Abstract
Objective
To identify the minimum data acquisition variables in the x – and y -planes required when using three-dimensional (3D) profilometry to produce accurate mean total volumetric wear and mean maximum wear depth measurements for a range of wear facets produced by an oral wear simulator.
Methods
The Oregon Health Science University (OHSU) oral wear simulator was employed to wear an experimental resin-based composite formulation from 25,000 to 400,000 wear cycles. Mean total volumetric wear and mean maximum wear depth were determined using a contact profilometry at a measurement speed of 1 mm/s. An area (8 mm length and 4 mm width) was profiled on each wear facet comprising 8001 horizontal traces ( y -axis at 1 μm intervals) with 4001 measurement points ( x -axis at 1 μm intervals) resulting in 32,012,001 measurement points with a z -axis resolution of 40 nm. The minimum x – and y -axis spacing data acquisition requirement were assessed using the TalyMap software by reducing the number of measurement points in the original scanned wear facets and normalized data were converted to percentage values.
Results
Minimum x – and y -axis spacing to achieve an accuracy of 99, 95 and 90% of the mean total volumetric wear value for the wear facets produced (25,000–400,000 wear cycles) were 20 μm × 20 μm, 100 μm × 100 μm and 200 μm × 200 μm, respectively but for maximum wear depth data normalized with respect to the ‘true value or gold standard’ the x – and y -axis spacing requirement varied with the size of the wear facet.
Significance
The study emphasizes the difficulty in employing mean maximum wear depth measurements when assessing the in vitro wear facets produced by an OHSU oral wear simulator and accurate quantification of the mean total volumetric wear of a wear facet is a prerequisite to informing the profession about dental restorative wear performance.
1
Introduction
Since the first in vitro resin-based composite (RBC) wear studies were published in 1975 , there has been considerable interest in the wear performance of RBCs in the dental literature. A pubmed enquiry using the identifiable Medical Subject Headings of ‘dentistry AND wear AND resin composite’ resulted in an average of 40 manuscripts published annually (from 2008 onwards) and herein lies the problem. There are four fundamental wear mechanisms (abrasion, adhesion, fatigue or corrosion) which influence wear – ‘the ultimate consequence of interaction between surfaces which is manifested in gradual removal of material’ . However, within dentistry, we use terms to describe clinical manifestations of wear rather than fundamental wear mechanisms . These terms focus on surface loss at sites of occlusal contact (attrition), surface loss at non-contacting sites (abrasion) and surface loss attributed to chemical effects (erosion) . Further analysis of these 40 manuscripts (published annually from 2008 onwards) identified that the research is infrequently in the form of in vivo studies that compare different RBCs under clinical conditions namely, direct observation at regular time intervals or indirect assessment of tooth replicas over time . The major reasons for the lack of in vivo studies include the significant shortcomings of both the observation indices and tooth replica techniques. Direct observation requires the same clinical observer(s) for numerous patient visits, resulting in considerable time and expense in data collection Tooth replicas are difficult to interpret owing to the susceptibility of dental impression and stone materials to operator induced variability on mixing and given the RBC wear rates involved, the dimensional accuracy of the tooth replicas are questionable .
Consequently, researchers have focused on developing in vitro oral wear simulators in an effort to assess the wear resistance of restorative materials prior to placement in the mouth. While this approach was admirable and if successful could have averted the need for expensive and time consuming clinical trials, the masticatory cycle in vitro was replicated with varying degrees of success . Attempts to inform the dental community about the pitfalls of testing restorative materials in a variety of in vitro oral wear simulators have been in the form of round robin tests , validation of in vitro wear depth data produced in the dental literature and a correlation of the in vitro wear depth data from oral wear simulators with in vivo clinical data . In short, no single in vitro wear simulator can simulate the range of wear mechanisms operative in the oral environment and significant differences in wear depth measurements (often in the region of 70%) were highlighted for the same RBC material tested using the same in vitro wear simulator technique . Interestingly, a significant correlation in the in vitro wear depth data from the Oregon Health Science University (OHSU) oral wear simulator (Proto-tech, Portland, OR, USA) with in vivo clinical data was shown . However, it should be noted that the correlation was in part due to the ‘semi-quantitative methods for assessing the wear in vivo on pooled data from clinical trials’ . In addition, the employment of non-sophisticated two-dimensional (2D) scanning was sub-optimal as the reproducibility of the mean wear depth results from different test centers was ‘best characterized as inconclusive’ .
