Abstract
Objective
The aim of this study was to identify the factors derived from the 405 nm laser-induced autofluorescence (AF) spectra that could be used to diagnose and stage caries.
Materials and methods
Teeth (20 teeth per stage) were classified as sound, stage II, III, and IV based on a visual and tactile inspection. The specimens were re-examined and reclassified based on micro-CT analysis. From the teeth, the AF was obtained using a 405 nm laser. Three spectral factors (spectral slope at 550–600 nm, area under the curve at 500–590 nm, and two-peak ratio between 625 and 667 nm) were derived from the AF spectra. Using these factors, the diagnosis and staging of caries were tested, and the results were compared with those of DIAGNOdent.
Results
After micro-CT analysis, only 13, 11, and 13 teeth were reclassified as stages II, III, and IV, respectively. The reclassified groups showed less data overlap between the stages, and the spectral slope was 40.1–74.6, 27.5–39.6, 11.1–27.4, and 1.0–9.7 for sound, stage II, III, and IV, respectively. The differentiation of stages III and IV using DIAGNOdent appeared to be difficult due to the considerable data overlap.
Conclusion
Among the factors tested, the spectral slope at 550–600 nm showed the best match with the caries specimens, in which their stage had been identified precisely.
Clinical significance
The 405 nm laser-induced AF spectra can be applied to the diagnosis and staging of caries alone or in conjunction with conventional methods, such as visual, tactile, and X-ray inspection.
1
Introduction
Caries is one of the most common chronic oral diseases that causes mental and physical distress. In many cases, caries is often neglected until it causes pain. On the other hand, if not properly diagnosed and treated in a timely manner, the affected tooth may need to be extracted due to the extensive damage to the dentin and pulp. Caries is a state of tooth demineralisation caused by the acids produced by oral bacteria, such as Streptococcus mutans and Lactobacillus , which reside in dental plaque biofilms. These bacteria consume sugars and carbohydrates as their food and produce several acids, such as acetic, lactic, and propionic acid, which can attack the enamel surface and dissolve carbonated hydroxyapatite, leaving a porous structure. During the early stages, the recovery of demineralised enamel can be achieved through the uptake of lost minerals, including calcium and phosphorus, which are dissolved in saliva or present in food. This remineralization process can be compromised when the pH at the tooth surface falls below 4.3, and carious lesions can form under such circumstances.
Initially, dental plaque is a colourless sticky thin layer that can form everywhere on the tooth surface; it then becomes brown or pale yellow when it transforms to calculus. If not controlled or removed properly from its initial stage by careful and periodic tooth brushing, it can progress to caries and various periodontal diseases. To minimise tooth damage and avoid subsequent treatment processes, the monitoring, diagnosis and the accurate staging of caries, if formed, are important for saving the teeth and reducing medical expenses. Conventionally, this is performed by a visual and tactile inspection with the aid of X-ray radiography. According to a wide systemic review and meta-analysis, a visual inspection was concluded to have good accuracy for the detection of caries at various stages on different tooth surfaces, but high study heterogeneity and the risk of bias were unavoidable. In addition to these conventional methods, several adjunctive methods have been introduced, such as, fibre optic transillumination (FOTI), quantitative light-induced fluorescence (QLF), digital image fibre optic transillumination (DIFOTI), and laser fluorescence (DIAGNOdent) using visual or laser light for excitation. Electrical conductance measurements (ECMs) and electrical impedance measurements (EIMs) employ an electrical current. These methods have their unique benefits and limitations in terms of achieving the reliable and accurate detection of caries. Thus far, DIAGNOdent has been adopted widely in clinics. This device uses a laser that emits 655 nm light, detects the fluorescence produced by oral bacteria and bacterial by-products, and produces digital results ranging from 0 to 99, which reflect the severity of caries. Therefore, DIAGNOdent is a cost-effective and nondestructive device that can detect caries from smooth and occlusal surfaces. Despite the claimed sensitivities and specificities of 70–80% regarding early enamel and occlusal dentin carious lesions, the results of in vivo studies conducted on this topic have been contradictory.
