Abstract
Aim
An innovative light-curable calcium-silicate cement containing a HEMA–TEGDMA-based resin (lc-MTA) was designed to obtain a bioactive fast setting root-end filling and root repair material.
Methods
lc-MTA was tested for setting time, solubility, water absorption, calcium release, alkalinizing activity (pH of soaking water), bioactivity (apatite-forming ability) and cell growth-proliferation. The apatite-forming ability was investigated by micro-Raman, ATR-FTIR and ESEM/EDX after immersion at 37 °C for 1–28 days in DPBS or DMEM + FBS. The marginal adaptation of cement in root-end cavities of extracted teeth was assessed by ESEM/EDX, and the viability of Saos-2 cell on cements was evaluated.
Results
lc-MTA demonstrated a rapid setting time (2 min), low solubility, high calcium release (150–200 ppm) and alkalinizing power (pH 10–12). lc-MTA proved the formation of bone-like apatite spherulites just after 1 day. Apatite precipitates completely filled the interface porosities and created a perfect marginal adaptation. lc-MTA allowed Saos-2 cell viability and growth and no compromising toxicity was exerted.
Significance
HEMA–TEGDMA creates a polymeric network able to stabilize the outer surface of the cement and a hydrophilic matrix permeable enough to allow water absorption. SiO − /Si–OH groups from the mineral particles induce heterogeneous nucleation of apatite by sorption of calcium and phosphate ions. Oxygen-containing groups from poly-HEMA–TEGDMA provide additional apatite nucleating sites through the formation of calcium chelates. The strong novelty was that the combination of a hydraulic calcium-silicate powder and a poly-HEMA–TEGDMA hydrophilic resin creates the conditions (calcium release and functional groups able to chelate Ca ions) for a bioactive fast setting light-curable material for clinical applications in dental and maxillofacial surgery. The first and unique/exclusive light-curable calcium-silicate MTA cement for endodontics and root-end application was created, with a potential strong impact on surgical procedures.
1
Introduction
Bacteria penetration into root canal is responsible for pulpitis and periapical tissue inflammation which may evolve in apical granuloma, cystic lesions and large bone defect . These lesions require the removal of infected dentin root-apex and large portions of periapical bone tissue and the filling of the root-apex cavity with a root-end sealing material preferably biocompatible, osteoconductive or osteoinductive. The failure rate of surgical root-end therapy with conventional materials, such as zinc-oxide cements and silver-amalgam, was reported approximately as 8–24% . The consequences are bone resorption and tooth extraction. Millions of persons are surgically treated for endodontic diseases. The success of the endodontic treatment allows to keep the tooth functionality.
Calcium-silicate hydraulic cements conventionally defined MTA (mineral trioxide aggregate) cements have been clinically proposed as root-end materials , and prospective studies have reported a failure of 9.8–16% at 1 year and 8% at 2 years .
The biological behavior and the apatite-forming ability (i.e. bioactivity) of MTA cements have been recently adequately documented .
The main clinical limitation of MTA cements is the long setting time and the consequent risk for a fast dissolution and removal of the cement and wash-out of the fresh (not-set) cement from the surgical site of root-end obturation due to the blood and fluid contamination at the apical region of root canal. The incorporation of light-curable resins has been proposed for many materials, such as the resin-modified glass-ionomer cements, to improve mechanical properties and reduce setting time. The reduced setting time of MTA materials may extend their clinical use and make their application advisable in extremely wet and blood-contaminated surgical sites.
HEMA (2-hydroxyethyl methacrylate) is a hydrophilic monovinyl monomer (i.e. it contains a single C C double bond, Fig. 1 ). Its polymer poly-HEMA is a hydrogel widely used together with other methacrylic resins in biomedical applications. Poly-HEMA can imbibe large amounts of water (from 10 to 600%) by swelling without dissolving, due to the hydrophilic pendant groups of the molecule ( Fig. 1 ); it has been extensively tested for bioactivity and biocompatibility .

TEGDMA (triethyleneglycol dimethacrylate, Fig. 1 ) is a hydrophobic monomer that contains two C C double bonds; its introduction into the resin formulation allows the formation of covalent crosslinks after curing and thus tight networks and solid structures .
