Abstract
Objectives
This study presents a variant of the Brazilian disk test (BDT) for assessing the bond strength between composite resins and dentin.
Methods
Dentin-composite disks (ϕ 5 mm × 2 mm) were prepared using either Z100 or Z250 (3M ESPE) in combination with one of three adhesives, Adper Easy Bond (EB), Adper Scotchbond Multi-Purpose (MP) and Adper Single Bond (SB), and tested under diametral compression. Acoustic emission (AE) and digital image correlation (DIC) were used to monitor debonding of the composite from the dentin ring. A finite element (FE) model was created to calculate the bond strengths using the failure loads. Fracture modes were examined by scanning electron microscopy (SEM).
Results
Most specimens fractured along the dentin-resin composite interface. DIC and AE confirmed interfacial debonding immediately before fracture of the dentin ring. Results showed that the mean bond strength with EB (14.9 ± 1.9 MPa) was significantly higher than with MP (13.2 ± 2.4 MPa) or SB (12.9 ± 3.0 MPa) ( p < 0.05 ); no significant difference was found between MP and SB ( p > 0.05 ). Z100 (14.5 ± 2.3 MPa) showed higher bond strength than Z250 (12.7 ± 2.5 MPa) ( p < 0.05 ). Majority of specimens (91.3%) showed an adhesive failure mode. EB failed mostly at the dentin-adhesive interface, whereas MP at the composite-adhesive interface; specimens with SB failed at the composite-adhesive interface and cohesively in the adhesive.
Conclusions
The BDT variant showed to be a suitable alternative for measuring the bond strength between dentin and composite, with zero premature failure, reduced variability in the measurements, and consistent failure at the dentin-composite interface.
Clinical significance
The new test could help to predict the clinical performance of adhesive systems more effectively and consistently by reducing the coefficient of variation in the measured bond strength.
1
Introduction
An often cited reason for the failure of composite restorations is the breakdown of the tooth-composite interface , whereas mechanical forces resulting from composite polymerization shrinkage or mastication are the main reasons for this breakdown . The marginal gaps thus formed around the restoration would allow bacterial invasion and biofilm accumulation, leading to secondary caries .
Bond strength testing is therefore routinely used to assess the interfacial strength between the tooth and restoration. Different bond strength tests have been developed over the years, with the shear (SBS) and tensile bond strength (TBS) tests being the most popular. However, there are certain drawbacks associated with these tests . For example, difficulties with machining, handling, aligning and fixing the matchstick specimens are some of the problems encountered in the TBS test . Also, when the bond strength is comparable or higher than the fracture strength of the substrates, a high percentage of cohesive fracture in the substrates may result with the TBS test . Similarly, in the SBS test, cohesive failure often occurs within the dental or composite substrate . Obviously, for a bond strength test to be valid, failure must initiate from the interface . In addition to the above problems, analyses have shown that the stress distribution at the tooth-restoration interface of some of the specimens is highly non-uniform and greatly depends on the material property mismatch, specimen geometry and attachment conditions . In the SBS test specimen, for example, the dominant stress state is that of tension rather than shear .
As an alternative bond strength test, we recently proposed a variant of the Brazilian disk test (BDT), or disk in diametral compression, to assess the interfacial debonding of endodontic posts from root dentin . The BDT has been used widely for testing the tensile strength and fracture toughness of brittle materials. It has also been used to test the interfacial fracture toughness of various dissimilar materials . During the test, tensile stresses are introduced in the horizontal direction, i.e., transverse to the applied vertical compressive load. The force required to cause failure is used to estimate the tensile strength . In the previous work , the disk specimen consisted of a slice of root dentin with a section of a circular post cemented in the enlarged concentric root canal. The dentin itself was surrounded by a layer of resin composite to form a disk of 10-mm diameter and 2-mm thick. Using the acoustic emission (AE) and digital image correlation (DIC) techniques, we confirmed that fracture of the disk under diametral compression was initiated by debonding at the post-dentin interface. Compared with some of the other bond tests for endodontic posts, the modified Brazilian disk test had the advantages of simpler specimen preparation and reduced variations in the results. In this paper, we introduce another modification of the BDT specimen that is more suitable for assessing the bond strength of direct composite restorations. Our goal is to determine whether the new test specimen would retain the advantages seen in its previous form for endodontic post testing. Again, DIC and AE were used to validate the test.