Recently, a 405 nm laser was tested for its applicability to the detection of caries. The basic principle of disease detection is the production of a characteristic autofluorescence (AF) spectrum by the disease-specific chromophores. In caries, the 405 nm laser produces a spectrum with peaks near 500 nm and higher than 600 nm, which are related to the organic substances embedded in the inorganic enamel and the by-products of oral bacterial activity, respectively. On the other hand, compared to DIAGNOdent, which responds only to oral bacteria and their endogenous by-products, the 405 nm laser interacts with both inorganic substances and the intrinsic and endogenous organic substances, and might be a more useful diagnostic tool. Thus far, however, there have been limited studies assessing the feasibility of the 405 nm laser for the diagnosis of caries. The purpose of the present study was to evaluate the feasibility of the AF spectra-related factors for the diagnosis and staging of carious lesion on teeth. The factors extracted from the AF spectrum, spectral slope, spectral area, and two-peak ratio, were assessed on the teeth whose state and stage of caries had been identified precisely by micro-CT. The most useful factor of the AF spectrum was determined by a comparison with the DIAGNOdent readings.
2
Materials and methods
2.1
Tooth preparation and classification
The present study was conducted using 325 teeth (molars and premolars) that were cleaned, frozen, and stored at a relative humidity of 100% after extraction. The Institutional Review Board at Pusan National University Dental Hospital, Yangsan, Korea, approved the study and waived informed consent. Two examiners inspected the stored teeth independently through a visual inspection (by eye and under an optical microscope) and by a tactile inspection using an explorer. The examined teeth were categorised as sound, stage II, III, and IV ( Table 1 ), and all teeth were classified identically by the two examiners. Twenty teeth were then selected randomly from each of these classes; these 80 teeth constituted the study groups. The teeth were then re-examined by micro-CT (inspeXio SMX-90CT, Shimadzu, Tokyo, Japan) at 90 kV and 100 μA. To determine the caries stage, the teeth were scanned longitudinally and transversely. As there is no way to determine the precise caries stage without cutting the specimens, micro-CT was used to determine the caries stage because it can visualise non-invasively the various lesions from the enamel surface to the dentin subsurface.
Code | Description | Relevant ICDAS II |
---|---|---|
Sound | Sound and glossy surface | Sound tooth surface (0) |
Stage II | Demineralization less 1/3 enamel, visible white spot, no glossy surface, remineralization can occur, dentist warns finding of incipient caries | First/Distinct visual change in enamel (1,2) |
Stage III | Demineralization over 1/3 enamel and possibly damaged to dentin, more clearly visible white spot than stage II, sometimes light to dark brown spot is visible, no cavitation yet, immediate treatment depend on internal demineralization | Enamel breakdown, no dentin visible (3) |
Stage IV | Cavitation is visible and progressed into dentin, white and/or brown discolored lesion is frequently visible, immediate treatment is required | Underlying dentinal shadow (not cavitated into dentin) − in our case, this case was grouped to III if there is no loss of surface integrity (4) |
Distinct cavity with visible dentin (5) | ||
Extensive distinct cavity with visible dentin (6) |
2.2
Autofluorescence analysis
The laser-induced autofluorescence (AF) spectra of the teeth were obtained using a 405 nm laser (LVI Technology, Seoul, Korea). The laser was operated at an output power of 2 ± 0.1 mW, as measured using a power meter (PM3/FieldMax, Coherent, Portland, OR, USA). To achieve stable and minimally fluctuating output power, the original power (100 mW) was attenuated to 2% using various combinations of filters (Thorlabs Inc., Newton, NJ, USA). For the easy and consistent positioning of the tooth, each tooth was placed on the XYZ-stage of the unit. The laser was focused normally on the center of each lesion for one second using a convex lens (f = 10 cm). The spot size on the lesion was less than 10 μm. The emission spectra were recorded using a spectrometer (QE65000FL, Ocean Optics Inc., Dunedin, FL, USA) by guiding the emitted light through an optical fibre (QP600-1-UV-VIS; 600 μm diameter silica core). The optical fibre was aligned at a 35−45° angle to the irradiated laser beam. The detection range of the spectrometer was 200–1100 nm and its optical resolution (grating #: 300; slit size: 200 μm) was 6.5 nm according to its slit and grating options. The end of the optical fibre (detector) was positioned 1 cm away from the lesion surface. A 450 nm longpass filter (Thorlabs Inc., Newton, NJ, USA) was placed in front of the optical fibre to attenuate the excitation light.
From the AF spectra obtained, three factors for determining the caries stage were extracted and assessed: the spectral slope, area under the curve (spectral area), and two-peak ratio. To determine the spectral slope, the AF spectra were analysed at 550–600 nm. This range was determined by checking the linearity (correlation coefficient, R) using a linear fit model. In this range, most specimens (78/80) had an R value of >0.97. The area under the curve at 500–590 nm was determined by integrating the curve using ORIGIN (Microcal Software Inc., Southampton, MA, USA). A range of 500 to 590 nm was used because some of the specimens showed an increasing emission profile after 590 nm. The 625/667 nm ratios (i.e., the two-peak ratios, the ratios of the peak emissions at 625 and 667 nm) were calculated to quantify the spectral changes after 600 nm. These wavelengths were determined because some specimens in stages II–IV showed one or two increasing peaks near 625 and 667 nm as caries progressed.
