Abstract
Objectives
Half of dental restorations fail in 10 years, with secondary caries as the main reason. Calcium phosphate composites could remineralize tooth lesions. The objectives of this study were to: (1) impart antibacterial activity to a composite with nanoparticles of amorphous calcium phosphate (NACP); and (2) investigate the effect of quaternary ammonium dimethacrylate (QADM) on mechanical and dental plaque microcosm biofilm properties for the first time.
Methods
The NACP and glass particles were filled into a dental resin that contained bis(2-methacryloyloxy-ethyl) dimethyl-ammonium bromide, the QADM. NACP nanocomposites containing 0%, 7%, 14%, and 17.5% of QADM by mass, respectively, were photo-cured. A commercial composite with no antibacterial activity was used as control. Mechanical properties were measured in three-point flexure. A human saliva microcosm model was used to grow biofilms on composites. Live/dead assay, metabolic activity, colony-forming unit (CFU) counts, and lactic acid production of biofilms on the composites were measured.
Results
Increasing QADM mass fraction monotonically reduced the biofilm viability, CFU and lactic acid. Biofilms on NACP nanocomposite with 17.5% QADM had metabolic activity that was 30% that on a commercial composite control ( p < 0.05). Total microorganisms, total streptococci, and mutans streptococci CFU counts (mean ± sd; n = 6) on composite control was 6-fold those on NACP + 17.5% QADM nanocomposite. Composite control had long strings of bacterial cells with normal short-rod shapes, while some cells on NACP–QADM nanocomposites disintegrated into pieces. Adding QADM to NACP did not decrease the composite strength and elastic modulus, which matched ( p > 0.1) those of a commercial composite without Ca-PO 4 or antibacterial activity.
Significance
A dental plaque microcosm model was used to evaluate the novel NACP–QADM nanocomposite. The nanocomposite greatly reduced the biofilm viability, metabolic activity and lactic acid, while its mechanical properties matched those of a commercial composite. NACP–QADM nanocomposite with calcium phosphate fillers, good mechanical properties and a strong antibacterial activity may have potential for anti-biofilm and anti-caries restorations.
1
Introduction
Dental caries is a dietary carbohydrate-modified bacterial infectious disease, and is one of the most common bacterial infections in humans . Demineralization of enamel and dentin is caused by acid generated by bacterial biofilms (dental plaque) in the presence of fermentable carbohydrates . Nearly 200 million dental restorations are placed annually in the U.S. . Resin composites are popular filling materials due to their esthetics and direct-filling capabilities . Composite filler particles, resin compositions, and handling and polymerization properties have all been greatly improved . However, studies have found that composites had more biofilms and plaques than other restorative materials . These plaques could lead to secondary caries, which was found to be a main cause for restoration failure . Currently, half of all restorations fail within 10 years, and replacing them consumes 50% to 70% of the dentist’s time . In the U.S. alone, replacement dentistry costs $5 billion annually .
Therefore, antibacterial composites are developed with the purpose to inhibit secondary caries . Quaternary ammonium salts (QAS) are antibacterial and used in water treatment, surface coatings, and the food industry . Previous studies incorporated polymerizable QAS monomers into resins by forming a covalent bond with the polymer network, thus immobilizing the QAS in the composite . Resins with 12-methacryloyloxydodecylpyridinium bromide (MDPB) effectively reduced the Streptococcus mutans ( S. mutans ) viability and growth . Other antibacterial agents, including methacryloxylethyl cetyl dimethyl ammonium chloride and cetylpyridinium chloride, were also added into dental resins .
Another approach to combat caries is to develop calcium phosphate (CaP)-resin composites . CaP composites could release supersaturating levels of calcium (Ca) and phosphate (PO 4 ) ions and remineralize tooth lesions . Traditional CaP composites contained particles of about 1–55 μm . Recent studies developed nanocomposites using calcium phosphate and calcium fluoride nanoparticles with sizes of 50–100 nm . In one study, nanoparticles of amorphous calcium phosphate (NACP) of 116 nm were incorporated into a composite . The advantage of NACP nanocomposite was that it released Ca and PO 4 similar to, but its mechanical properties were 2–3 fold higher than, those of traditional CaP composites . The NACP nanocomposite was “smart” and greatly increased the ion release at acidic pH, when these ions are most needed to combat caries . Another study showed that, when placed in a lactic acid solution with a cariogenic pH of 4, the NACP nanocomposite quickly neutralized the acid and increased the pH to a safe level of above 6 . The pH stayed at about 4 when commercial restorative controls were tested in the same manner .