2
Methods
2.1
Sample preparation
The root portions from approximately 30 bovine incisors were removed and cut into two halves with a diamond saw under water cooling. The cut was made perpendicularly to the long root axis, with each half having an approximate length of 6–7 mm. From these, the halves that had a root canal larger than the intended diameter were rejected. Next, the canal of each selected root segment was enlarged with a 1.9-mm diameter fiber post drill using water as coolant (3M ESPE, Dental products, St. Paul, MN, USA) to obtain a circular hole of ∼2-mm diameter. Afterwards, the root segments were trimmed down using a lathe to remove the cementum and the external layer of dentin to produce hollow dentin cylinders of 5 ± 0.05-mm outer diameter and 2.05 ± 0.05-mm inner diameter ( Fig. 1 ).
The machined dentin cylinders were randomly assigned to three bonding systems: Total-etch Adper™ Single Bond Plus (SB), Self-etch Adper™ Easy Bond Adhesive (EB) and total-etch Adper™ Scotchbond™ Multi-Purpose Adhesive (MP); see Table 1 . Each bonding system was applied to the inner surface of the hollow dentin cylinders with a disposable brush applicator provided by the manufacturer and subsequently cured according to the manufacturer’s instructions. After this, the canal of each cylinder was restored with either Filtek™ Z250 Universal Restorative or Z100™ Restorative; see also Table 1 . The composites were applied incrementally to minimize shrinkage stress, with each layer being less than 2-mm thick. The first increment was placed in the middle section of the cylinder with the aid of a composite condenser and the subsequent increments placed at its ends. The first increment was cured from both ends for 20 s each. The end increments were cured from the respective ends of the cylinder for 40s. Light curing was done with an Elipar Trilight (3M ESPE, Dental Products, St. Paul, MN, USA) curing light operated at 800 mW/cm 2 .
Product | Composition | Batch number |
---|---|---|
Z100™ Restorative | Silane treated ceramic, triethylene glycol dimethacrylate (TEGDMA), bisphenol a diglycidyl ether dimethacrylate (BISGMA), 2-benzotriazolyl-4-methylphenol a . | N362970 |
Filtek™ Z250 Universal Restorative | Silane treated ceramic, bisphenol a polyethylene glycol diether dimethacrylate (BISEMA6), diurethane dimethacrylate (UDMA), bisphenol a diglycidyl ether dimethacrylate (BISGMA), triethylene glycol dimethacrylate (TEGMA), benzotriazol, ethyl 4-dimethyl aminobenzoate (EDMAB) a . | N326080 |
Adper Single Bond Plus | Ethyl alcohol, silane treated silica (nanofiller), bisphenol a diglycidyl ether dimethacrylate (BISGMA), 2-hydroxyethyl methacrylate(HEMA), glycerol 1,3-dimethacrylate, copolymer of acrylic and itaconic acids, water, diurethane dimethacrylate (UDMA) diphenyliodonium hexafluorophosphate, ethyl 4-dimethyl aminobenzoate (EDMAB) a . | N509492 |
Adper™ Easy Bond Self-Etch Adhesive | Bisphenol a diglycidyl ether dimethacrylate (BISGMA), 2-hydroxyethyl methacrylate, ethanol, water, phosphoric acid−6-methacryloxy-hexylesters, silane treated silica, 1,6-hexanediol dimethacrylate, copolymer of acrylic and itaconic acid, (dimethylamino)ethyl methacrylate camphorquinone, 2,4,6-trimethylbenzoyldiphenylphosphine oxide a . | N497589 |
Adper™ Scotchbond™ Multi-Purpose Adhesive | Primer: Water, 2-hydroxyethyl methacrylate (HEMA), copolymer of acrylic and itaconic acids Bond: Bisphenol a diglycidyl ether dimethacrylate (BISGMA), 2-hydroxyethyl methacrylate (hema), triphenylantimony a . |
N491015 N494505 |
The filled dentin cylinders were cut using an Isomet™ (Buehler, Lake Bluff, IL, USA) diamond saw into 2-mm thick dentin-resin composite disks under cooling water; see Fig. 1 and inset in Fig. 2 . Two or three disk specimens could be obtained from each root segment. The disks were examined for defects under a microscope (Olympus MVX10, Olympus America Inc.) with a magnification of 3.2×. Defective samples with pores on the surface, air bubbles that could be clearly seen in the composite, irregularities or cracks were removed from the study. A total of 15–17 disks were used for each group and they were stored in 0.1% thymol solution at 4 °C overnight before testing.