Bioactivity of calcium-silicate Portland cements and other MTA materials such as ProRoot MTA has been recently demonstrated . An essential requirement for a bioactive material is the formation of a biologically active bone-like apatite layer on its surface in a biological environment . The concept of bioactivity is closely correlated with biointeractivity, i.e. the ability to exchange information within a biological system . This means that a bioactive material reacts chemically with body fluids in a manner compatible with the repair processes of the tissue.
The examination of apatite formation on a material in a simulated body fluid (SBF) is a commonly accepted method to predict the in vivo bone bioactivity of a specific material .
The aim of the study was to characterize an innovative light-curable resin-modified calcium-silicate cement (lc-MTA), containing a HEMA–TEGDMA-based anphiphilic resin as organic light-curable matrix and a calcium-silicate powder, specifically designed for applications in contact with bone and dentin for oral surgery and dentistry. The goal was to obtain a material with more adequate characteristics and properties for applications in wet apical cavities contaminated by blood during the preparation of a bone window, as it occurs during root-end surgery procedures and root repair procedures. Chemical–physical properties, i.e. setting time, solubility, water absorption, calcium release, alkalinizing activity were evaluated. The in vitro apatite-forming ability was assessed by ESEM/EDX, micro-Raman and ATR-FTIR techniques after soaking in phosphate-containing solutions. Osteoblast-like cells (Saos-2) were used to estimate the biological compatibility of solid cements and cement extracts.
2
Materials and methods
2.1
Sample preparation
The light-curable resin-modified calcium-silicate cement (lc-MTA) was prepared by mixing a calcium-silicate cement powder (wTC-Ba) and an anphiphilic light-curable resin liquid phase (Gandolfi MG & Prati C, patent of the University of Bologna).
The wTC-Ba powder was constituted by di- and tricalcium-silicates, tricalcium aluminate, barium sulfate, calcium sulfate and calcium chloride; it was prepared by a conventional melt-quenching technique . The liquid phase contained 2-hydroxyethyl methacrylate (HEMA), triethyleneglycol dimethacrylate (TEGDMA), camphorquinone (CQ) and ethyl-4-(dimethylamino)benzoate (EDMAB).
To obtain the wTC-Ba cement, the wTC-Ba powder was mixed with Dulbecco’s phosphate buffer solution (DPBS, Lonza, Lonza Walkersville Inc., Walkersville, MD, USA, cat. no. BE17-512) for 30 s in a powder/liquid ratio of 3:1.
To obtain the lc-MTA cement, the wTC-Ba powder was mixed with the liquid phase for 30 s in a powder/liquid ratio of 4:1 and light-cured for 120 s with a halogen lamp (T-LED elca, Anthos Cefla, Imola, Italy).
A commercial hydraulic calcium-aluminosilicate Portland cement named ProRoot MTA (white ProRoot MTA, Dentsply Maillefer, Tulsa, Ok, USA—lot no. 08003395) was used as reference (a reference material is required by ISO 7405 clause 3) since biocompatible osteoconductive material . ProRoot MTA powder was mixed with the supplied deionized water for 30 s in a powder/liquid ratio of 3:1.
Vitrebond (3M ESPE AG, Dental Products, St.Paul, MN, USA—lot. no. 70-2010-2611-2) is a resin-modified glass-ionomer cement and was used as light-curable control (a positive control is required by both ISO 7405 and ISO 10993-5 clause 3) since is a material able to evoke a positive or reactive response (i.e. cytotoxic material) . The cement was prepared according to the manufacturer directions and light-cured for 30 s with a halogen lamp. As stated by the manufacturer, Vitrebond is composed by a fluoro-aluminosilicate powder (constituted by SiO 2 , AlF 3 , ZnO, SrO, Na 3 AlF 6 (criolite), NH 4 F, MgO, and P 2 O 5 ) mixed with a light-curable liquid (containing polyacrylic acid with pendant methacrylate groups (PAA), 2-hydroxyethylmethacrylate (HEMA), water and photoinitiator camphorquinone) .
