Abstract
The aim of this study was to evaluate the degeneration and regeneration of a sensory nerve and a motor nerve at the histological level after a crush injury. Twenty-five female Wistar rats had their mental nerve and the buccal branch of their facial nerve compressed unilaterally against a glass rod for 30 s. Specimens of the compressed nerves and the corresponding control nerves were dissected at 3, 7, and 19 days after surgery. Nerve cross-sections were stained with osmium tetroxide and toluidine blue and analysed using two-dimensional stereology. We found differences between the two nerves both in the normal anatomy and in the regenerative pattern. The mental nerve had a larger cross-sectional area including all tissue components. The mental nerve had a larger volume fraction of myelinated axons and a correspondingly smaller volume fraction of endoneurium. No differences were observed in the degenerative pattern; however, at day 19 the buccal branch had regenerated to the normal number of axons, whereas the mental nerve had only regained 50% of the normal number of axons. We conclude that the regenerative process is faster and/or more complete in the facial nerve (motor function) than it is in the mental nerve (somatosensory function).
Nerve injury is a relatively frequent complication after orthognathic surgery in the mandible, and both temporary and permanent nerve damage may occur after such operations. Nerve injury can be caused by direct mechanical trauma to the nerve or its arterial supply, or indirectly by compression from oedema or haematoma formation. The local anaesthetic agents may also occasionally induce neurotoxic nerve damage. The hazard rate depends on the orthognathic surgical procedure. The sagittal split osteotomy has been reported to generate sensory disturbances at a higher rate than the intraoral subcondylar ramus osteotomy followed by the extraoral approach. The reported incidence of neurosensory disturbances ranges from 9% to 85% following sagittal split osteotomy, and from 0% to 35% following vertical ramus osteotomy. Muscle weakness following the same surgical procedures has been reported with much lower incidences than neurosensory disturbances. This difference may be attributed to the anatomical positions of the facial nerve and the inferior alveolar nerve in relation to the surgical procedure performed, where the latter may be more easily damaged directly during orthognathic operations.
Alternative non-anatomical explanations for this differential incidence, such as a difference in the degenerative and/or regenerative capacity of the nerves following a trauma to the nerve, are also conceivable. There are some reports focusing on the potential difference between sensory and motor nerve fibres in degenerative and/or regenerative capacity (summarized in Table 1 ). As regards nerve degeneration, no difference between sensory and motor nerve fibres has, to our knowledge, been reported. However, as regards the regenerative process, the results are quite variable, with some studies showing the motor axons to regenerate faster, but others showing the sensory fibres to regenerate faster, and still others finding no difference.
Study | Animal and nerve(s) damaged | Nerve lesion model | Evaluation method(s) | Examination time(s) | Conclusion(s) |
---|---|---|---|---|---|
Moldovan et al. | Cat; tibial | Crush lesion and complete transection | Electrophysiology | Every 4–6 days in the first month; monitoring for up to 140 days | Similar regeneration |
Jenq and Coggeshall | Neonatal and adult rat; sciatic | Crush lesion | Axon counts in motor and sensory nerves arising tributary from the sciatic nerve | Lesion evaluated 1 h post lesion; regeneration evaluated 8 weeks post lesion | In neonatal rats the motor fibres regenerate to a greater than normal number, whereas sensory fibres do not; in adult rats both sensory and motor fibres regenerate to supernumerary numbers |
da Silva et al. | Mouse; sciatic | Transection with a final nerve gap length of 4–5 mm | Counting of retrograde HRP-labelled cell profiles in the lumbar enlargement of the spinal cord and the L3–L5 dorsal root ganglia corrected with Abercrombie’s formula | 2, 4, and 6 weeks | More (as a percentage of the normal number) motor cell profiles were counted both 4 and 6 weeks following the lesion |