2.2
Diametral compression
The diametral compression test was carried out using a Universal Test Machine (858 Mini Bionix, MTS, USA), with the specimen located between two flat and parallel steel components ( Fig. 2 ). A loading rate of 0.5 mm/min was applied to fracture the samples. The load and displacement time histories were recorded during the loading process.
2.3
Acoustic emission (AE) measurement
An AE system (Physical Acoustics Corporation, NJ, USA) was used to monitor microcracking of the specimens during loading. The AE sensor was attached to the lower support plate ( Fig. 2 ). Signals detected by the sensor were passed through a preamplifier of 10-dB gain with a band pass of 100 kHz–2 MHz and a threshold set at 35 dB. The AE results were used together with the load time histories and DIC data (see below) to identify the point at which interfacial debonding first occurred.
2.4
Digital image correlation (DIC)
Surface deformation of the disks was measured by using DIC to identify interfacial debonding. This technique utilized a non-contact optical method for tracking movements of surface features. The system consisted of a high-speed CCD camera (Point Grey Grasshopper GRAS-20S4C-C) and propriety software (DaVis 7.2, LaVision-GmbH, Goettingen, Germany) for displacement and strain calculation ( Fig. 2 b). To allow deformations to be determined, the disk surface facing the CCD camera was first sprayed with a white fixation paint (Krylon products group, Cleveland, OH, USA) followed by a thin layer of charcoal particles (Sigma Aldrich, St. Louis, MO, USA). These created irregular speckles on the surface for local displacement tracking. A reference image was taken before the specimen was loaded. During loading, further images were taken at 10–20 frames per second (fps) for comparison with the reference image. The proprietary software was then used to calculate the full-field strain maps, and interfacial debonding could be identified from the strain concentration developed.
2.5
Finite element (FE) simulation
There is no simple analytical solution for the stress distribution within the dentin-composite disk. The FE method was therefore used to calculate the interfacial bond strength based on the load that caused debonding. Due to symmetry, a 2-D model representing a quadrant of the disc specimen ( Fig. 3 a) was constructed using Hypermesh 11.0 (HyperWorks, Altair Engineering, Troy, MI, USA). Appropriate boundary conditions were assigned to the vertical and horizontal planes of symmetry of the quadrant model ( Fig. 3 a). A point load was applied downward at the topmost node of the model to simulate diametral compression. To capture the stress distribution at the dentin-composite interface accurately, the regions around the interface were meshed more finely than other regions. This numerical model was then exported to ABAQUS (version 6.10-EF1; Dassault Systèmes Simulia, Waltham, MA, USA) to solve for the stresses. The model was meshed with the plane-stress elements CPS4I and CPS3 . All materials were assumed to be isotropic, homogeneous and behave linear elastically. The material properties for each component are listed in Table 2 . Fig. 3 b shows the radial stress distribution within the disk and the node at the interface (indicated by the arrow) where debonding was observed to initiate.
Material | Elastic modulus (GPa) | Poisson’s ratio | Reference |
---|---|---|---|
Z100 | 8.5 | 0.3 | |
Z250 | 12 | 0.3 | |
Dentin | 18.6 | 0.31 |
2.6
Scanning electron microscopy (SEM)
To evaluate the mode of interfacial debonding, SEM images of the fracture surfaces of the specimens were obtained using an environmental scanning electron microscope (TM-3000, Hitachi, High-Technologies Corporation, Tokyo, Japan) with the following settings: X50 magnification, compositional mode and 15 kV.
2.7
Statistical analysis
A two-way analysis of variance (ANOVA) was used to explore the effect of the composite and adhesive systems on the bond strength and the interaction between them. To determine whether a significant difference in bond strength existed among the different combinations of composites and adhesives, a one-way ANOVA was carried out using Tukey’s HSD test as the post hoc test. Fisher’s exact test was used to analyze the failure modes and their association with the composite and adhesive systems used. SAS ® 9.3 Software (SAS Institute Inc., Cary, NC, USA) was used to perform the statistical analysis.