There has been little report on imparting an antibacterial activity to CaP composites. Recently, a quaternary ammonium dimethacrylate (QADM) was incorporated into the NACP nanocomposite to combine remineralizing and antibacterial properties in the same composite . A single bacterial species, S. mutans , was tested in that study . However, dental plaque is a complicated ecosystem with about 1000 different bacterial species . Therefore, several previous studies used dental plaque microcosm models, which are laboratory models inoculated with saliva from human donors, to examine antibacterial dental materials .
Accordingly, the objective of this study was to investigate the effect of QADM mass fraction in NACP nanocomposite on dental plaque microcosm biofilms for the first time. It was hypothesized that: (1) the capability of the NACP nanocomposite to hinder microcosm biofilm growth would be directly proportional to the QADM mass fraction; (2) the NACP–QADM nanocomposites would have much lower biofilm viability, metabolic activity and lactic acid, than those of a commercial control composite; (3) the NACP–QADM nanocomposite would have mechanical properties matching those of the commercial control composite.
2
Materials and methods
2.1
NACP and glass filler particles
NACP [Ca 3 (PO 4 ) 2 ] was synthesized via a spray-drying technique as recently described . Briefly, calcium carbonate and dicalcium phosphate anhydrous were dissolved into an acetic acid solution to obtain Ca and PO 4 ionic concentrations of 8 mmol/L and 5.333 mmol/L, respectively, yielding a Ca/P molar ratio of 1.5. This solution was sprayed into a heated chamber, and an electrostatic precipitator was used to collect the dried particles. This yielded NACP with a mean particle size of 116 nm . As a co-filler to reinforce the composite, a barium boroaluminosilicate glass of a mean particle size of 1.4 μm (Caulk/Dentsply, Milford, DE) was silanized with 4% 3-methacryloxypropyltrimethoxysilane and 2% n -propylamine. These fillers were used in the composites as described below.
2.2
QADM resin composites
BisGMA (bisphenol glycidyl dimethacrylate) and TEGDMA (triethylene glycol dimethacrylate) (Esstech, Essington, PA) were mixed at a mass ratio of 1:1, and rendered light-curable with 0.2% camphorquinone and 0.8% ethyl 4- N , N -dimethylaminobenzoate. The QADM was bis(2-methacryloyloxy-ethyl) dimethyl-ammonium bromide, which was synthesized as described recently . Its synthesis employed a modified Menschutkin reaction in which a tertiary amine group was reacted with an organo-halide. 10 mmol of 2-( N , N -dimethylamino)ethyl methacrylate (DMAEMA, Sigma, St. Louis, MO) and 10 mmol of 2-bromoethyl methacrylate (BEMA, Monomer-Polymer and Dajec Labs, Trevose, PA) were combined with 3 g of ethanol in a 20-mL scintillation vial. After stirring at 60 °C for 24 h, the solvent was removed and the QADM was obtained in the form of a clear, colorless and viscous liquid. This method is desirable because the reaction products were generated at quantitative amounts and required no further purification. The QADM was mixed with the photo-activated BisGMA–TEGDMA (referred to as BT). Previous studies used a single QADM mass fraction of 20% . The present study tested the following QADM/(BT + QADM) mass fractions: 0%, 20%, 40%, and 50%. Each resin was mixed with 30% NACP and 35% glass fillers, with a total filler level of 65%, to yield a cohesive paste. Because there was 35% resin in the composite, the QADM mass fractions in the composite were: 0%, 7%, 14%, and 17.5%.
For mechanical testing, the paste was placed into rectangular molds of 2 × 2 × 25 mm. For biofilm testing, disk molds of 9 mm in diameter and 2 mm in thickness were used. A commercial composite, Renamel (Cosmedent, Chicago, IL), was also tested. It consisted of nanofillers of 20–40 nm with 60% fillers in a multifunctional methacrylate ester resin. Renamel is designated as “composite control”. All the specimens were photo-polymerized (Triad 2000, Dentsply, York, PA) for 1 min on each side. This yielded five composites: composite control, NACP + 0% QADM, NACP + 7% QADM, NACP + 14% QADM, NACP + 17.5% QADM. Six specimens per composite were used for each test ( n = 6).