2.3
Examination using DIAGNOdent
The caries in each specimen was assessed using a DIAGNOdent pen (KaVo Dental, Biberach, Germany) according to the manufacturer’s instructions. Before the measurement, the device was calibrated against a ceramic standard. The measurements were taken three times on each tooth on the same area that was used to produce the AF spectra. As described by the manufacturer, the peak digital values were classified as follows: 0–13, healthy; 14–20, beginning of demineralisation; 21–29, strong demineralisation; and >30, dentin caries. Values >30 indicate the consideration of an X-ray test and possible minimally invasive treatment or resin filling. The manufacturer’s guidelines do not match the description in Table 1 precisely, but healthy (0–13), beginning demineralisation (14–20), strong demineralisation (21–29), and dentin caries (>30) correspond approximately to sound, stage II, stage III, and stage III/IV, respectively, of the present classification.
2.4
Fourier-transform infrared spectroscopy (FTIR) analysis
To analyse the compositional changes of the carious lesions, a FTIR spectrophotometer (Nicolet 6700/8700, Thermo Fisher Scientific Inc., Waltham, MA, USA) connected to an attenuated total reflection (ATR) accessory was used to obtain the spectra in the range, 7800–350 cm −1 . Thirty-two scans were performed per specimen at a resolution of 0.09 cm −1 . For each specimen, 2 mg of ground powder that was obtained from a carious lesion using a fissure bur was mixed with 10 mg of potassium bromide (KBr) and made into a thin film for the measurements.
2.5
Statistical analysis
The results (spectral slopes, areas under curves, two-peak ratios, and DIAGNOdent readings) were analysed by one-way ANOVA followed by a Tukey’s post-hoc test for multiple comparisons; p values <0.05 were considered significant.
2
Materials and methods
2.1
Tooth preparation and classification
The present study was conducted using 325 teeth (molars and premolars) that were cleaned, frozen, and stored at a relative humidity of 100% after extraction. The Institutional Review Board at Pusan National University Dental Hospital, Yangsan, Korea, approved the study and waived informed consent. Two examiners inspected the stored teeth independently through a visual inspection (by eye and under an optical microscope) and by a tactile inspection using an explorer. The examined teeth were categorised as sound, stage II, III, and IV ( Table 1 ), and all teeth were classified identically by the two examiners. Twenty teeth were then selected randomly from each of these classes; these 80 teeth constituted the study groups. The teeth were then re-examined by micro-CT (inspeXio SMX-90CT, Shimadzu, Tokyo, Japan) at 90 kV and 100 μA. To determine the caries stage, the teeth were scanned longitudinally and transversely. As there is no way to determine the precise caries stage without cutting the specimens, micro-CT was used to determine the caries stage because it can visualise non-invasively the various lesions from the enamel surface to the dentin subsurface.
Code | Description | Relevant ICDAS II |
---|---|---|
Sound | Sound and glossy surface | Sound tooth surface (0) |
Stage II | Demineralization less 1/3 enamel, visible white spot, no glossy surface, remineralization can occur, dentist warns finding of incipient caries | First/Distinct visual change in enamel (1,2) |
Stage III | Demineralization over 1/3 enamel and possibly damaged to dentin, more clearly visible white spot than stage II, sometimes light to dark brown spot is visible, no cavitation yet, immediate treatment depend on internal demineralization | Enamel breakdown, no dentin visible (3) |
Stage IV | Cavitation is visible and progressed into dentin, white and/or brown discolored lesion is frequently visible, immediate treatment is required | Underlying dentinal shadow (not cavitated into dentin) − in our case, this case was grouped to III if there is no loss of surface integrity (4) |
Distinct cavity with visible dentin (5) | ||
Extensive distinct cavity with visible dentin (6) |
2.2
Autofluorescence analysis
The laser-induced autofluorescence (AF) spectra of the teeth were obtained using a 405 nm laser (LVI Technology, Seoul, Korea). The laser was operated at an output power of 2 ± 0.1 mW, as measured using a power meter (PM3/FieldMax, Coherent, Portland, OR, USA). To achieve stable and minimally fluctuating output power, the original power (100 mW) was attenuated to 2% using various combinations of filters (Thorlabs Inc., Newton, NJ, USA). For the easy and consistent positioning of the tooth, each tooth was placed on the XYZ-stage of the unit. The laser was focused normally on the center of each lesion for one second using a convex lens (f = 10 cm). The spot size on the lesion was less than 10 μm. The emission spectra were recorded using a spectrometer (QE65000FL, Ocean Optics Inc., Dunedin, FL, USA) by guiding the emitted light through an optical fibre (QP600-1-UV-VIS; 600 μm diameter silica core). The optical fibre was aligned at a 35−45° angle to the irradiated laser beam. The detection range of the spectrometer was 200–1100 nm and its optical resolution (grating #: 300; slit size: 200 μm) was 6.5 nm according to its slit and grating options. The end of the optical fibre (detector) was positioned 1 cm away from the lesion surface. A 450 nm longpass filter (Thorlabs Inc., Newton, NJ, USA) was placed in front of the optical fibre to attenuate the excitation light.
