Introduction
The success of maxillary expansion should not be assessed solely by the elimination of the teeth in crossbite; it should also be defined as the reestablishment of normal maxillary growth. The aim of this research was to quantify longitudinal palatal changes in children treated for maxillary constriction associated with functional crossbite.
Methods
The subjects included 48 white children (mean age, 5.2 ± 0.6 years), divided into a treatment group ( n = 23) and a control group ( n = 25). Children in the treatment group had maxillary constriction associated with functional crossbite and were treated with a cemented acrylic splint expander. Dental casts were collected at baseline and at 6, 12, 18, 30, 42, and 54 months later. The casts were scanned with a laser scanner, and the palatal surface areas and volumes, and their increments over time were calculated. Nonparametric tests were used for the data analysis. The diagnostic performance in assessing successful treatment of palatal constriction was evaluated by receiver operating characteristic curves.
Results
Significantly greater increments in palatal surface area and volume were seen in the treatment group up to 30 months ( P <0.05, at least). According to the receiver operating characteristic curves, the best overall diagnostic performance in terms of accuracy was for palatal volume at 18 months, reaching up to a value of 0.85, by using a cutoff value of increments of 13.5%.
Conclusions
An increase in palatal volume of at least 13.5% at 18 months after treatment is a good indicator to assess the reestablishment of normal growth in subjects treated for maxillary constriction in the deciduous dentition.
Over the last decade, several 3-dimensional (3D) recording methods have become more widely used in dentistry, including invasive (eg, cone-beam computed tomography) and noninvasive (eg, laser scanning) procedures. Invasive methods have been used to assess the skeletal effects of active maxillary expansion in 3 dimensions. On the other hand, noninvasive 3D recording methods have been used to monitor growth of the palatal vault in subjects with a normal maxilla or a constricted maxilla or to assess the treatment effects of maxillary expansion.
The success of expansion treatment of a maxillary constriction is frequently assessed only by the elimination of the teeth in crossbite. However, in growing subjects, this success should also include reestablishment of the normal growth rate on a longitudinal basis. Ideally, a diagnostic tool for the assessment of successful maxillary expansion must also incorporate the changes from normal development in growing subjects. Moreover, in a clinical setting, a noninvasive method should be preferred.
Recent studies that have assessed maxillary expansion effects by using 3D scanning have reported that, after active expansion, there is a relevant palatal volume change. In this regard, another longitudinal study that used the same methodology reported that the palatal surface area varies most during normal growth in the prepubertal period. However, no previous study based on 3D digital models has evaluated the diagnostic performance of the parameters examined for the assessment of successful maxillary expansion.
In particular, in a clinical setting (where no control group is available), the assessment of successful maxillary expansion on consecutive longitudinal recordings can be based on a given diagnostic parameter, as long as a reliable cutoff value is available. Another issue relates to the optimal timing at which the best diagnostic performance can be expected after maxillary expansion.
In consideration of these issues, the aim of our controlled study was to determine whether palatal surface areas and volumes measured by laser 3D scanning can provide satisfactory diagnostic performance for assessment of treatment success of palatal constriction. The ultimate goal is to provide a noninvasive diagnostic tool for the assessment of the success of maxillary expansion on an individual basis in growing subjects.
Material and methods
Approval for this study was granted by the institutional review board of the Slovenian National Medical Ethics Committee, with signed informed consents from the parents of all subjects obtained before the study. A total of 48 white subjects, aged 4.1 to 6.6 years (mean, 5.2 ± 0.6 years), were included. The treated group consisted of 23 subjects (15 girls, 8 boys; mean age, 5.1 ± 0.8 years) with a constricted maxilla associated with a unilateral posterior crossbite. Only subjects in the deciduous dentition with all posterior teeth in crossbite on 1 side and a midline deviation caused by a functional mandibular shift of at least 2 mm were included. The functional mandibular shift was assessed clinically by an experienced orthodontist (M.O.). These subjects were selected from a group of patients who had no relapse after treatment and were included in a previous study. Treatment success was assessed clinically at each time point, and relapse was considered when at least 1 tooth in the dental arch was in crossbite, in either the posterior or anterior region, including edge-to-edge relationships. A control group of 25 subjects (12 girls, 13 boys; mean age, 5.3 ± 0.2 years) without malocclusion was also included; these subjects were randomly selected from a local kindergarten. None of them had respiratory, deglutition, or masticatory problems and had no previous or concomitant orthodontic treatment. This sample belonged to a larger group of subjects for which details have been published previously.