Sanger et al. | Rabbit; sciatic | Transection | Histochemical identification of carbonic anhydrase and cholinesterase | 1, 2, 3, 4, 9, and 35 days | Sensory axons regenerated earlier and to a greater degree than motor axons in the developing neuroma |
Madorsky et al. | Rat; sciatic | Transection | Collagen conduits, HRP retrograde labelling | Different observation times up to 6 months post lesion | Sensory axons regenerate faster |
Suzuki et al. | Rat; sciatic | Transection | Counting retrograde Fluoro-Gold-labelled cell profiles in the lumbar enlargement of the spinal cord and the L3–L5 dorsal root ganglia corrected with Abercrombie’s formula | 5, 7, and 14 days | Sensory axons regenerate faster |
Kawasaki et al. | Rat; peroneal | Transection | Cholinesterase staining (Karnovsky’s staining) for motor axons, carbonic anhydrase staining for sensory axons, and anti-neurofilament immunohistochemical staining for all axons | 2, 4, and 12 weeks | Myelinated sensory axons regenerate faster in the early stage of nerve regeneration, and regeneration of the myelinated motor axons is prominent in the subsequent stage |
Forman and Berenberg | Rat; sciatic | Crush lesion | Labelling regenerating axons with axonally transported radioactive proteins [3H] proline | 2–14 days | Sensory faster regeneration |
The aims of this study were to compare the quantitative aspects of the degenerative and regenerative patterns following experimental nerve trauma in a motor nerve and a sensory nerve relevant to the orthognathic surgical procedures, i.e. the mental nerve and the buccal branch of the facial nerve. To this end we examined the nerves with unbiased stereological techniques to circumvent potential biases that may arise when using older morphometric methods.
Materials and methods
Animals, surgery, and nerve compression
Twenty-five adult female Wistar rats from a commercial breeder (Taconic, Denmark) were used. They were kept in standard cages in a 12:12 h light–dark cycle and had free access to tap water and standard rat food. The animals were kept at the animal facility for 2 weeks prior to the study start to ensure adaptation to the environment. At the study start the rats weighed 250 ± 5 g; 2 weeks later they weighed 290 ± 7 g and a further 2 weeks later they weighed 310 ± 6 g. The rats were randomized into a group where the left mental nerve and the left buccal branch of the facial nerve were subjected to compression ( n = 13) and a group where the corresponding right nerves were subjected to compression ( n = 12). All surgical procedures were performed by the same investigator. The first operation was chosen randomly by flipping a coin to be the left side, and thereafter the operations were performed alternately between the right and the left side to cancel out an eventual effect of the investigator becoming more adept at the surgery. The surgery was performed over a period of 25 days. Rats were anaesthetized with an intraperitoneal injection of sodium pentobarbital 30 mg/kg (Pentobarbital Natrium vet. APL, Sweden, 60 mg/ml) and the relevant side of the face was shaved. Using an aseptic technique, the plane superficial to the muscular layer in the buccal area was exposed via a longitudinal incision to identify the buccal branch of the facial nerve (BF), while a submandibular incision was done to allow access to the mental foramen and the mental nerve (MN). The nerves were identified and isolated with blunt dissection using a pair of microsurgical scissors and a dissection microscope (Wild Heerbrugg M651). The actual nerves were compressed by tying them to a glass rod for 30 s. The wound was sutured with an absorbable suture (Vicryl 5-0). Rats were returned to their home cage to recover without antibiotic or analgesic treatment. One rat was excluded from the study for technical reasons. All experimental procedures were conducted in accordance with current national regulations issued by The Swedish Council on Animal Care. We chose female rats because they tend to accept the sutures better than male rats. Whether female rats are more prone to nerve damage than males remains to be elucidated. It is consistently reported that nerve damage caused by local anaesthetics is more often reported in females than in males (Hillerup, personal communication), but the reason for this is at present not fully elucidated. Apart from a gender difference in sensitivity, the reason could also be that females receive more treatments or that they are more willing to report side effects of the treatment.