2.3
Mechanical properties
A computer-controlled Universal Testing Machine (5500R, MTS, Cary, NC) was used. Composite specimens were immersed in distilled water at 37 °C for 1 d, and then fractured in three-point flexure with a 10 mm span at a crosshead-speed of 1 mm/min. The specimens were wet and not dried, and were fractured within a few minutes after being taken out of the water. Flexural strength ( S ) was calculated as: S = 3 P max L /(2 bh 2 ), where P max is the fracture load, L is span, b is specimen width and h is thickness. Elastic modulus ( E ) was calculated as: E = ( P / d )( L 3 /[4 bh 3 ]), where load P divided by displacement d is the slope in the linear elastic region.
2.4
Saliva collection for biofilm inoculum
The microcosm model of this study was approved by the University of Maryland. Saliva is ideal for growing dental plaque microcosm biofilms in vitro , with the advantage of maintaining much of the complexity and heterogeneity of the dental plaque in vivo . Saliva was collected from a healthy adult donor, following a previous study . The donor had natural dentition without active caries or periopathology, and without the use of antibiotics within the last 3 months. The donor did not brush teeth for 24 h and abstained from food/drink intake for 2 h prior to donating saliva. Stimulated saliva was collected during parafilm chewing and was kept on ice. The saliva was diluted in sterile glycerol to a concentration of 70%, and stored at −80 °C .
2.5
Dental plaque microcosm biofilm formation
The saliva–glycerol stock was added, with 1:50 final dilution, into the growth medium as inoculum. The growth medium contained mucin (type II, porcine, gastric) at a concentration of 2.5 g/L; bacteriological peptone, 2.0 g/L; tryptone, 2.0 g/L; yeast extract, 1.0 g/L; NaCl, 0.35 g/L, KCl, 0.2 g/L; CaCl 2 , 0.2 g/L; cysteine hydrochloride, 0.1 g/L; hemin, 0.001 g/L; vitamin K1, 0.0002 g/L, at pH 7 . Composite disks were sterilized in ethylene oxide (Anprolene AN 74i, Andersen, Haw River, NC). 1.5 mL of inoculum was added to each well of 24-well plates with a composite disk, and incubated in 5% CO 2 at 37 °C for 8 h. Then, the disks were transferred to new 24-well plates filled with fresh medium and incubated. After 16 h, the disks were transferred to new 24-well plates with fresh medium and incubated for 24 h. This totals 48 h of incubation, which was adequate to form plaque microcosm biofilms .
2.6
Live/dead assay and bacterial viability
After 48 h growth, the plaque microcosm biofilms on the disks were gently washed three times with phosphate buffered saline (PBS), and then stained using the BacLight live/dead bacterial viability kit (Molecular Probes, Eugene, OR). Live bacteria were stained with Syto 9 to produce a green fluorescence, and bacteria with compromised membranes were stained with propidium iodide to produce a red fluorescence. Disks were examined using an inverted epifluorescence microscope (Eclipse TE2000-S, Nikon, Melville, NY).
Disks with 48-h biofilms were rinsed with PBS and then immersed in 1% glutaraldehyde in PBS for 4 h at 4 °C. The specimens were rinsed with PBS, subjected to graded ethanol dehydrations, and rinsed twice with 100% hexamethyldisilazane. The specimens were then sputter-coated with gold and examined via scanning electron microscopy (SEM, Quanta 200, FEI, Hillsboro, OR).
2.7
MTT assay of metabolic activity
The MTT (3-[4,5-dimethylthiazol-2-yl]-2,5-diphenyltetrazolium bromide) assay was used to examine the metabolic activity of biofilms . MTT is a colorimetric assay that measures the enzymatic reduction of MTT, a yellow tetrazole, to formazan. Each disk with 48-h biofilm was transferred to a new 24-well plate, then 1 mL of MTT dye (0.5 mg/mL MTT in PBS) was added to each well and incubated at 37 °C in 5% CO 2 for 1 h. During this process, metabolically active bacteria reduced the MTT to purple formazan. After 1 h, the disks were transferred to a new 24-well plate, 1 mL of dimethyl sulfoxide (DMSO) was added to solubilize the formazan crystals, and the plate was incubated for 20 min with gentle mixing at room temperature in the dark. After mixing via pipetting, 200 μL of the DMSO solution from each well was transferred to a 96-well plate, and the absorbance at 540 nm (optical density OD540) was measured via a microplate reader (SpectraMax M5, Molecular Devices, Sunnvale, CA). A higher absorbance is related to a higher formazan concentration, which indicates a higher metabolic activity in the biofilm on the disk.