Wear volume rather than wear depth was considered to be a measure of the ‘work done’ due to the interaction of opposing surfaces , such that DeLong recommended the use of volumetric wear as the parameter of choice for reporting in vitro wear . Unfortunately, in vitro volumetric wear determination requires three-dimensional (3D) scanning equipment which has not been routinely employed in the dental literature .
When the 3D measurement technique was employed to assess the mean total volumetric wear of RBC materials , the data acquisition variables in the x – and y -planes (parallel and perpendicular, respectively) to the sliding direction of the wear simulator were randomly chosen varying from 4–5 μm ( x -plane) to 4–40 μm ( y -plane). However, the data acquisition variables in the x – and y -planes have not been examined to consider how they can influence the accuracy and precision of the in vitro measurements wear measurements (mean total volumetric wear and mean maximum wear depth) reported. The aim of the current study was therefore to identify the minimum data acquisition variables in the x – and y -planes required to produce accurate mean total volumetric wear and mean maximum wear depth measurements for a range of wear facets produced by the OHSU oral wear simulator.
2
Materials and methods
2.1
RBC material
The RBC restorative material tested was an experimental formulation based on an existing commercially successful RBC (Grandio, Voco GmbH, Cuxhaven, Germany). The experimental formulation contained camphorquinone as the photo-initiator and was produced using the same manufacturing processing route as Grandio by manipulating the monomeric resin composition and the barium-alumina borosilicate filler characteristics . The monomeric blend ratio of BisGMA:TEGDMA of Grandio was increased from 3:1 to 3.5:1 for the experimental RBC formulation while the mean filler diameter (2.5 μm) and filler volume fraction (73 vol%) were increased compared with Grandio (1.5 μm and 71.4 vol%, respectively).
2.2
Specimen preparation
The experimental RBC material was prepared into eight disc-shaped specimens (13.0 ± 0.1 mm diameter and 2.0 ± 0.1 mm thickness) using a nylon ring-mold which was placed on a glass slab covered with an acetate strip. The assembly was placed on a balance reading to 0.001 g (Sartorius Expert, Sartorius AG, Goettingen, Germany), the balance zeroed and a consistent mass (0.62 g) of uncured resin was syringed into the center of the ring-mold. A second acetate strip was placed on top and a 1 kg weight applied to dissipate the RBC evenly . Specimens were light irradiated from the top surface only for 20 s by placing a 13 mm light tip diameter in direct contact with the top acetate strip using a hand-held quartz-tungsten halogen (QTH) light curing unit (LCU) (Optilux 501, Kerr, Orange Co., CA, USA) operating at an output intensity of 560 ± 24 mW/cm 2 . Following irradiation, specimens were removed from the ring-molds and stored under light-proof conditions for 23 h in 50 mL of distilled water at 37 ± 1 °C (Firlabo SP BVEHF, Société Firlabo, Meyzieu, France) .
2.3
In vitro wear testing
The disc-shaped RBC specimens were mounted in a two part cold-setting acrylic resin (Varidur; Buehler, Lake Bluff, IL, USA), 60 min prior to wear testing. The specimens were centrally positioned in individual mounting cups (Metset Cups, Buehler), the acrylic resin mixed (powder to liquid mixing ratio of 1 g to 1 mL) and poured to a height of 10.0 ± 1 mm . After 45 min, the mounted specimens were removed and wet ground sequentially for 30 s each with P600 and P1200 silicon carbide abrasive paper (Buehler), under water lubrication on a grinding-polishing machine (Alpha and Beta Grinder-Polisher, Buehler) at a 10 N force.
Each OHSU chamber ( n = 4) were calibrated for abrasion and attrition forces prior to the commencement of each wear testing regime using a 500 N load cell custom jig (Proto-tech) . The mounted specimens were secured into the individual chambers of the OHSU oral wear simulator 24 h following irradiation and marked with a waterproof alcohol pen for future realignment . A spherical (10.0 ± 0.1 mm diameter) steatite antagonist (Union Process Inc., Akron, OH, USA) was forced into contact with the RBC specimens in the presence of a food-like slurry containing 5 mL of distilled water, 0.5 g of poly(methyl methacrylate) beads with a mean particle diameter of 50-100 μm (ProBase Cold Polymer; Ivoclar Vivadent, Schaan, Liechtenstein) and 1.0 g of crushed poppy seeds (Holland and Barrett, Burton-upon-Trent, England). The antagonist imparted a 20 N sliding force along a 7 mm sliding path (abrasion) and a 90 N impact force at the end of the 7 mm sliding path (attrition) before being returned automatically to the start of the sliding path and the wear cycle repeated at a frequency of 1 Hz for the predetermined number of wear cycles.