2
Methods
2.1
Sample preparation
The root portions from approximately 30 bovine incisors were removed and cut into two halves with a diamond saw under water cooling. The cut was made perpendicularly to the long root axis, with each half having an approximate length of 6–7 mm. From these, the halves that had a root canal larger than the intended diameter were rejected. Next, the canal of each selected root segment was enlarged with a 1.9-mm diameter fiber post drill using water as coolant (3M ESPE, Dental products, St. Paul, MN, USA) to obtain a circular hole of ∼2-mm diameter. Afterwards, the root segments were trimmed down using a lathe to remove the cementum and the external layer of dentin to produce hollow dentin cylinders of 5 ± 0.05-mm outer diameter and 2.05 ± 0.05-mm inner diameter ( Fig. 1 ).
The machined dentin cylinders were randomly assigned to three bonding systems: Total-etch Adper™ Single Bond Plus (SB), Self-etch Adper™ Easy Bond Adhesive (EB) and total-etch Adper™ Scotchbond™ Multi-Purpose Adhesive (MP); see Table 1 . Each bonding system was applied to the inner surface of the hollow dentin cylinders with a disposable brush applicator provided by the manufacturer and subsequently cured according to the manufacturer’s instructions. After this, the canal of each cylinder was restored with either Filtek™ Z250 Universal Restorative or Z100™ Restorative; see also Table 1 . The composites were applied incrementally to minimize shrinkage stress, with each layer being less than 2-mm thick. The first increment was placed in the middle section of the cylinder with the aid of a composite condenser and the subsequent increments placed at its ends. The first increment was cured from both ends for 20 s each. The end increments were cured from the respective ends of the cylinder for 40s. Light curing was done with an Elipar Trilight (3M ESPE, Dental Products, St. Paul, MN, USA) curing light operated at 800 mW/cm 2 .
