) and (D). This is done in the implant planning software and the CBCT is examined to confirm that the anatomy allows this placement. (E) and (F) represent the surgical procedure.
For edentulous patients, since soft tissue is not a reliable indicator for the merging process (as it is not captured accurately in the CBCT) the ideal restoration can be merged to the 3D radiographic images through a process known as a dual scan. This process consists of obtaining a CBCT of the patient wearing an ideal denture that is embedded with radiopaque markers. Subsequently, another CBCT of the denture alone is captured. Two different DICOM files (one of the patient with the denture and one of the denture alone) are exported. The radiographic markers are then used to superimpose both images. This allows for visualization of the ideal denture teeth position in relation to the bone. A surface (STL file) can then be extracted from the denture scan and used as a framework for a surgical guide.
Currently, a multitude of guidance systems in dental implantology are available. They can be divided in two major categories: static and dynamic guidance. Static guides are characterized by the use of a physical template based on the digital plan, which is usually made of an acrylic material. This plan cannot be modified, and its accuracy is affected by multiple factors including but not limited to: planning software, distortion of the initial intraoral scan (STL) or DICOM, process of manufacturing of the guide, and most importantly the supporting or stabilization method of the guide intraorally (Ozan et al. 2009; Tahmaseb et al. 2018). Literature shows that tooth-supported guides usually offer superior precision over bone supported or mucosa supported (Ozan 2009). Surgical guides can be designed to be used for every step of the surgical procedure (fully guided) or to be used for one or some of the drills only (partially guided). As a rule of thumb, given the guide is fitting properly, fully guided surgery will be more accurate than partially guided surgery. It is also important to keep in mind that partially guided surgery is still significantly more accurate than free-hand surgery (Younes 2018). Static guides are the most common guidance method used but are subject to inaccuracy of on average 0.9 mm (up to 2 mm) and 2 degrees if a fully guided protocol is followed (Younes et al. 2018).
Static guides usually present as perforated arch-shaped templates. The perforations allow osteotomy drills and implants to pass in a restricted fashion, therefore limiting the range of motion of the operator to the desired position of the implant. These perforations are sometimes fitted with metallic sleeves to increase rigidity and further limit the range of motion. Usually, the sleeve will be of the diameter of the largest drill or of the implant attachment. Adaptors with the same external diameter as the sleeve, but with a smaller internal diameter also known as “drill keys” or “drill spoons” will be fitted inside the sleeves during osteotomy preparation to accommodate smaller drills and guide the entirety of the surgery.
Dynamic guidance consists of installing a fixture through either a navigation system or robotic guidance. These both allow for intraoperative modification of the surgical plan, hence the name “dynamic.” In general, both methods will allow for a full digital workflow without the need for a physical guide. This eliminates the main drawback of physical guides, which is the inability to perform the surgical procedure immediately after planning (because of the time needed to manufacture the guide). Furthermore, not having a physical guide also allows for better access to the surgical site, facilitating visualization and irrigation.
Based on available evidence, both methods can achieve levels of accuracy similar to fully guided static guides, if not more. However, as these technologies are fairly new and are still being developed, more evidence is required (Bolding et al. 2021; Rawal et al. 2022; Stefanelli et al. 2019). That said, to this date there is no system that is 100% accurate. Consequently, the surgeon must still verify the accuracy of the guidance system throughout the implant surgery.
Static Implant Guidance
Tooth-borne Guide
Tooth-supported templates are the most accurate type of static guides (Dawson et al. 2009). They are therefore preferred when the conditions allow for their use. The surgical template must be stable and not present any deformation while under pressure in order to provide adequate precision for the implant placement. Caution must be exerted while treating a large edentulous area or a distal end. It has been shown that the quality and the location of teeth supporting the surgical guide influence the accuracy. However, for single implant sites, there is no benefit in covering more than four teeth adjacent to the surgical site or more than three posterior teeth. Including more teeth might increase the chances of misfit. Span of the guide for longer edentulous areas need to be evaluated in a case-by-case fashion (El Kholy et al. 2019).
Most digital implant software suites have an extension for the design of surgical templates. After the implant planning, the clinician will need to select a sleeve diameter and position based on the case and implant type. Then, the perimeter of the guide has to be selected. Care must be taken not to include too much soft tissue, as this can interfere with the stability of the guide. Retention must also be adequately assessed, as a too retentive guide might not sit, and a poorly retentive guide might move during the surgical procedure. Once the template is designed, it can then be manufactured in-house via either milling, 3D printing, or outsourced to a third party.
Figure 12.5 shows an immediate implant installed with a tooth-supported template. In immediate maxillary implant cases, the use of a guide is preferred because it reduces facial drift of the implant during placement compared to freehand surgery (Chen et al. 2018). A facially positioned implant might be detrimental to the overall success of the case because it can prevent the use of a screw-retained restoration or can result in the screw hole being in an unaesthetic position. The use of guided surgery also allows the preoperative preparation of a provisional restoration to be “picked up” after the placement, such as in Figure 12.5.