Standard cell culture plastic surface (Tissue Culture Polystyrene, TCPS) was used as negative control since a material that proved a non-reactive response in the test system as required by both ISO 7405 and ISO 10993-5 clause 3 .
Once mixed, all cements were compacted to the excess into PVC molds (8 mm in diameter and 1.6 mm thick). The wTC-Ba and ProRoot MTA were cured at 37 °C and 98% relative humidity. For solubility, calcium release and alkalinizing activity tests, the samples were cured (at 37 °C and 98% relative humidity) for a period corresponding to 70% of final setting time, i.e. 40 min for wTC-Ba and 117 min for ProRoot MTA (period of time 50% longer than the setting time stated by the manufacturer, according with ISO6876 clause 7.7.2.) and then demolded.
The lc-MTA and Vitrebond specimens were light-cured through a mylard streep (Directa Matrix Strips, Directa AB, Upplands Vasby, Sweden) and immediately demolded.
The obtained cylindrical specimens (8 mm in diameter, 1.6 mm thick and 0.3 g of weight) exposed an exchange surface of 90.43 ± 0.01 mm 2 (upper surface πr 2 = 50.24 mm 2 and lateral surface 2 rπh = 40.19 mm 2 ).
2.2
Setting times
The initial and final setting times of the cements were evaluated using Gilmore needles according to ASTM C266-03 and ADA specifications . Briefly, the Gilmore initial setting time was the elapsed time (min) between the mixing of the cement with liquid and the first penetration measurement that does not mark the specimen surface with a complete circular impression. As the initial or final setting times approached (i.e. no indentation), the specimens were tested every minute to determine the exact Gilmore setting time. The initial setting time needle was 113.4 g in weight and 2.12 mm in tip diameter. After the initial setting time was measured, the specimens were tested every 5 min with the final setting time needle with a tip diameter of 1.06 mm and a weight of 453.6 g.
The wTC-Ba and ProRoot MTA samples were removed from the curing chamber (37 °C and 98% relative humidity) and immediately tested for setting time to prevent the dehydration of cement surface. The lc-MTA and Vitrebond samples were light-cured (for 120 or 60 s, respectively) and immediately tested for setting time to standardize the polymerization level and to prevent any polymerization progress. Each sample was used only for one penetration/indentation test and then discarded. A large number of samples were used to find the approximate initial and final setting times of the cements. Their exact evaluation was performed on three replicates for each material.
2.3
Solubility
The solubility of the materials was determined using the method described in ISO 6876 . The mass of the cements was measured gravimetrically using an analytical balance (Bel Engineering series M, Monza, Italy) with an accuracy of 0.001 g after 1, 14 and 28 days of soaking in deionized water or in DMEM + FBS, i.e. Dulbecco’s modified eagle medium (DMEM, Lonza, Lonza Walkersville Inc., Walkersville, MD, USA, cat. no. 12-604) added with 10% fetal bovine serum (FBS, Lonza, Lonza Walkersville Inc., Walkersville, MD, USA, cat. no. DE14-801E). Each weight measurement was repeated three times.
The specimens ( n = 5 for each material) were weighed (Initial weight) and placed in sealed cylindrical polystyrene holders (3 cm high and 4 cm in diameter) containing 5 mL of deionized water or DMEM + FBS, at 37 °C. At the pre-determined intervals, the samples were removed from the solutions, blotted dry at 37 °C for 48 h, i.e. till the weight was stable and then weighed and finally discarded.