The treatment group had maxillary expansion with an acrylic plate and a midline screw. The acrylic plate with the biteplate was cemented onto the maxillary deciduous molars. The screw was activated by 0.25 mm every 2 days for 4 weeks, and overexpansion of the maxillary arch was achieved in all subjects. The plate was left in place for the next 4 weeks without activation. The biteplate was removed, and the acrylic plate was then used as a removable retainer for a further 4 months. Therefore, the expected expansion in the treatment group was approximately 3.5 mm, and active therapy was followed by about 4 months with the retainer. Treatment success was evaluated at follow-ups 6, 12, 18, 30, 42, and 54 months later. Relapse was considered if at least 1 tooth was in crossbite, in either the posterior or anterior region.
In all subjects, impressions of the dental arches were taken at baseline (before treatment in the treatment group) and 6, 12, 18, 30, 42, and 54 months later. At each time point, clinical assessments of malocclusion and orofacial functions of all subjects were also performed.
The study casts were scanned at a distance of 60 cm with a scanner (Vivid 910; Konica Minolta Optics, Inc, Tokyo, Japan) by using a lens with a focal distance of 25 mm. With this lens, the scanner has a reported accuracy of 0.22 mm. The scanning time for all study casts with this scanner would be approximately 5 minutes; however, only the parts of interest of the cast were scanned to shorten the process. If the scanner is calibrated properly, there is no need to repeat the scanning process.
Each scan of a study cast was preprocessed to remove unwanted data. The preprocessing of the digital models, although it is fully automated, can take another 3 to 5 minutes. To measure the palatal surface area and calculate the palatal volume, the boundaries of the palate had to be defined. The gingival plane and a distal plane were used as boundaries for the palate. The gingival plane was created by connecting the midpoints of the dento-gingival junctions of all deciduous teeth. The distal plane was created through 2 points at the distal part of the second deciduous molars, perpendicular to the gingival plane ( Fig 1 ). The palatal surface area and the volume were then calculated.
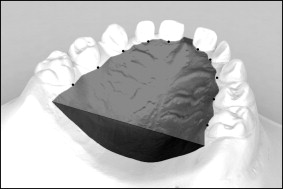
A sample size of at least 17 subjects per group was necessary to detect an effect-size coefficient of 1.0 for either palatal parameter (surface area or volume) in any comparison between the groups, at each time point, with an alpha set at 0.05 and a power of 0.8. The effect-size coefficient is the ratio of the difference between the recordings of the 2 groups, divided by the within-subject standard deviation. An effect size of at least 0.8 is regarded as a large effect, and a value of at least 1.0 is associated with good diagnostic potential.
The method error for each palatal parameter was calculated by using intraclass correlation coefficients on a random sample of 10 replicate measurements. To quantify the full method error of the recordings for both palatal parameters, the method of moments variance estimator was used. Therefore, the mean error and the 95% confidence intervals between the repeated recordings were calculated by using the method of moments variance estimator, expressed as percentages. The method of moments variance estimator has the advantage of not being affected by an unknown bias (ie, systematic errors) between pairs of measurements.
Statistical analysis
SPSS software (version 13.0; SPSS, Chicago, Ill) and Comprehensive Meta-Analysis (version 2; Biostat, Englewood, NJ) were used for the statistical analyses. The balance of the experimental groups according to age and sex was tested with the Student t test and the Fisher exact test, respectively.