2.8
Colony forming unit (CFU) counts and lactic acid production
Each disk with 48-h biofilm was rinsed with cysteine peptone water (CPW) to remove loose bacteria. The disks were transferred to 24-well plates containing buffered peptone water (BPW) plus 0.2% sucrose, and incubated in 5% CO 2 at 37 °C for 3 h to allow the biofilms to produce acid. Subsequently, the BPW solutions were stored for lactate analysis.
The disks with biofilms were then transferred into tubes with 2 mL CPW, and the biofilms were harvested by sonication and vortexing at the maximum speed for 20 s using a vortex mixer (Fisher, Pittsburgh, PA). Three types of agar plates were used to measure the CFU counts to assess the microorganism viability. First, tryptic soy blood agar culture plates were used to determine total microorganisms . Second, mitis salivarius agar (MSA) culture plates, containing 15% sucrose, were used to determine total streptococci . This is because MSA contains selective agents crystal violet, potassium tellurite and trypan blue, which inhibit most Gram-negative bacilli and most Gram-positive bacteria except streptococci, thus enabling streptococci to grow . Third, cariogenic mutans streptococci is known to be resistant to bacitracin, and this property is often used to isolate mutans streptococci from the highly heterogeneous oral microflora. Hence, MSA agar culture plates plus 0.2 units of bacitracin per mL was used to determine mutans streptococci. The purpose of measuring these three CFU counts was to provide antibacterial information on not only the total microorganisms, but also mutans streptococci, in the plaque microcosm biofilm. The mutans streptococci group consists of S. mutans and S. sobrinus , both species playing a key role in tooth decay.
Lactate concentrations in the BPW solutions were determined using an enzymatic (lactate dehydrogenase) method, following previous studies . The microplate reader was used to measure the absorbance at 340 nm (optical density OD 340 ) for the collected BPW solutions. Standard curves were prepared using a lactic acid standard (Supelco, Bellefonte, PA).
One-way and two-way analyses of variance (ANOVA) were performed to detect the significant effects of the variables. Tukey’s multiple comparison test was used to compare the data at a p value of 0.05.
2
Materials and methods
2.1
NACP and glass filler particles
NACP [Ca 3 (PO 4 ) 2 ] was synthesized via a spray-drying technique as recently described . Briefly, calcium carbonate and dicalcium phosphate anhydrous were dissolved into an acetic acid solution to obtain Ca and PO 4 ionic concentrations of 8 mmol/L and 5.333 mmol/L, respectively, yielding a Ca/P molar ratio of 1.5. This solution was sprayed into a heated chamber, and an electrostatic precipitator was used to collect the dried particles. This yielded NACP with a mean particle size of 116 nm . As a co-filler to reinforce the composite, a barium boroaluminosilicate glass of a mean particle size of 1.4 μm (Caulk/Dentsply, Milford, DE) was silanized with 4% 3-methacryloxypropyltrimethoxysilane and 2% n -propylamine. These fillers were used in the composites as described below.
2.2
QADM resin composites
BisGMA (bisphenol glycidyl dimethacrylate) and TEGDMA (triethylene glycol dimethacrylate) (Esstech, Essington, PA) were mixed at a mass ratio of 1:1, and rendered light-curable with 0.2% camphorquinone and 0.8% ethyl 4- N , N -dimethylaminobenzoate. The QADM was bis(2-methacryloyloxy-ethyl) dimethyl-ammonium bromide, which was synthesized as described recently . Its synthesis employed a modified Menschutkin reaction in which a tertiary amine group was reacted with an organo-halide. 10 mmol of 2-( N , N -dimethylamino)ethyl methacrylate (DMAEMA, Sigma, St. Louis, MO) and 10 mmol of 2-bromoethyl methacrylate (BEMA, Monomer-Polymer and Dajec Labs, Trevose, PA) were combined with 3 g of ethanol in a 20-mL scintillation vial. After stirring at 60 °C for 24 h, the solvent was removed and the QADM was obtained in the form of a clear, colorless and viscous liquid. This method is desirable because the reaction products were generated at quantitative amounts and required no further purification. The QADM was mixed with the photo-activated BisGMA–TEGDMA (referred to as BT). Previous studies used a single QADM mass fraction of 20% . The present study tested the following QADM/(BT + QADM) mass fractions: 0%, 20%, 40%, and 50%. Each resin was mixed with 30% NACP and 35% glass fillers, with a total filler level of 65%, to yield a cohesive paste. Because there was 35% resin in the composite, the QADM mass fractions in the composite were: 0%, 7%, 14%, and 17.5%.
