Abstract
Objective
The bonding potential of ‘mild’ self-etch adhesives may be compromised due to smear interference, as they may not dissolve/penetrate the smear layer effectively due to their relatively low acidity. We observed that the thickness of the dentin smear layer differed depending on the surface-preparation methodology used.
Methods
The interaction of an (ultra-)mild self-etch adhesive (Clearfil S3 Bond, Kuraray Noritake) with human dentin, prepared either using a medium-grit diamond bur (‘thick’, clinically relevant smear layer) or 600-grit SiC-paper (‘thin’ smear layer), or just fractured (smear-free), was evaluated using high-resolution transmission electron microscopy (TEM). Non-demineralized/demineralized 30–100 nm interfacial cross-sections were prepared following common TEM-specimen processing and diamond-knife ultra-microtomy.
Results
The adhesive did not dissolve the bur-cut, nor the SiC-ground smear layer, but impregnated it. Within this ‘resin-smear complex’, hydroxyapatite was abundantly present. At fractured dentin, this complex was not present, while the actual layer of interaction of the adhesive was limited to about 100 nm. Non-demineralized ‘ultra-thin’ (30–50 nm) sections confirmed the interfacial ultra-structure to differ for the three surface-preparation methods. An electron dense band was consistently disclosed at the adhesive interface, most likely representing the documented chemical interaction of the functional monomer 10-MDP with Ca.
Significance
The dentin surface-preparation method significantly affects the nature of the smear layer and the interaction with the ultra-mild self-etch adhesive.
1
Introduction
In many laboratory studies investigating dentin bonding effectiveness, SiC-ground surfaces are used, for reasons of standardization and ease of preparation . Nevertheless, a smear layer created during clinical procedures with a diamond or tungsten bur is rather thick and compact, and so may compromise the bonding effectiveness to dentin, in particular when a self-etch adhesive is used . A thick smear layer may also be porous and thus have weak mechanical properties . In this respect, the documented compromised dentin bonding effectiveness of contemporary one-step self-etch adhesives, which combine conditioning, priming and application of adhesive resin, could to a certain extent be attributed to interference of bur debris smeared across dentin during cavity preparation. However, there is very little detailed morphological data on the effect of smear interposition at the adhesive-dentin interface produced by so-called ‘ultra-mild’ self-etch adhesives, which are significantly less acidic (pH ≈ 2.7). Especially completeness of resin penetration and adequate resin envelopment of potential residual smear is highly important, as insufficient resin impregnation may result in more rapid interface degradation . Therefore, high-resolution ultra-morphological examination is thought to give a better insight what impact potential interfacial interference of bur smear may have on the bond durability .
The purpose of this study was to examine the effect of different surface preparation methods on the interfacial ultrastructure of an ultra-mild self-etch adhesive bonded to dentin. For this, high-resolution transmission electron microscopy (TEM) is the method of choice, as the combination of resolution and image detail is unsurpassed.
2
Materials and methods
Non-carious human third molars were stored in 0.5% chloramine solution at 4 °C and used within one month after extraction. The teeth were randomly divided into 3 groups: ‘BUR-CUT’, ‘SiC-GROUND’ and ‘FRACTURED’ dentin (see below). All teeth were mounted in gypsum blocks in order to ease manipulation. For ‘BUR-CUT’ dentin, the occlusal third of the crown was removed at the level of mid-coronal dentin using a slow-speed diamond saw (Isomet 1000, Buehler, Lake Bluff, IL, USA), after which a standard smear layer was produced by a medium-grit (100 μm) diamond bur (842, Komet, Lemgo, Germany) using a water-cooled high-speed contra-angle hand piece mounted in the MicroSpecimen Former (The University of Iowa, Iowa City, IA, USA). Half of the bur-cut specimens were further processed by wet-sanding with 600-grit silicone-carbide paper (‘SiC-GROUND’). For ‘FRACTURED’ (smear-free) dentin, a shallow 1–2 mm deep groove was cut circumferentially around the tooth at the level of mid-coronal dentin, after which the coronal part was fractured using a forceps to produce a fractured dentin surface free of smear debris. All dentin surfaces were carefully verified for absence of enamel and/or pulp tissue using a stereo-microscope (Wild M5A, Wild Heerbrugg AG, Heerbrugg, Switzerland).
The ultra-mild one-step self-etch adhesive Clearfil S3 Bond (Kuraray Noritake, Tokyo, Japan) was applied according to the manufacturer’s instructions, followed by a thin layer of flowable composite (Clearfil Protect Liner F, Kuraray Noritake). Light-curing was performed using an Optilux 500 (Demetron/Kerr, Danbury, CT, USA) device with a light output not less than 600 mW/cm 2 . After bonding procedures, specimens were stored for 1 day in distilled water at 37 °C. The specimens were processed according to the procedure described in detail by Van Meerbeek et al. . Non-demineralized and laboratory-demineralized thin (60–100 nm) and non-demineralized ultra-thin (30–50 nm) sections were cut (Ultracut UCT, Leica, Vienna, Austria) and examined unstained and positively stained (3% uranyl acetate for 12 min/lead citrate for 13 min) using TEM (JEM-1200EX II, JEOL, Tokyo, Japan). In order to reveal potential porous zones at the interface, additional specimens were immersed in a 50 wt% ammoniacal silver nitrate solution according to a nanoleakage-detection protocol previously described by Tay et al. .