Following 25,000 wear cycles (Group A), the specimens were removed from the individual chambers of the OHSU oral wear simulator, rinsed under running water to remove remnants of the food-like slurry and patted with absorbent tissue until totally dry. The mean total volumetric wear and mean maximum wear depth were determined using a contact diamond stylus profilometer (Talysurf CLI 2000, Taylor-Hobson Precision, Leicester, England) containing a 90° conisphere stylus tip of 2 μm radius, at a measurement speed of 1 mm/s. An area of 8 mm length and 4 mm width was profiled on each wear facet comprising 8001 horizontal traces ( y -axis: perpendicular to the sliding direction at 1 μm intervals) with 4001 measurement points ( x -axis: parallel to the sliding direction at 1 μm intervals) recorded for each wear facet resulting in a total of 32,012,001 measurement points. The resolution in the z -axis was 40 nm for the scanning conditions investigated (measurement speed, x – and y -axis scanning intervals). Three-dimensional (3D) representations of the wear facets were generated using TalyMap Gold analysis software Version 4.2 (Taylor-Hobson Precision) where the non-worn areas around the wear facet were used as the reference plane and the mean total volumetric wear (mm 3 ) and mean maximum wear depth (mm) were determined. Immediately following profilometric analyses, the RBC specimens were replaced, aligned using the waterproof alcohol pen markings and secured into the individual wear chambers of the OHSU oral wear simulator. Fresh food-like slurry was added to each chamber and the specimens were subjected to a further 25,000 wear cycles (50,000 wear cycles in total: Group B) at 1 Hz. The wear facets were re-analyzed using the contact diamond stylus profilometric (procedure outlined above) and the mean total volumetric wear (mm 3 ) and mean maximum wear depth (mm) were determined from the non-worn areas around the wear facet used as the reference plane . The RBC specimens were then re-inserted into the oral wear simulator, a fresh food-like slurry added and the specimens subjected to a further 50,000 wear cycles (100,000 wear cycles in total: Group C) at 1 Hz. The wear facets were re-analyzed and the mean total volumetric wear (mm 3 ) and mean maximum wear depth (mm) were determined from the non-worn areas around the wear facet (as previously described ). The procedure was repeated at 100,000 wear cycle intervals (Group D: 200,000; Group E: 300,000; Group F: 400,000 wear cycles in total) to cover a range of wear facets comparable with the range of restorative materials employed clinically.
To determine the accuracy and precision of the mean total volumetric wear (mm 3 ) and mean maximum wear depth (mm) measurements, the Talysurf CLI 2000 contact diamond stylus profilometer (Taylor-Hobson Precision) was used at a speed of 1 mm/s. The accuracy and precision of the total volumetric wear measurements from the wear facets were determined by scanning a standard wear facet (7.405 mm 3 ) of known total volume (which was supplied with the profilometer as an internal standard) on ten consecutive occasions. A series of horizontal traces (perpendicular to the sliding direction of the OHSU oral wear simulator ( y -axis)) were conducted across the wear facet at 1 μm intervals with longitudinal measurements (parallel to the sliding direction of the OHSU oral wear simulator ( x -axis)) recorded every 1 μm to scan an area of 32 mm 3 (8v × 4 mm) around the wear facet. A three-dimensional image of each wear facet was created using the TalyMap Gold analysis software and the total volumetric wear (mm 3 ) measurements were quantified using the non-worn areas surrounding the wear facet as the reference plane . Additionally, ten traces were performed across a standard step height of 1.0 mm (supplied with the profilometer as an internal standard) to assess the accuracy and precision of the wear depth measurement using the TalyMap Gold analysis software Version 4.2 (Taylor-Hobson Precision). The accuracy of the mean total volumetric wear (mm 3 ) and mean maximum wear depth (mm) measurements were calculated as the mean error from the true value, whilst the precision of the wear measurements were quantified as the standard deviation of the errors measured .