Tissue preparation
The animals were sacrificed at 3, 7, or 19 days after surgery by perfusion fixation in deep sedation using 60 mg/kg sodium pentobarbital. After a 15-s initial perfusion with saline through the ascending aorta, the animals were perfused for 10 min with 5% glutaraldehyde in 0.75 Tyrode’s buffer. The heads were kept at 5 °C in the same fixative for at least 30 days before dissection. Eight–12-mm long segments of the relevant nerves were dissected ( Fig. 1 ). After three rinses in 0.15 M phosphate buffer (pH 7.4), the specimens were post fixed in 2% OsO 4 in 0.12 M sodium cacodylate buffer (pH 7.2) for 2 h. The specimens were subsequently dehydrated in ethanol, transferred to propylene oxide, and embedded in Epon resin, in accordance with standard procedures. Semi-thin (1-μm) sections were cut perpendicular to the long axis of the nerve segment with a Reichert Ultracut S microtome (Leica, Herlev, Denmark), stained with toluidine blue, and a cover-slip placed with Pertex mounting medium.

Stereological analysis
The stereological analyses are described in detail elsewhere. The total numbers of myelinated axons were estimated by the two-dimensional (2D) fractionating technique, and the volume fractions and cross-sectional areas were estimated by point counting. Briefly, the numbers of specified axon profiles were counted with counting frames positioned in a systematic random manner over the entire cross-section. The area of the counting frames constituted a known fraction of the nerve cross-section, and the total number of axon profiles was thus estimated as the number of profiles counted in the known fraction divided by the sampling fraction. The myelinated axons were classified into the following four subgroups depending on their morphology: (1) normal axons where the axons had normal morphology, (2) demyelinated axons where the axons had lost their entire myelin sheath but the axonal body could still be identified, (3) degenerating axons where the axons had different degenerative morphology but retained all or part of their myelin sheath, and (4) regenerating axons where the axons were much smaller than normal axons, had a thin myelin sheath and a lightly stained axoplasm, and were almost always associated with darkly stained Schwann cell nuclei (see examples in Fig. 2 ).

The cross-sectional areas of various tissue components were estimated by the point counting technique by placing a point grid randomly on the nerve cross-section and counting the number of points hitting the tissue component of interest. The cross-sectional area of the tissue component is the number of points hitting the structure multiplied by the area associated with each point in the grid. The following tissue components were distinguished: (1) the myelinated axons with their myelin sheath, (2) the endoneurium including the unmyelinated axons and the cytoplasm of residing cells, and (3) cell nuclei of residing cells. The collected data from the point counting were also used to estimate the volume fractions of the various tissue components. The volume fraction of tissue equals the area fraction of the tissue.
The practical procedures were carried out on video images of microscopic fields transmitted to a computer screen onto which the relevant test probe was superimposed using the CAST-Grid software (Olympus, Denmark). The entire nerve was delineated at a low magnification (×102 using a ×2 PlanApo objective). Both number estimation and area estimation were performed at a final magnification of ×5125 using a ×100 UPlanApo oil immersion objective (NA = 1.35) to which the objective used for delineation was para-centered. At high magnification the computer-controlled stage of the Olympus BX51 microscope was programmed to move the section systematically, random in a raster pattern within the delineated region, with step lengths in the x – and y -axes of 25 μm for the number estimation and of 40 μm for the area estimation, respectively. For the number estimation, the image of an unbiased counting frame (of 30.1 μm 2 area) was superimposed onto the microscope image at each point in the raster pattern and axons were counted using the unbiased counting rules. The sampling fraction was thus 0.04816. In analogy, for the area estimation, a single point was superimposed onto the image and the area per point was thus 1600 μm 2 . All stereological analyses were performed by the same investigator. Nerve cross-sections that were of substandard quality were excluded from the study. Five BF and 12 MN segments were excluded from the number estimation and three BF and nine MN segments were excluded from the area and volume fraction estimation.
Statistics
In order to test for dissimilarity between the MN and BF as regards the normal anatomy, we deselected the paired t -test (even though it would have been the optimal test) since not all animals were represented by both the MN and the BF. The uncrushed control nerves from the three time groups were pooled and data were tested using a t -test assuming unequal variance. We found significant differences between time groups for two parameters (area of myelinated axons in BF and volume fraction of cell nuclei in MN) with the analysis of variance (ANOVA) test. However, when the two nerves were compared for these two parameters for each time point there was a significant difference between nerves in all three time groups, and for simplicity the results are also shown with the nerves pooled for these parameters. Comparisons between crushed and uncrushed nerves were performed in the same way, where the time groups for the uncrushed nerves were pooled if the ANOVA test did not result in a significant difference between time groups. In order to test for potential differences in the degeneration and regeneration capacities of the MN and BF, we converted the absolute values to percentages, where the group mean values of the uncrushed reference nerves were defined as 100%. When comparing the numbers of specified axons, the numbers of myelinated axons in the reference nerves were defined as 100% because the normal nerves have no degenerating and newly formed axons. The percentages were compared with the t -test assuming unequal variance. A P -value of 5% or lower was considered significant.