For mechanical testing, the paste was placed into rectangular molds of 2 × 2 × 25 mm. For biofilm testing, disk molds of 9 mm in diameter and 2 mm in thickness were used. A commercial composite, Renamel (Cosmedent, Chicago, IL), was also tested. It consisted of nanofillers of 20–40 nm with 60% fillers in a multifunctional methacrylate ester resin. Renamel is designated as “composite control”. All the specimens were photo-polymerized (Triad 2000, Dentsply, York, PA) for 1 min on each side. This yielded five composites: composite control, NACP + 0% QADM, NACP + 7% QADM, NACP + 14% QADM, NACP + 17.5% QADM. Six specimens per composite were used for each test ( n = 6).
2.3
Mechanical properties
A computer-controlled Universal Testing Machine (5500R, MTS, Cary, NC) was used. Composite specimens were immersed in distilled water at 37 °C for 1 d, and then fractured in three-point flexure with a 10 mm span at a crosshead-speed of 1 mm/min. The specimens were wet and not dried, and were fractured within a few minutes after being taken out of the water. Flexural strength ( S ) was calculated as: S = 3 P max L /(2 bh 2 ), where P max is the fracture load, L is span, b is specimen width and h is thickness. Elastic modulus ( E ) was calculated as: E = ( P / d )( L 3 /[4 bh 3 ]), where load P divided by displacement d is the slope in the linear elastic region.
2.4
Saliva collection for biofilm inoculum
The microcosm model of this study was approved by the University of Maryland. Saliva is ideal for growing dental plaque microcosm biofilms in vitro , with the advantage of maintaining much of the complexity and heterogeneity of the dental plaque in vivo . Saliva was collected from a healthy adult donor, following a previous study . The donor had natural dentition without active caries or periopathology, and without the use of antibiotics within the last 3 months. The donor did not brush teeth for 24 h and abstained from food/drink intake for 2 h prior to donating saliva. Stimulated saliva was collected during parafilm chewing and was kept on ice. The saliva was diluted in sterile glycerol to a concentration of 70%, and stored at −80 °C .
2.5
Dental plaque microcosm biofilm formation
The saliva–glycerol stock was added, with 1:50 final dilution, into the growth medium as inoculum. The growth medium contained mucin (type II, porcine, gastric) at a concentration of 2.5 g/L; bacteriological peptone, 2.0 g/L; tryptone, 2.0 g/L; yeast extract, 1.0 g/L; NaCl, 0.35 g/L, KCl, 0.2 g/L; CaCl 2 , 0.2 g/L; cysteine hydrochloride, 0.1 g/L; hemin, 0.001 g/L; vitamin K1, 0.0002 g/L, at pH 7 . Composite disks were sterilized in ethylene oxide (Anprolene AN 74i, Andersen, Haw River, NC). 1.5 mL of inoculum was added to each well of 24-well plates with a composite disk, and incubated in 5% CO 2 at 37 °C for 8 h. Then, the disks were transferred to new 24-well plates filled with fresh medium and incubated. After 16 h, the disks were transferred to new 24-well plates with fresh medium and incubated for 24 h. This totals 48 h of incubation, which was adequate to form plaque microcosm biofilms .
2.6
Live/dead assay and bacterial viability
After 48 h growth, the plaque microcosm biofilms on the disks were gently washed three times with phosphate buffered saline (PBS), and then stained using the BacLight live/dead bacterial viability kit (Molecular Probes, Eugene, OR). Live bacteria were stained with Syto 9 to produce a green fluorescence, and bacteria with compromised membranes were stained with propidium iodide to produce a red fluorescence. Disks were examined using an inverted epifluorescence microscope (Eclipse TE2000-S, Nikon, Melville, NY).