To investigate the minimum x – and y -axis spacing requirement for the data acquisition variable in the x – and y -axes, the wear facets were re-analyzed using the TalyMap software. The x -axis spacing was increased from 1 μm to 2, 4, 8, 10, 20, 40, 80, 100, 200 and 400 μm by reducing the number of measurement points in the original wear facets scanned at a measurement speed of 1 mm/min from 4001 to 11 as illustrated in Table 1 to maintain a consistent z -axis resolution. Similarly, the y -axis spacing was increased from 1 μm to 2, 4, 8, 10, 20, 40, 80, 100, 200, 400 and 800 μm by re-analysing the wear facets using the TalyMap software to effectively reduce the number of horizontal measurement points on each trace from 8001 to 11 ( Table 1 ). The z -axis resolution was maintained at 40 nm owing to the data acquisition measurement speed of 1 mm/min remaining constant throughout. The mean total volumetric wear (mm 3 ) and mean maximum wear depth (mm) of each wear facet were re-calculated at the increased x – and y -axis spacing using the TalyMap software. The mean total volumetric wear and mean maximum wear depth for each wear facet was calculated at the increased x – and y -axis spacing were normalized with respect to the mean total volumetric wear and mean maximum wear depth determined at an x – and y -axis spacing of 1 μm × 1 μm (“the gold standard”). The normalized data were converted to percentage values to show the change in mean total volumetric wear and mean maximum wear depth with increasing x – and y -axes spacing. For each wear regime under investigation (25,000 (Group A); 50,000 (Group B), 100,000 (Group C); 200,000 (Group D), 300,000 (Group E) and 400,000 (Group F) wear cycles) the percentage values for the total volumetric wear and maximum wear depth data of the eight wear facets were pooled and the mean, standard deviation and coefficient of variation were determined.
x -Axis spacing (μm) | Number of measurement points | y -Axis spacing (μm) | Number of profilometric traces | Total number of measurement points |
---|---|---|---|---|
1 | 4001 | 1 | 8001 | 32,012,001 |
2 | 2001 | 2 | 4001 | 8,006,001 |
4 | 1001 | 4 | 2001 | 200,3001 |
8 | 501 | 8 | 1001 | 501,501 |
10 | 401 | 10 | 801 | 321,201 |
20 | 201 | 20 | 401 | 80,601 |
40 | 101 | 40 | 201 | 20,301 |
80 | 51 | 80 | 101 | 5151 |
100 | 41 | 100 | 81 | 3321 |
200 | 21 | 200 | 41 | 861 |
400 | 11 | 400 | 21 | 231 |
800 | 11 |
2.4
Statistical analysis
The normality of the data in each group was assessed using the Shapiro-Wilk test ( p < 0.05). The statistical analyses included paired comparisons using twelve Independent Student’s t-tests (for each total number of wear cycles examined (Group A-F)) for the mean total volumetric wear ( n = 6) and mean maximum wear depth data ( n = 6)) at a significance value of p = 0.05.
2
Materials and methods
2.1
RBC material
The RBC restorative material tested was an experimental formulation based on an existing commercially successful RBC (Grandio, Voco GmbH, Cuxhaven, Germany). The experimental formulation contained camphorquinone as the photo-initiator and was produced using the same manufacturing processing route as Grandio by manipulating the monomeric resin composition and the barium-alumina borosilicate filler characteristics . The monomeric blend ratio of BisGMA:TEGDMA of Grandio was increased from 3:1 to 3.5:1 for the experimental RBC formulation while the mean filler diameter (2.5 μm) and filler volume fraction (73 vol%) were increased compared with Grandio (1.5 μm and 71.4 vol%, respectively).