The solubility (percentage weight variation, Δ W %) at each time t was calculated according to the following equation:
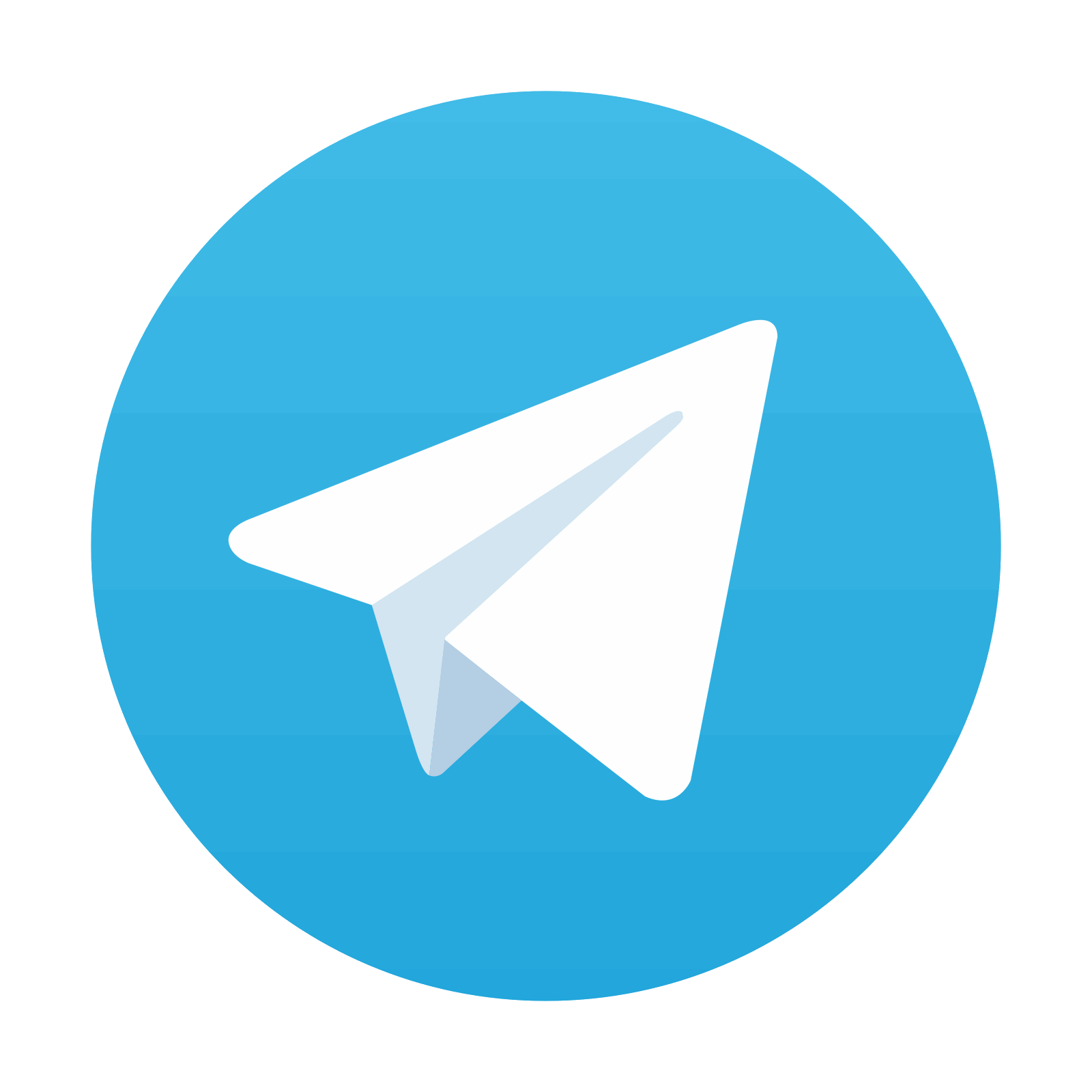
Stay updated, free dental videos. Join our Telegram channel

VIDEdental - Online dental courses
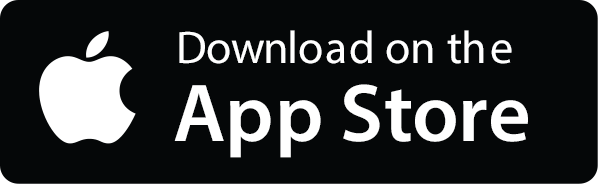
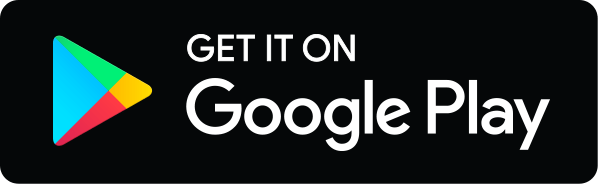