Disks with 48-h biofilms were rinsed with PBS and then immersed in 1% glutaraldehyde in PBS for 4 h at 4 °C. The specimens were rinsed with PBS, subjected to graded ethanol dehydrations, and rinsed twice with 100% hexamethyldisilazane. The specimens were then sputter-coated with gold and examined via scanning electron microscopy (SEM, Quanta 200, FEI, Hillsboro, OR).
2.7
MTT assay of metabolic activity
The MTT (3-[4,5-dimethylthiazol-2-yl]-2,5-diphenyltetrazolium bromide) assay was used to examine the metabolic activity of biofilms . MTT is a colorimetric assay that measures the enzymatic reduction of MTT, a yellow tetrazole, to formazan. Each disk with 48-h biofilm was transferred to a new 24-well plate, then 1 mL of MTT dye (0.5 mg/mL MTT in PBS) was added to each well and incubated at 37 °C in 5% CO 2 for 1 h. During this process, metabolically active bacteria reduced the MTT to purple formazan. After 1 h, the disks were transferred to a new 24-well plate, 1 mL of dimethyl sulfoxide (DMSO) was added to solubilize the formazan crystals, and the plate was incubated for 20 min with gentle mixing at room temperature in the dark. After mixing via pipetting, 200 μL of the DMSO solution from each well was transferred to a 96-well plate, and the absorbance at 540 nm (optical density OD540) was measured via a microplate reader (SpectraMax M5, Molecular Devices, Sunnvale, CA). A higher absorbance is related to a higher formazan concentration, which indicates a higher metabolic activity in the biofilm on the disk.
2.8
Colony forming unit (CFU) counts and lactic acid production
Each disk with 48-h biofilm was rinsed with cysteine peptone water (CPW) to remove loose bacteria. The disks were transferred to 24-well plates containing buffered peptone water (BPW) plus 0.2% sucrose, and incubated in 5% CO 2 at 37 °C for 3 h to allow the biofilms to produce acid. Subsequently, the BPW solutions were stored for lactate analysis.
The disks with biofilms were then transferred into tubes with 2 mL CPW, and the biofilms were harvested by sonication and vortexing at the maximum speed for 20 s using a vortex mixer (Fisher, Pittsburgh, PA). Three types of agar plates were used to measure the CFU counts to assess the microorganism viability. First, tryptic soy blood agar culture plates were used to determine total microorganisms . Second, mitis salivarius agar (MSA) culture plates, containing 15% sucrose, were used to determine total streptococci . This is because MSA contains selective agents crystal violet, potassium tellurite and trypan blue, which inhibit most Gram-negative bacilli and most Gram-positive bacteria except streptococci, thus enabling streptococci to grow . Third, cariogenic mutans streptococci is known to be resistant to bacitracin, and this property is often used to isolate mutans streptococci from the highly heterogeneous oral microflora. Hence, MSA agar culture plates plus 0.2 units of bacitracin per mL was used to determine mutans streptococci. The purpose of measuring these three CFU counts was to provide antibacterial information on not only the total microorganisms, but also mutans streptococci, in the plaque microcosm biofilm. The mutans streptococci group consists of S. mutans and S. sobrinus , both species playing a key role in tooth decay.
Lactate concentrations in the BPW solutions were determined using an enzymatic (lactate dehydrogenase) method, following previous studies . The microplate reader was used to measure the absorbance at 340 nm (optical density OD 340 ) for the collected BPW solutions. Standard curves were prepared using a lactic acid standard (Supelco, Bellefonte, PA).
One-way and two-way analyses of variance (ANOVA) were performed to detect the significant effects of the variables. Tukey’s multiple comparison test was used to compare the data at a p value of 0.05.
