Highlights
- •
The results show that the composites, independent of composition, had equivalent potency in terms of reducing the viability of the cells in culture.
- •
Soaking the composites for 7 days before exposing them to the cells suggested that the “toxic” components had been extracted and the materials were no longer cytotoxic.
- •
The results demonstrate that the cytotoxicity of composites with and without BAG must predominantly be attributed to the release of residual monomers, and not to the presence of the BAG.
Abstract
Objective
To determine the in vitro cytotoxicity of dental composites containing bioactive glass fillers.
Methods
Dental composites (50:50 Bis-GMA/TEGDMA resin: 72.5 wt% filler, 67.5%Sr–glass and 5% OX50) containing different concentrations (0, 5, 10 and 15 wt%) of two sol–gel bioactive glasses, BAG65 (65 mole% SiO 2 , 31 mole% CaO, 4 mole% P 2 O 5 ) and BAG61 (3 mole% F added) were evaluated for cytotoxicity using Alamar Blue assay. First, composite extracts were obtained from 7 day incubations of composites in cell culture medium at 37 °C. Undifferentiated pulp cells (OD-21) were exposed to dilutions of the original extracts for 3, 5, and 7 days. Then freshly cured composite disks were incubated with OD-21 cells ( n = 5) for 2 days. Subsequently, fresh composite disks were incubated in culture medium at 37 °C for 7 days, and then the extracted disks were incubated with OD-21 cells for 2 days. Finally, fresh composites disks were light cured for 3, 5, and 20 s and incubated with OD-21 cells ( n = 5) for 1, 3, 5, and 7 days. To verify that the three different curing modes produced different levels of degree of conversion (DC), the DC of each composite was determined by FTIR. Groups ( n = 5) were compared with ANOVA/Tukey’s ( α ≤ 0.05).
Results
Extracts from all composites significantly reduced cell viability until a dilution of 1:8 or lower, where the extract became equal to the control. All freshly-cured composites showed significantly reduced cell viability at two days. However, no reduction in cell viability was observed for any composite that had been previously soaked in media before exposure to the cells. Composites with reduced DC (3 s vs. 20 s cure), as verified by FTIR, showed significantly reduced cell viability.
Significance
The results show that the composites, independent of composition, had equivalent potency in terms of reducing the viability of the cells in culture. Soaking the composites for 7 days before exposing them to the cells suggested that the “toxic” components had been extracted and the materials were no longer cytotoxic. The results demonstrate that the cytotoxicity of composites with and without BAG must predominantly be attributed to the release of residual monomers, and not to the presence of the BAG.
1
Introduction
The cytotoxicity of dental composite materials has been attributed to the release of residual monomers, a result of an incomplete polymerization reaction, or to the by-products of resin matrix degradation processes . It has been shown that well cured resin composites are less cytotoxic than those with lower degree of conversion . In fact, the elimination of leachable components from polymerized composites by the use of organic solvents completely eliminated the cytotoxicity of certain composites in one study .
The principal monomers used in dental resin composites are bisphenol A-glycidylmethacrylate (Bis-GMA), triethyleneglycol dimethacrylate (TEGDMA), and urethane dimethacrylate (UDMA), all of which have been identified as cytotoxic molecules . It has also been shown that TEGDMA may be a mutagenic compound in mammalian cells. The material induced gene mutations probably because of the covalent binding to DNA via Michael addition . Based on these concerns, considerable effort is being expended to develop alternative monomer systems which may have fewer negative biological consequences.
Additionally, new materials are being formulated with additives that may provide a positive biological effect, such as antimicrobial characteristics, or tooth remineralizing potential. Adhesives containing silver nanoparticles have been shown to significantly decrease bacteria compared to the adhesive alone . Quaternary ammonium compounds (QACs) are a group of cationic antimicrobials which exhibit stable and long-term antibacterial effects. Imazato et al. combined a QAC, with a methacrylate group to synthesize a novel dental resin monomer called MDPB (12-methacryloyloxydodecylpyridinium bromide). MDPB-containing resins can inhibit the growth of bacteria, such as Streptococcus mutans . In other work, it has been shown that adhesives containing S-PRG (pre-reacted glass ionomer) filler decreased demineralization of tooth structure . Weir et al. found that NACP (Amorphous calcium phosphate nanoparticles) containing nanocomposites could effectively remineralize human enamel by releasing Ca and PO 4 and increasing the pH . While there is excitement over the potential for these potentially “bioactive” materials, there is a need to assess their biocompatibility.
Bioactive glasses (BAG) are another type of biologically active compound that have been used as a constituent of materials designed for regeneration of bone , orthopedic bone cements , dental restorative materials , dental cements pit and fissure sealants , and also for treatment of hypersensitive teeth . The potential remineralizing effect of these materials, owing to the release of calcium and phosphate ions, has attracted much attention. The development of sol–gel processed glasses for these purposes is particularly interesting due to the high surface area attainable with the particulate material, which enhances ion release . These glasses are produced with higher silica contents than typical melt derived glasses and do not contain sodium, but remain highly reactive because the processing method results in a nanoporous glass structure that increases their surface area by orders of magnitude over typical melt derived glasses. These glasses may also have antibacterial characteristics that would be beneficial when used in dental restorations where they may be placed very close to vital pulp tissues of teeth previously infected by bacteria . Recently, Hu et al. showed that bioactive glass 45S5 had a strong antibacterial effect against both gram-positive and gram-negative bacteria, though the sensitivity of the two different bacteria varied . Waltimo et al. found that a nanometric bioactive glass 45S5 released more alkaline species due to a larger surface area, and subsequently showed a higher antimicrobial effect than melt-derived, micron-sized 45S5 bioactive glass .