2.2
Specimen preparation
The experimental RBC material was prepared into eight disc-shaped specimens (13.0 ± 0.1 mm diameter and 2.0 ± 0.1 mm thickness) using a nylon ring-mold which was placed on a glass slab covered with an acetate strip. The assembly was placed on a balance reading to 0.001 g (Sartorius Expert, Sartorius AG, Goettingen, Germany), the balance zeroed and a consistent mass (0.62 g) of uncured resin was syringed into the center of the ring-mold. A second acetate strip was placed on top and a 1 kg weight applied to dissipate the RBC evenly . Specimens were light irradiated from the top surface only for 20 s by placing a 13 mm light tip diameter in direct contact with the top acetate strip using a hand-held quartz-tungsten halogen (QTH) light curing unit (LCU) (Optilux 501, Kerr, Orange Co., CA, USA) operating at an output intensity of 560 ± 24 mW/cm 2 . Following irradiation, specimens were removed from the ring-molds and stored under light-proof conditions for 23 h in 50 mL of distilled water at 37 ± 1 °C (Firlabo SP BVEHF, Société Firlabo, Meyzieu, France) .
2.3
In vitro wear testing
The disc-shaped RBC specimens were mounted in a two part cold-setting acrylic resin (Varidur; Buehler, Lake Bluff, IL, USA), 60 min prior to wear testing. The specimens were centrally positioned in individual mounting cups (Metset Cups, Buehler), the acrylic resin mixed (powder to liquid mixing ratio of 1 g to 1 mL) and poured to a height of 10.0 ± 1 mm . After 45 min, the mounted specimens were removed and wet ground sequentially for 30 s each with P600 and P1200 silicon carbide abrasive paper (Buehler), under water lubrication on a grinding-polishing machine (Alpha and Beta Grinder-Polisher, Buehler) at a 10 N force.
Each OHSU chamber ( n = 4) were calibrated for abrasion and attrition forces prior to the commencement of each wear testing regime using a 500 N load cell custom jig (Proto-tech) . The mounted specimens were secured into the individual chambers of the OHSU oral wear simulator 24 h following irradiation and marked with a waterproof alcohol pen for future realignment . A spherical (10.0 ± 0.1 mm diameter) steatite antagonist (Union Process Inc., Akron, OH, USA) was forced into contact with the RBC specimens in the presence of a food-like slurry containing 5 mL of distilled water, 0.5 g of poly(methyl methacrylate) beads with a mean particle diameter of 50-100 μm (ProBase Cold Polymer; Ivoclar Vivadent, Schaan, Liechtenstein) and 1.0 g of crushed poppy seeds (Holland and Barrett, Burton-upon-Trent, England). The antagonist imparted a 20 N sliding force along a 7 mm sliding path (abrasion) and a 90 N impact force at the end of the 7 mm sliding path (attrition) before being returned automatically to the start of the sliding path and the wear cycle repeated at a frequency of 1 Hz for the predetermined number of wear cycles.
Following 25,000 wear cycles (Group A), the specimens were removed from the individual chambers of the OHSU oral wear simulator, rinsed under running water to remove remnants of the food-like slurry and patted with absorbent tissue until totally dry. The mean total volumetric wear and mean maximum wear depth were determined using a contact diamond stylus profilometer (Talysurf CLI 2000, Taylor-Hobson Precision, Leicester, England) containing a 90° conisphere stylus tip of 2 μm radius, at a measurement speed of 1 mm/s. An area of 8 mm length and 4 mm width was profiled on each wear facet comprising 8001 horizontal traces ( y -axis: perpendicular to the sliding direction at 1 μm intervals) with 4001 measurement points ( x -axis: parallel to the sliding direction at 1 μm intervals) recorded for each wear facet resulting in a total of 32,012,001 measurement points. The resolution in the z -axis was 40 nm for the scanning conditions investigated (measurement speed, x – and y -axis scanning intervals). Three-dimensional (3D) representations of the wear facets were generated using TalyMap Gold analysis software Version 4.2 (Taylor-Hobson Precision) where the non-worn areas around the wear facet were used as the reference plane and the mean total volumetric wear (mm 3 ) and mean maximum wear depth (mm) were determined. Immediately following profilometric analyses, the RBC specimens were replaced, aligned using the waterproof alcohol pen markings and secured into the individual wear chambers of the OHSU oral wear simulator. Fresh food-like slurry was added to each chamber and the specimens were subjected to a further 25,000 wear cycles (50,000 wear cycles in total: Group B) at 1 Hz. The wear facets were re-analyzed using the contact diamond stylus profilometric (procedure outlined above) and the mean total volumetric wear (mm 3 ) and mean maximum wear depth (mm) were determined from the non-worn areas around the wear facet used as the reference plane . The RBC specimens were then re-inserted into the oral wear simulator, a fresh food-like slurry added and the specimens subjected to a further 50,000 wear cycles (100,000 wear cycles in total: Group C) at 1 Hz. The wear facets were re-analyzed and the mean total volumetric wear (mm 3 ) and mean maximum wear depth (mm) were determined from the non-worn areas around the wear facet (as previously described ). The procedure was repeated at 100,000 wear cycle intervals (Group D: 200,000; Group E: 300,000; Group F: 400,000 wear cycles in total) to cover a range of wear facets comparable with the range of restorative materials employed clinically.