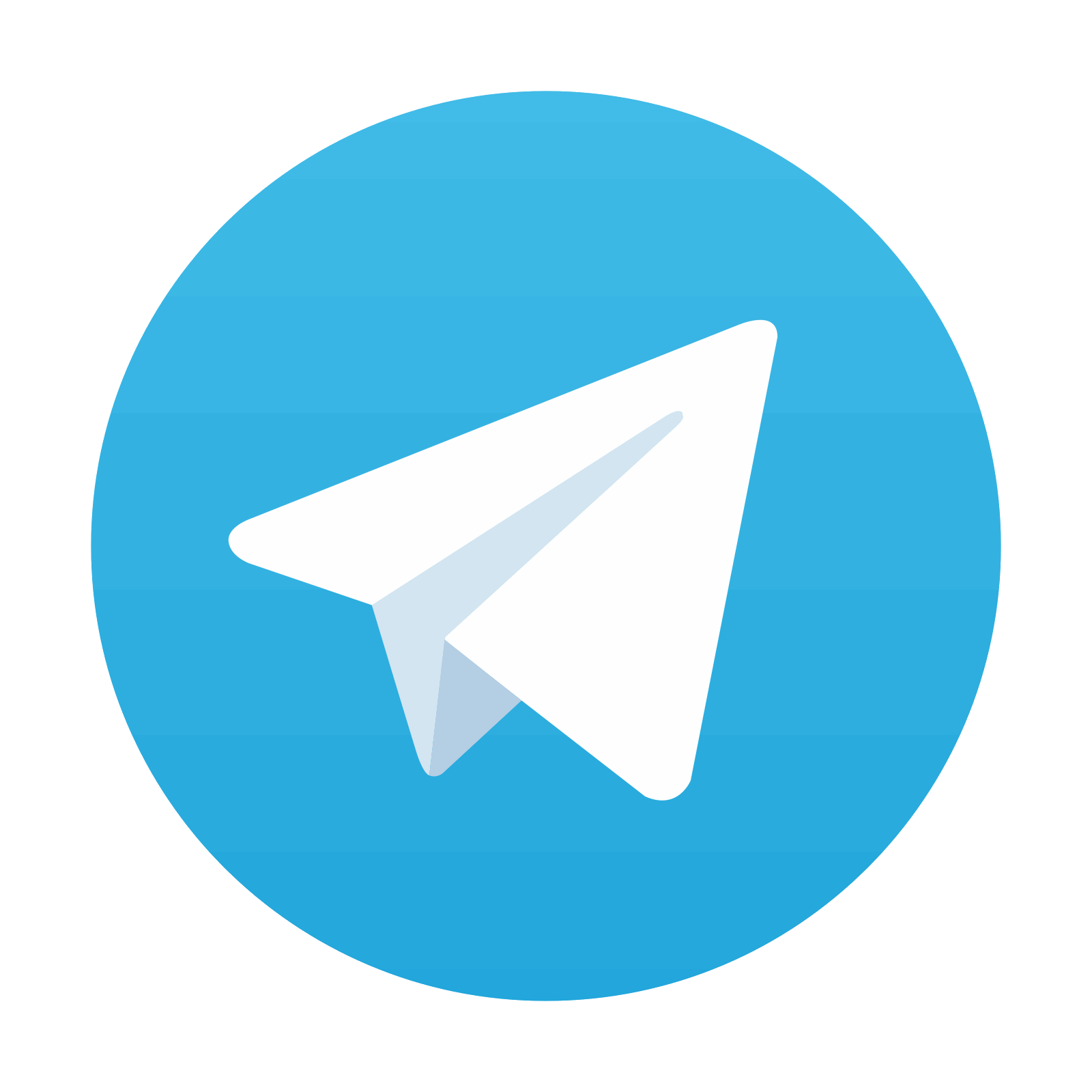
Stay updated, free dental videos. Join our Telegram channel

VIDEdental - Online dental courses
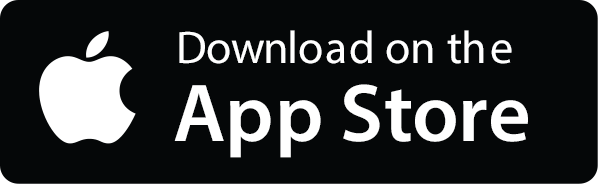
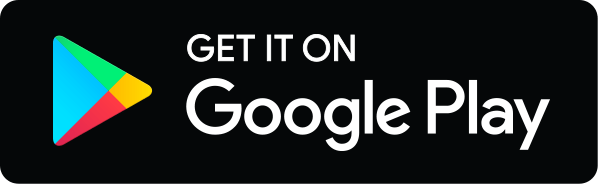