To analyze the changes in the palatal parameters, the normalized changes (ie, increments) in the palatal surface areas and volumes were calculated and expressed as percentages of the corresponding baseline values. After testing the normality of the data with the Shapiro-Wilks test and the equality of variance among the data sets by using the Levene test, nonparametric methods were used for data analysis. The Friedman test was used to test the significance of the differences of these increments in either palatal parameter over time in each group. The Mann-Whitney U test was used to assess the significance between the 2 groups in each time point. For both palatal parameters, the proper statistical power for the normalized changes was assessed by calculation of the rho correlation coefficients between the nonnormalized baseline values and the corresponding data sets recorded at the subsequent time points. A threshold of 0.65 was considered acceptable for using normalized changes without a relevant loss of statistical power.
Moreover, for these increments of both palatal parameters, the actual effect-size coefficients along with the 95% confidence intervals were calculated as indexes of potential diagnostic accuracy at each time point between the groups, as previously described. Here, a threshold of 1.0, including the full confidence interval, was used to assess potentially good diagnostic accuracy.
The diagnostic performance in the assessment of the successful treatment of the palatal constriction was evaluated by using receiver operating characteristic curves. A receiver operating characteristic curve is obtained by calculating the sensitivity and specificity of every observed data value and plotting sensitivity against 1−specificity. A global assessment of the performance of the test is given by the area delimited, ie, under the curve. This area is equal to the probability that a random person with a given condition has a higher (or lower) value of the measurement than a random person without that condition. An area under the curve of 0.5 is an uninformative test, whereas a value equal to 1.0 indicates a perfect diagnostic test. Therefore, receiver operating characteristic curves for both palatal parameters were computed at each time point in which the effect-size coefficient was significantly greater than zero, and the corresponding areas under the curve were also calculated as indexes of diagnostic performance.
Finally, by maximizing the sum of the sensitivity and the specificity of the receiver operating characteristic curves, diagnostic cutoff values for both palatal parameters (ie, those associated with the highest sum of the corresponding sensitivity and specificity) were obtained, and a comprehensive diagnostic performance analysis was performed, including sensitivity, specificity, positive predictive value, and accuracy.
P <0.05 was used for rejection of the null hypothesis.
Results
The intraclass correlation coefficients for both palatal parameters were above 0.95. Method errors as means (95% confidence interval) were 1.0% (0.5-1.6) and 3.2% (1.5-5.4) for palatal surface areas and volumes, respectively.
The mean age and sex distribution at baseline were similar between the treatment and control groups ( P >0.1 at least; data not shown). Relevant dropouts were seen mostly at the last time point, when the numbers of subjects decreased to 10 and 23 for the treatment and control groups, respectively. However, a posteriori analysis showed a statistical power of 0.72 at 54 months. The mean ages of the groups at each time point remained similar, even considering those who dropped out (data not shown). Moreover, the rho correlation coefficients of the nonnormalized palatal surface area and volume recorded at each time point with the corresponding baseline values were all above the required threshold of 0.65: 0.657 to 0.895 (both for palatal surface areas, at 12 and 30 months, respectively).
At baseline, the palatal surface areas (means ± standard deviations) were 744 ± 71.0 mm 2 and 783 ± 79.3 mm 2 in the treatment and control groups, respectively. The palatal volumes were 2615.0 ± 379.4 mm 3 and 2983 ± 472.6 mm 3 in the treatment and control groups, respectively. The difference between the groups was statistically significant for palatal volume ( P <0.05) but not for palatal surface area.
The data for the increases in palatal surface area and volume are shown in Table I . In each group, significant increments in these 2 palatal parameters were seen over time (all comparisons at P <0.05, at least). Although for the control group a clear linear behavior was seen, for the treatment group a slight change in the behavior of the slope of the curve occurred between 42 and 54 months. At the cross-sectional analyses between the groups at each time point, significantly greater increments in palatal surface areas and volumes were seen for the treatment group up to 30 months ( P <0.05, at least). In contrast, no significant differences were seen between the groups at 42 and 54 months for either palatal parameter.