2
Materials and methods
Non-carious human third molars were stored in 0.5% chloramine solution at 4 °C and used within one month after extraction. The teeth were randomly divided into 3 groups: ‘BUR-CUT’, ‘SiC-GROUND’ and ‘FRACTURED’ dentin (see below). All teeth were mounted in gypsum blocks in order to ease manipulation. For ‘BUR-CUT’ dentin, the occlusal third of the crown was removed at the level of mid-coronal dentin using a slow-speed diamond saw (Isomet 1000, Buehler, Lake Bluff, IL, USA), after which a standard smear layer was produced by a medium-grit (100 μm) diamond bur (842, Komet, Lemgo, Germany) using a water-cooled high-speed contra-angle hand piece mounted in the MicroSpecimen Former (The University of Iowa, Iowa City, IA, USA). Half of the bur-cut specimens were further processed by wet-sanding with 600-grit silicone-carbide paper (‘SiC-GROUND’). For ‘FRACTURED’ (smear-free) dentin, a shallow 1–2 mm deep groove was cut circumferentially around the tooth at the level of mid-coronal dentin, after which the coronal part was fractured using a forceps to produce a fractured dentin surface free of smear debris. All dentin surfaces were carefully verified for absence of enamel and/or pulp tissue using a stereo-microscope (Wild M5A, Wild Heerbrugg AG, Heerbrugg, Switzerland).
The ultra-mild one-step self-etch adhesive Clearfil S3 Bond (Kuraray Noritake, Tokyo, Japan) was applied according to the manufacturer’s instructions, followed by a thin layer of flowable composite (Clearfil Protect Liner F, Kuraray Noritake). Light-curing was performed using an Optilux 500 (Demetron/Kerr, Danbury, CT, USA) device with a light output not less than 600 mW/cm 2 . After bonding procedures, specimens were stored for 1 day in distilled water at 37 °C. The specimens were processed according to the procedure described in detail by Van Meerbeek et al. . Non-demineralized and laboratory-demineralized thin (60–100 nm) and non-demineralized ultra-thin (30–50 nm) sections were cut (Ultracut UCT, Leica, Vienna, Austria) and examined unstained and positively stained (3% uranyl acetate for 12 min/lead citrate for 13 min) using TEM (JEM-1200EX II, JEOL, Tokyo, Japan). In order to reveal potential porous zones at the interface, additional specimens were immersed in a 50 wt% ammoniacal silver nitrate solution according to a nanoleakage-detection protocol previously described by Tay et al. .
3
Results
TEM of non-demineralized sections revealed clear differences in substrate irregularity and smear-layer thickness for the different surface-preparation methods applied. At FRACTURED dentin, a smear layer could not be observed, but distinct resin tags were clearly formed ( Fig. 1 a and b ). At SiC-GROUND dentin, the interface appeared relatively flat and smooth with a regularly thin smear layer (up to 1 μm) deposited by the shearing/pushing motion during grinding ( Fig. 2 a and b ). Due to the smear plugs in the dentin tubules, resin tags were clearly less prominent than at fractured dentin. At BUR-CUT dentin, the surface was clearly more irregular with a much thicker (up to 5 μm), but also much more in thickness varying smear layer deposited ( Fig. 3 a and b ). The adhesive was not able to visibly dissolve the underlying dentin substrate at FRACTURED dentin ( Fig. 1 b), nor able to dissolve the SiC-GROUND ( Fig. 2 b) and the BUR-CUT smear layer ( Fig. 3 b). In the two latter conditions, a ‘resin-smear complex’ was formed, in which hydroxyapatite (HAp), often fragmented, was abundantly present. AgNO 3 -immersed specimens revealed relatively small deposits of silver within the adhesive and at the adhesive-dentin interface at FRACTURED dentin ( Fig. 1 c), most likely due to absence of surface smear. In contrast, a varying pattern of both spot- and cluster-like appearance of nano-leakage was revealed within the resin-smear complex and within dentin for the SiC-GROUND or BUR-CUT dentin specimens.