Figure 12.5 Immediate implant placement with tooth-supported template. (A), (B), and (C)The atraumatic extraction of a non-restorable vertically fractured central incisor. (D) and (E) The surgical guide in situ. (F) The implant in final position. (G) and (H) The provisional restoration. (I) Immediate outcome. (J) 2 weeks post-surgery. Courtesy of Dr. Elias Exarchos.
This workflow can also be modified for the template to be supported by prepared crown or bridge abutment teeth. In some cases of full-arch rehabilitation, the edentulous spaces can be temporarily restored by provisional bridges. To do so, the abutment teeth must be reduced before implant placement. If the provisional restorations are made with a radiolucent material, e.g., polymethylmethacrylate (PMMA), they will not be captured by the CBCT, therefore merging with an intraoral scan of the provisional teeth will be impossible. To address this, it is possible to merge the CBCT with an intraoral scan of the prepared abutment teeth, leading to the fabrication of an abutment teeth-supported guide. This also has the advantage of allowing the making and modifying of a new digital wax-up based on the abutment teeth and therefore gives more flexibility to the clinician. Figure 12.6 shows a scan that has been obtained with a PMMA provisional restoration, and Figures 12.7 and 12.8 show the workflow of a prepared teeth-borne surgical guide.

Figure 12.6 Merging CBCT with scan of prepared teeth. This CBCT was captured with a PMMA provisional restoration. Note that the provisional is not visible on any of the images due to its radiolucency. (A)3D reconstruction of the CBCT. (B) 3D reconstruction of the CBCT with the merged scan of abutment teeth. (C) 3D reconstruction of the CBCT with the merged scan of abutment teeth and a digital wax-up. (D), (E), and (F) Cross-sectional views of A, B, and C.

Figure 12.7 Abutment teeth-borne surgical guide. (A)The digital wax-up with implant planning. (B) The implant planning on the digital cast of the abutment teeth. (C) The designed surgical guide. (D) The provisional restoration made with the digital wax-up. (E) Prepared teeth and edentulous areas. (F) The surgical guide in situ.