From the AF spectra obtained, three factors for determining the caries stage were extracted and assessed: the spectral slope, area under the curve (spectral area), and two-peak ratio. To determine the spectral slope, the AF spectra were analysed at 550–600 nm. This range was determined by checking the linearity (correlation coefficient, R) using a linear fit model. In this range, most specimens (78/80) had an R value of >0.97. The area under the curve at 500–590 nm was determined by integrating the curve using ORIGIN (Microcal Software Inc., Southampton, MA, USA). A range of 500 to 590 nm was used because some of the specimens showed an increasing emission profile after 590 nm. The 625/667 nm ratios (i.e., the two-peak ratios, the ratios of the peak emissions at 625 and 667 nm) were calculated to quantify the spectral changes after 600 nm. These wavelengths were determined because some specimens in stages II–IV showed one or two increasing peaks near 625 and 667 nm as caries progressed.
2.3
Examination using DIAGNOdent
The caries in each specimen was assessed using a DIAGNOdent pen (KaVo Dental, Biberach, Germany) according to the manufacturer’s instructions. Before the measurement, the device was calibrated against a ceramic standard. The measurements were taken three times on each tooth on the same area that was used to produce the AF spectra. As described by the manufacturer, the peak digital values were classified as follows: 0–13, healthy; 14–20, beginning of demineralisation; 21–29, strong demineralisation; and >30, dentin caries. Values >30 indicate the consideration of an X-ray test and possible minimally invasive treatment or resin filling. The manufacturer’s guidelines do not match the description in Table 1 precisely, but healthy (0–13), beginning demineralisation (14–20), strong demineralisation (21–29), and dentin caries (>30) correspond approximately to sound, stage II, stage III, and stage III/IV, respectively, of the present classification.
2.4
Fourier-transform infrared spectroscopy (FTIR) analysis
To analyse the compositional changes of the carious lesions, a FTIR spectrophotometer (Nicolet 6700/8700, Thermo Fisher Scientific Inc., Waltham, MA, USA) connected to an attenuated total reflection (ATR) accessory was used to obtain the spectra in the range, 7800–350 cm −1 . Thirty-two scans were performed per specimen at a resolution of 0.09 cm −1 . For each specimen, 2 mg of ground powder that was obtained from a carious lesion using a fissure bur was mixed with 10 mg of potassium bromide (KBr) and made into a thin film for the measurements.
2.5
Statistical analysis
The results (spectral slopes, areas under curves, two-peak ratios, and DIAGNOdent readings) were analysed by one-way ANOVA followed by a Tukey’s post-hoc test for multiple comparisons; p values <0.05 were considered significant.
3
Results
Table 2 shows both the number of specimens allocated to sound, stages II, III, and IV after the visual and tactile inspections and after micro-CT analysis, which was performed using the same specimens used for the visual and tactile inspections. Stages II, III, and IV were classified differently; only 13, 11, and 13 out of 20 specimens were re-classified as stages II, III, and IV, respectively, after micro-CT analysis.
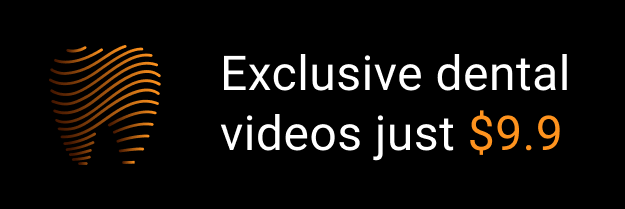