Results
The comparison of the normal anatomy of the uncrushed control nerves is shown in Fig. 3 . We found significant differences in the normal anatomy of all examined parameters with the exception of the volume fraction of cell nuclei. The total number of myelinated axons was twice as high in the MN as in the BF. The total cross-sectional area of the nerve was approximately 50% higher in the MN than in the BF and all three quantified tissue components were significantly larger in the MN than in the BF. The volume fraction of cell nuclei (excluding Schwann cell nuclei) was small and comparable in the two nerves, but the volume fraction of myelinated axons including their myelin was highest in the MN, whereas the volume fraction of endoneurium was highest in the BF.
The effects of a crush lesion are summarized in Figs. 4 and 5 . The total numbers of specified axons are given in Fig. 4 and the cross-sectional areas of specified tissues in Fig. 5 . Normal myelinated axons were dominant at day 3 after the crush injury, but degenerating axons were prominent and demyelinated axons were also seen. At day 7 the normal myelinated axons were virtually absent and the dominant axons were the newly formed axons, although some degenerating axons could also be seen. At day 19 we could not distinguish between the regenerating axons which had a more mature morphology and the normal axons, although the regenerating axons were smaller in diameter with a thinner myelin sheath. However, since virtually no normal axons were observed at day 7, the majority of the so-called normal myelinated axons at day 19 must be regenerated myelinated axons. In the MN there was a trend towards a loss in the total number of myelinated axons ( P = 0.062) and a corresponding significant decrease in the cross-sectional area of myelinated axons at day 3, and both parameters were significantly decreased at day 7. A significant increase in the area of cell nuclei was observed both at days 3 and 7, and a significant increase in the cross-sectional area of intercellular tissue was seen at day 7. The two latter changes probably reflect an inflammatory oedema. The increase in the area of the intercellular tissue is cancelled out by the decrease in the area of the myelinated axons, so that the total area is not increased, i.e. no entrapment was observed in the MN. In the BF, the axon loss was significant at day 3, although it was smaller than the insignificant loss seen in the MN. The axon number was back to normal at day 7, but the area of myelinated axons was decreased, indicating that the axons are smaller than normal axons. At day 19 the axon number was insignificantly increased, but the axon area was still decreased. The area of cell nuclei was significantly increased at all time points and the endoneurial area was significantly increased at days 3 and 7. In the BF, where the volume fraction of endoneurium is larger than it is in the MN, the increase in the endoneurial tissue entailed a 46% increase in the total area of the BF at day 7.