Product | Composition | Batch number |
---|---|---|
Z100™ Restorative | Silane treated ceramic, triethylene glycol dimethacrylate (TEGDMA), bisphenol a diglycidyl ether dimethacrylate (BISGMA), 2-benzotriazolyl-4-methylphenol a . | N362970 |
Filtek™ Z250 Universal Restorative | Silane treated ceramic, bisphenol a polyethylene glycol diether dimethacrylate (BISEMA6), diurethane dimethacrylate (UDMA), bisphenol a diglycidyl ether dimethacrylate (BISGMA), triethylene glycol dimethacrylate (TEGMA), benzotriazol, ethyl 4-dimethyl aminobenzoate (EDMAB) a . | N326080 |
Adper Single Bond Plus | Ethyl alcohol, silane treated silica (nanofiller), bisphenol a diglycidyl ether dimethacrylate (BISGMA), 2-hydroxyethyl methacrylate(HEMA), glycerol 1,3-dimethacrylate, copolymer of acrylic and itaconic acids, water, diurethane dimethacrylate (UDMA) diphenyliodonium hexafluorophosphate, ethyl 4-dimethyl aminobenzoate (EDMAB) a . | N509492 |
Adper™ Easy Bond Self-Etch Adhesive | Bisphenol a diglycidyl ether dimethacrylate (BISGMA), 2-hydroxyethyl methacrylate, ethanol, water, phosphoric acid−6-methacryloxy-hexylesters, silane treated silica, 1,6-hexanediol dimethacrylate, copolymer of acrylic and itaconic acid, (dimethylamino)ethyl methacrylate camphorquinone, 2,4,6-trimethylbenzoyldiphenylphosphine oxide a . | N497589 |
Adper™ Scotchbond™ Multi-Purpose Adhesive | Primer: Water, 2-hydroxyethyl methacrylate (HEMA), copolymer of acrylic and itaconic acids Bond: Bisphenol a diglycidyl ether dimethacrylate (BISGMA), 2-hydroxyethyl methacrylate (hema), triphenylantimony a . |
N491015 N494505 |
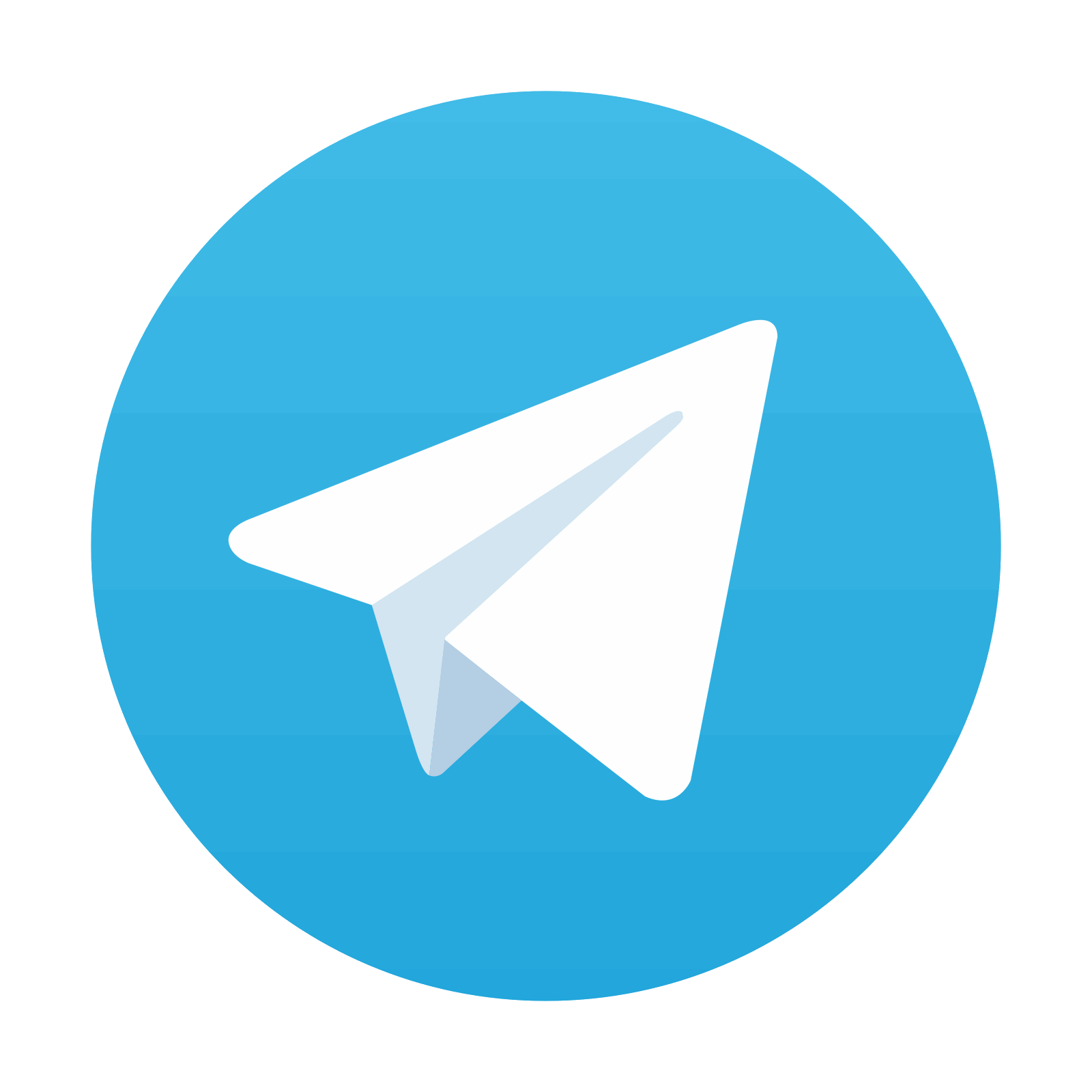
Stay updated, free dental videos. Join our Telegram channel

VIDEdental - Online dental courses
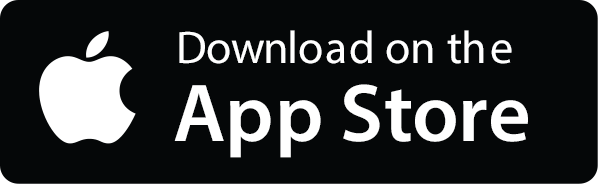
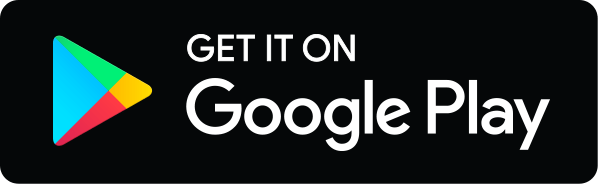
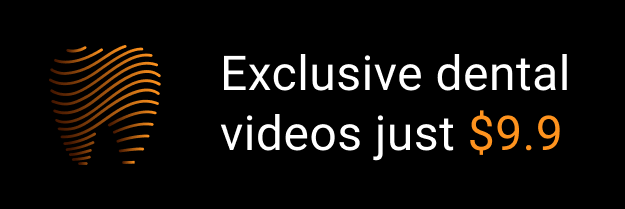