Time out of treatment (mo) | Palatal surface area increment (%) | Diff between groups ( P ) | Palatal volume | Increment (%) | Diff between groups ( P ) | |
---|---|---|---|---|---|---|
TG | CG | TG | CG | |||
6 | 5.7 (3.8-12.0) | 1.2 (−1.8-6.5) | 0.001 | 9.2 (3.7-16.9) | 1.4 (−3.0-5.8) | <0.001 |
12 | 9.4 (5.6-12.8) | 2.7 (−0.3-8.7) | <0.01 | 10.1 (4.8-18.3) | 5.3 (2.8-9.1) | <0.05 |
18 | 16.4 (11.3-25.3) | 8.1 (4.0-11.1) | 0.001 | 23.7 (14.3-33.8) | 3.0 (−1.8-11.3) | <0.001 |
30 | 18.9 (11.7-22.7) | 11.8 (8.5-14.7) | <0.05 | 19.9 (13.5-31.5) | 8.8 (4.8-16.1) | 0.001 |
42 | 16.1 (12.2-23.1) | 13.2 (10.1-19.9) | NS | 22.8 (15.7-25.4) | 15.3 (6.7-19.8) | NS |
54 | 15.0 (3.0-22.5) | 13.0 (7.8-16.7) | NS | 20.1 (1.2-39.5) | 13.1 (4.7-21.1) | NS |
Diff over time ( P ) | <0.05 | <0.001 | <0.001 | <0.001 |
The data for the effect-size coefficients for both palatal parameters are shown in Table II . The effect-size coefficients ranged from 0.13 for palatal surface area (at 54 months) to 1.59 for palatal volume (at 18 months). With the exception of 12 