Figure 12.8 Immediate implant placement with abutment teeth-borne surgical guide. (A) Provisional restoration in place preoperatively. (B) Tooth preparations exposed and cleaned after removal of provisional restoration. (C) Atraumatic extraction of the maxillary lateral incisor. (D) Surgical guide in situ. (E) Final implant position. (F) Site closed with collagen matrix after bone grafting.
Mucosa-supported Guide
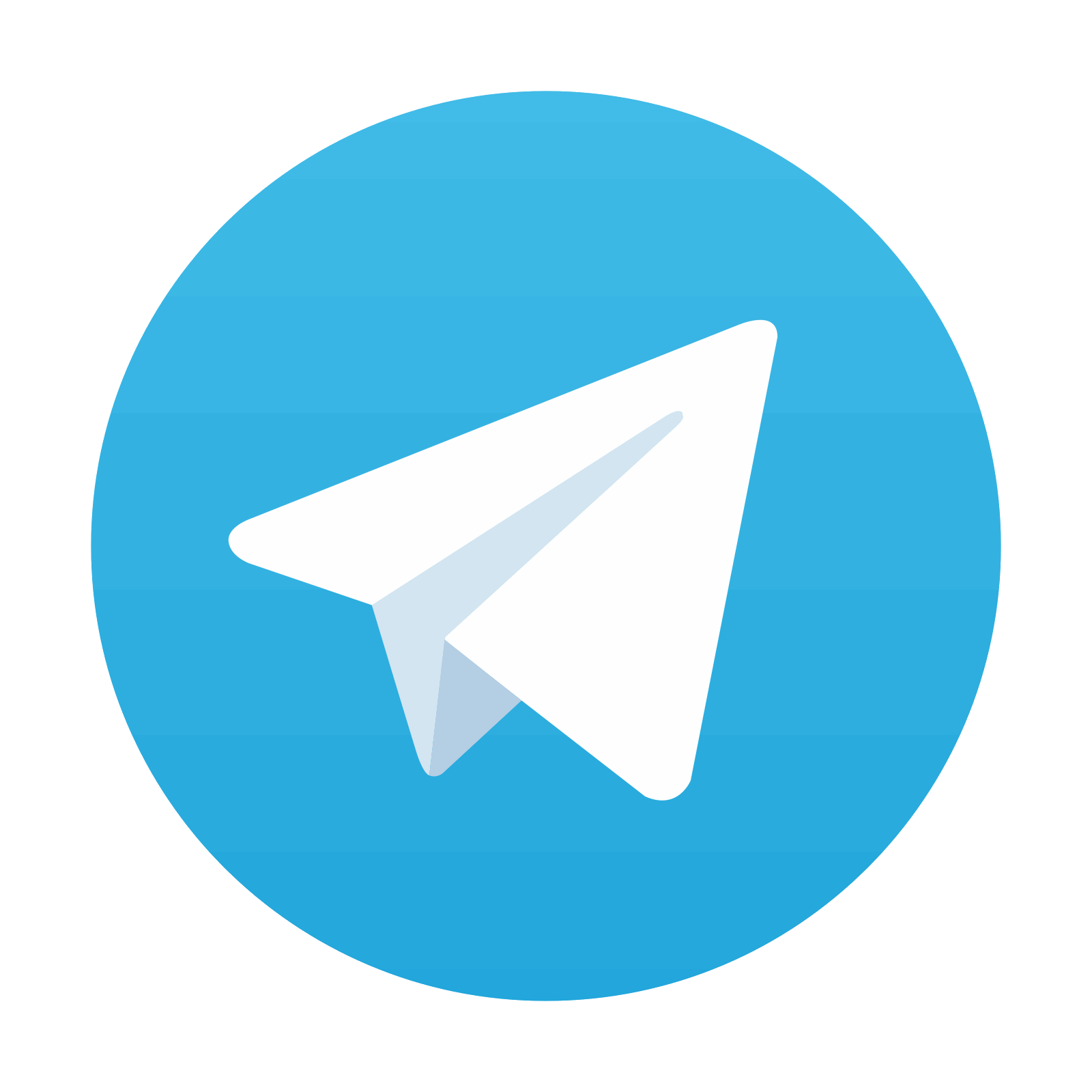
Stay updated, free dental videos. Join our Telegram channel

VIDEdental - Online dental courses
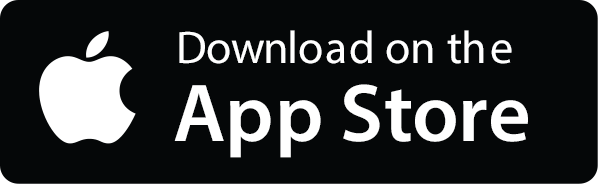
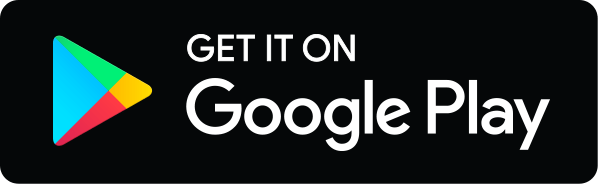