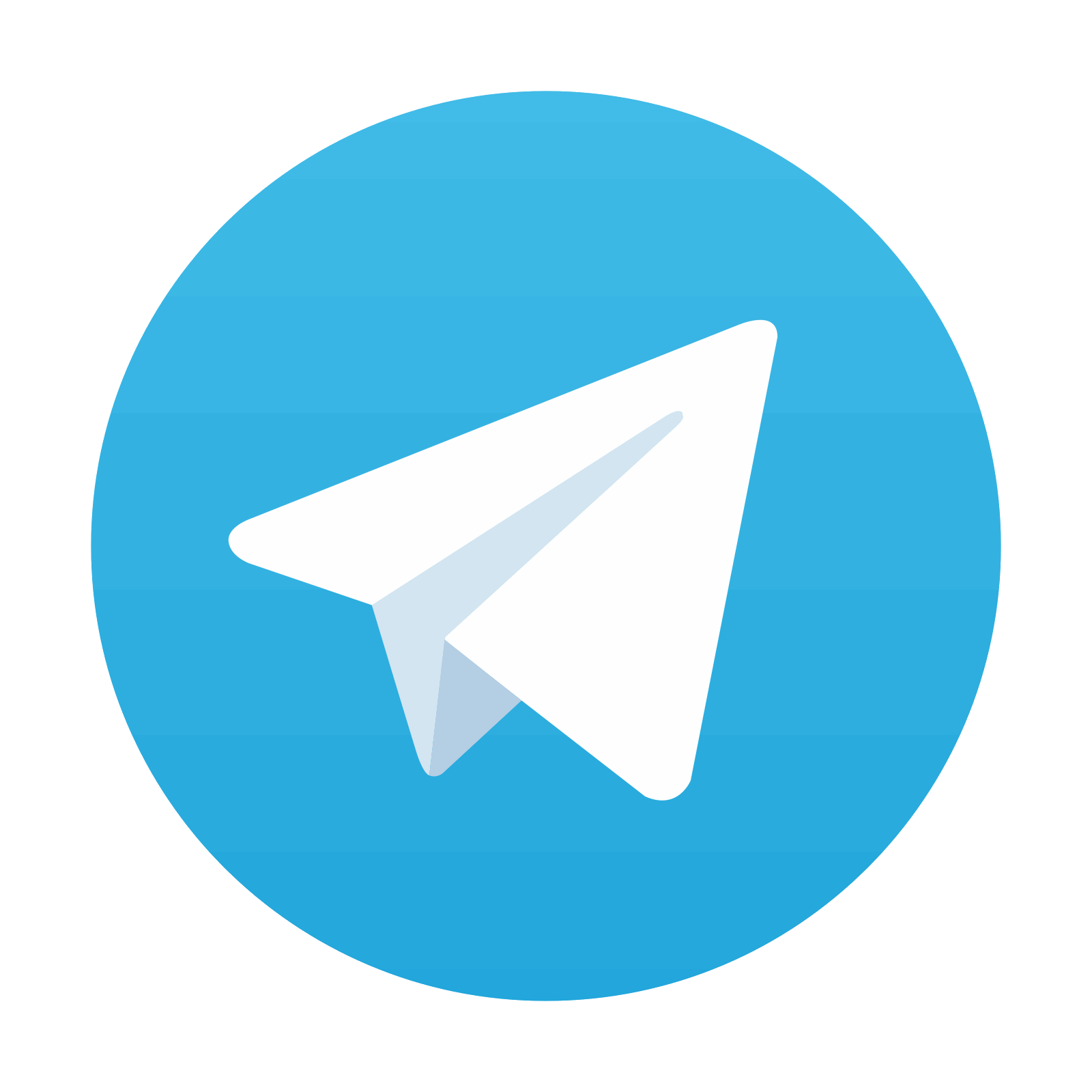
Stay updated, free dental videos. Join our Telegram channel

VIDEdental - Online dental courses
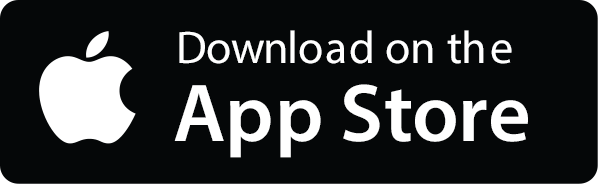
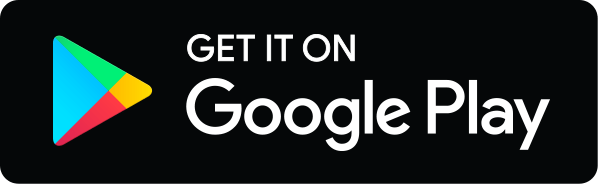
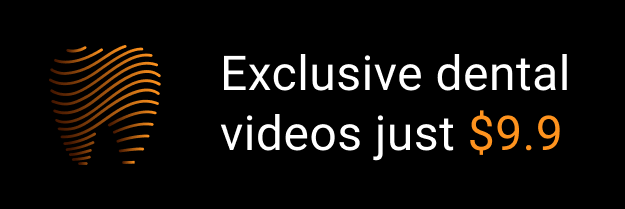