To determine the accuracy and precision of the mean total volumetric wear (mm 3 ) and mean maximum wear depth (mm) measurements, the Talysurf CLI 2000 contact diamond stylus profilometer (Taylor-Hobson Precision) was used at a speed of 1 mm/s. The accuracy and precision of the total volumetric wear measurements from the wear facets were determined by scanning a standard wear facet (7.405 mm 3 ) of known total volume (which was supplied with the profilometer as an internal standard) on ten consecutive occasions. A series of horizontal traces (perpendicular to the sliding direction of the OHSU oral wear simulator ( y -axis)) were conducted across the wear facet at 1 μm intervals with longitudinal measurements (parallel to the sliding direction of the OHSU oral wear simulator ( x -axis)) recorded every 1 μm to scan an area of 32 mm 3 (8v × 4 mm) around the wear facet. A three-dimensional image of each wear facet was created using the TalyMap Gold analysis software and the total volumetric wear (mm 3 ) measurements were quantified using the non-worn areas surrounding the wear facet as the reference plane . Additionally, ten traces were performed across a standard step height of 1.0 mm (supplied with the profilometer as an internal standard) to assess the accuracy and precision of the wear depth measurement using the TalyMap Gold analysis software Version 4.2 (Taylor-Hobson Precision). The accuracy of the mean total volumetric wear (mm 3 ) and mean maximum wear depth (mm) measurements were calculated as the mean error from the true value, whilst the precision of the wear measurements were quantified as the standard deviation of the errors measured .
To investigate the minimum x – and y -axis spacing requirement for the data acquisition variable in the x – and y -axes, the wear facets were re-analyzed using the TalyMap software. The x -axis spacing was increased from 1 μm to 2, 4, 8, 10, 20, 40, 80, 100, 200 and 400 μm by reducing the number of measurement points in the original wear facets scanned at a measurement speed of 1 mm/min from 4001 to 11 as illustrated in Table 1 to maintain a consistent z -axis resolution. Similarly, the y -axis spacing was increased from 1 μm to 2, 4, 8, 10, 20, 40, 80, 100, 200, 400 and 800 μm by re-analysing the wear facets using the TalyMap software to effectively reduce the number of horizontal measurement points on each trace from 8001 to 11 ( Table 1 ). The z -axis resolution was maintained at 40 nm owing to the data acquisition measurement speed of 1 mm/min remaining constant throughout. The mean total volumetric wear (mm 3 ) and mean maximum wear depth (mm) of each wear facet were re-calculated at the increased x – and y -axis spacing using the TalyMap software. The mean total volumetric wear and mean maximum wear depth for each wear facet was calculated at the increased x – and y -axis spacing were normalized with respect to the mean total volumetric wear and mean maximum wear depth determined at an x – and y -axis spacing of 1 μm × 1 μm (“the gold standard”). The normalized data were converted to percentage values to show the change in mean total volumetric wear and mean maximum wear depth with increasing x – and y -axes spacing. For each wear regime under investigation (25,000 (Group A); 50,000 (Group B), 100,000 (Group C); 200,000 (Group D), 300,000 (Group E) and 400,000 (Group F) wear cycles) the percentage values for the total volumetric wear and maximum wear depth data of the eight wear facets were pooled and the mean, standard deviation and coefficient of variation were determined.
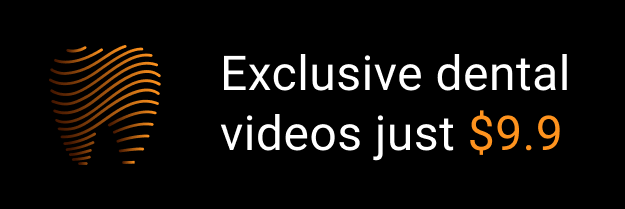