months, the effect-size coefficients for palatal volume were greater than those for palatal surface area. Effect-size coefficients greater than 1.0 were seen for both palatal parameters only at 18 months. At 42 and 54 months, for both palatal parameters, the effect-size coefficients were not significantly greater than zero. Therefore, the receiver operating characteristic curves were not computed for these last 2 time points.
Time out of treatment (mo) | Palatal surface area increment | Palatal volume increment |
---|---|---|
6 | 0.91 (0.31-1.50) ∗ | 0.95 (0.35-1.54) ∗ |
12 | 0.90 (0.25-1.54) ∗ | 0.64 (0.01-1.27) ∗ |
18 | 1.15 (0.52-1.79) ∗ | 1.59 (0.92-2.26) ∗ |
30 | 0.71 (0.06-1.36) ∗ | 0.99 (0.33-1.66) ∗ |
42 | 0.23 (−0.38-0.85) | 0.47 (−0.15-1.09) |
54 | 0.13 (−0.61-0.87) | 0.60 (−0.16-1.35) |
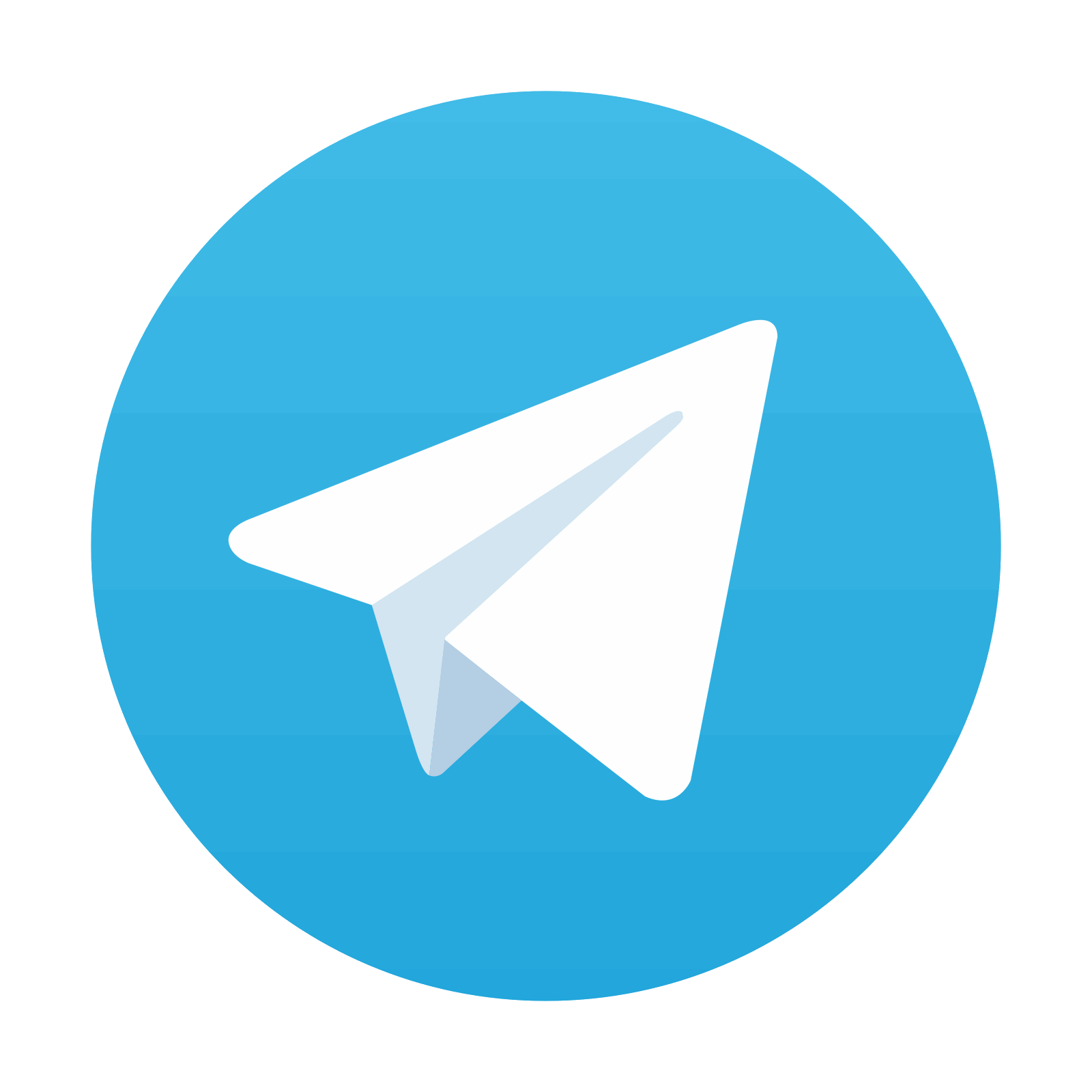
Stay updated, free dental videos. Join our Telegram channel

VIDEdental - Online dental courses
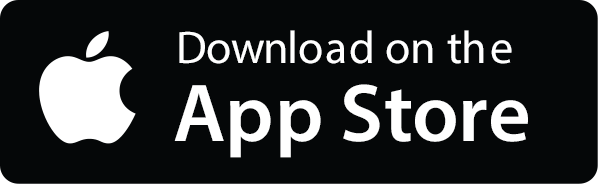
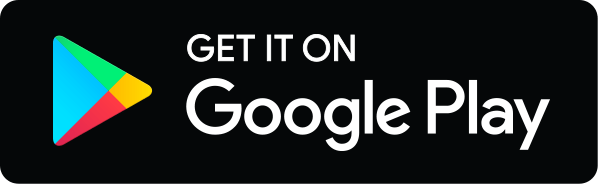
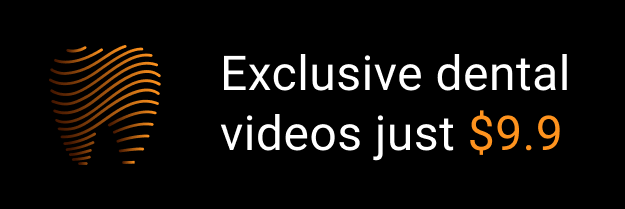