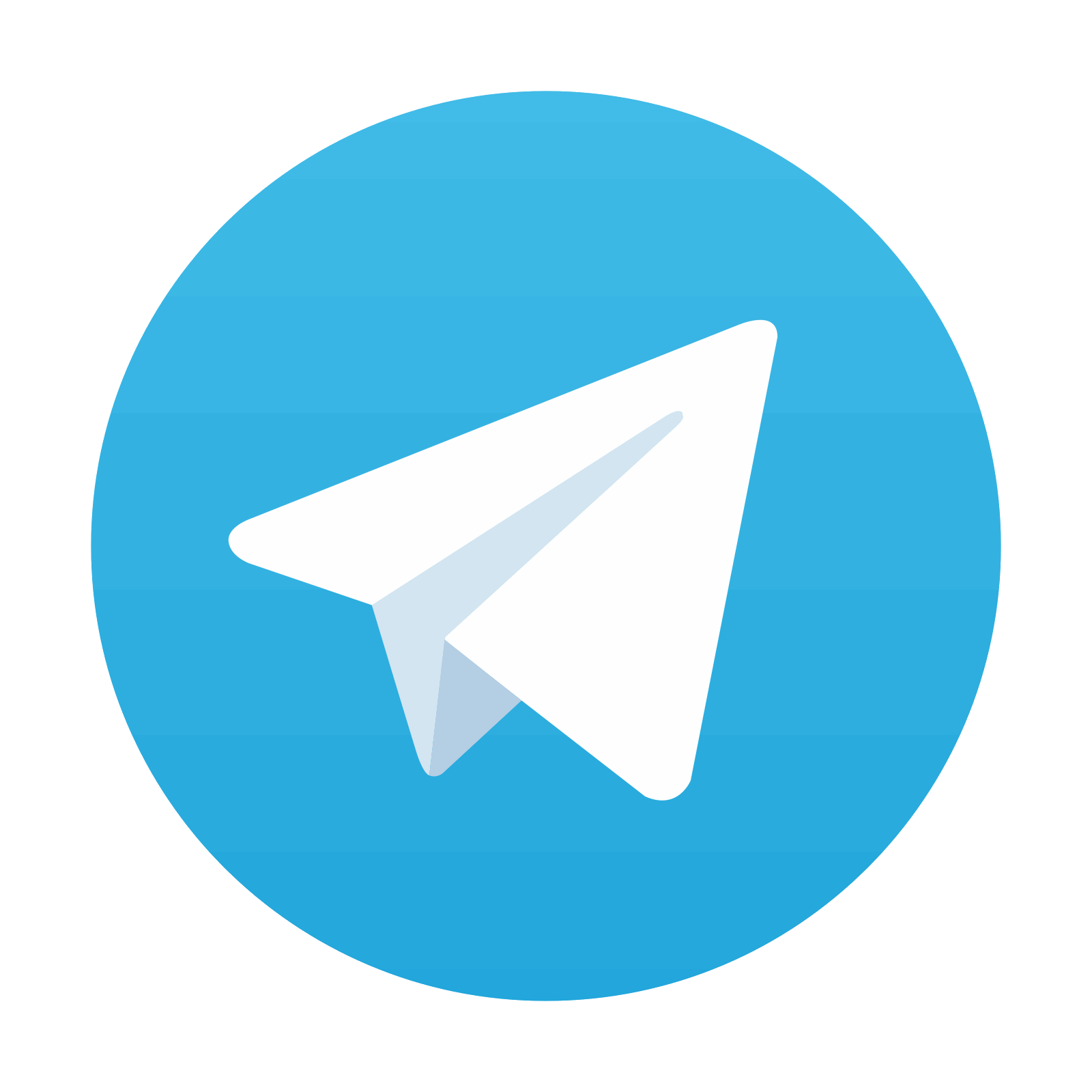
Stay updated, free dental videos. Join our Telegram channel

VIDEdental - Online dental courses
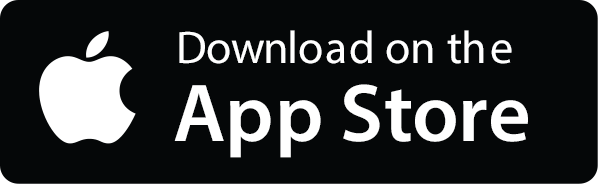
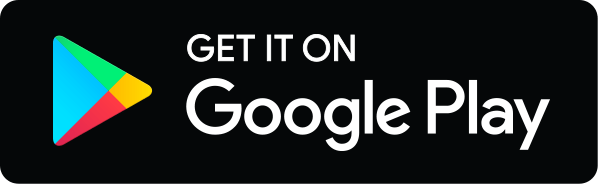
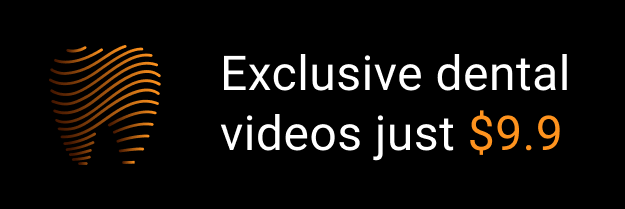