The objective of this study was to determine the in vitro cytocompatibility of dental resin composites containing sol–gel derived bioactive glass fillers using undifferentiated dental pulp cells (OD-21).
The hypothesis to be tested was that any cytotoxicity of the composites would be attributable to the residual monomer in the cured materials, and not to the ions leached from the bioactive glass additive. To accomplish this, composites were directly exposed to cells immediately after being light cured to various extents, as well as after extraction of residual monomer in media. In addition, cells were exposed directly to the extracts from the variably cured composites.
2
Materials and methods
2.1
Preparation of experimental resin composites
Experimental resins were prepared with a mix of 50 wt% BisGMA (bisphenol A-glycidyl-dimethacrylate) and 50 wt% TEGDMA (triethylene glycol-dimethacrylate), both of which were graciously donated by ESSTECH Technology Inc., (Essington, PA, USA). To this mixture, 0.4 wt% of Camphorquinone and 0.8 wt% of EdMAB (Ethyl 4-dimethyl aminobenzoate) were added as photoinitiator and accelerator, respectively (purchased from Sigma-Aldrich Inc., St. Louis, MO, USA). Chemicals were used as provided by the manufacturers.
Control dental composites were prepared by mixing the resin with 72.5 wt% of filler comprised of 5% silane-treated OX50 and 67.5% of a silane-treated strontium glass of approximately 2–3 μm average size (graciously supplied by Bisco, Inc., Schaumburg, IL, USA) in a mechanical mixer (SpeedMixer DAC 150 FVZ, Flacktek, Landrum, SC, USA) at 2300 rpm for 2 min. Experimental composites were made from the same resin and strontium glass filler, but without the OX50 and with the substitution of the strontium glass with 0, 5, 10 and 15 wt% of two sol–gel processed bioactive glasses: BAG65 (65 mole% SiO 2 , 31 mole% CaO, 4 mole% P 2 O 5 ) and BAG61 (3 mole% F added with commensurate reduction of CaO). The BAG was synthesized as described previously . Thus, seven composite formulations were produced, six containing bioactive glass (three of which contained fluoride) and one control without BAG.
2.2
Specimen and extract preparation
2.2.1
Composite extract group—Production of composite extracts
Disk-shaped specimens ( D = 5 mm, 2 mm thick) were made by packing uncured composite into a polyvinylsiloxane mold, sandwiched between two glass slides and clamped with a paper clip for 20 s to extrude the excess material. The specimens were then light cured using a dental curing unit (Demi, Kerr, Danbury, CT, USA) with a 11 mm diameter light tip and measured exitance irradiance of approximately 600 mW/cm 2 (PM1 Laser Power Meter, Molectron, Portland, OR, USA). The materials were light-cured for 20 s (∼12 J/cm 2 ) from the top surface. All samples were stored dry for 24 h and then the top surface was finished with sequential SiC sandpapers (600, 1200, 4000 grit) under water cooling. All the specimens were sterilized by UV radiations for 15 min on each side in a cell culture hood. Extracts of these samples were prepared following ISO 10993-12. Extracts were prepared from 15 samples (each of 70.68 mm 2 surface area) in 5 ml cell culture medium. After a 7 day soaking period, these original extracts were then serially diluted in cell culture medium as follows: 1:1, 1:2, 1:4, 1:8, 1:16, and 1:32 . Five replicate cell cultures were exposed to serial dilutions (at least six dilutions down to 1:32) of the original extracts of the materials.
2.2.2
Fresh composite-direct extract group—Production of fresh-cured composites for direct exposure to cells
Disk-shaped specimens ( D = 9 mm, 2 mm thick, n = 5 per composite/BAG formulation) were made as described in Composite extract group, and exposed directly to cultured cells as described below.
2.2.3
Previously extracted composite-direct extract group—Production of previously extracted composites for direct exposure to cells
Disk-shaped specimens ( D = 9 mm, 2 mm thick, n = 5 per composite/BAG formulation) were made as described in Composite extract group and subjected to the same extraction protocol before the composite disks were then exposed directly to cultured cells as described below.
2.2.4
Reduced cure composite group—Production of composites with reduced radiant exposure for curing
Three different light curing modes were used to produce different levels of degree of conversion (DC). Specimens with and without BAG 65 and BAG 61 were prepared as in Composite extract group. The DC was evaluated by near-FTIR spectrometry (Nicolet 6700 FTIR, Thermo Scientific, Madison, WI, USA). The uncured composites were placed in silicone rubber molds ( n = 5; D = 5 mm; 2 mm thick) sandwiched between glass slides separated by spacers to collect the FTIR spectra (32 scans per spectrum, 4 cm −1 resolution; KBr beam splitter; InGaAs detector). The specimens were then light-cured with three different curing protocols: 360 mW/cm 2 for 3 s (1 J); 360 mW/cm 2 for 5 s (1.8 J) and 600 mW/cm 2 for 20 s (12 J). The lower irradiance (360 mW/cm 2 ) was obtained by placing a 0.2 absorptive neutral density filter (Thorlabs Inc, Newton, NJ) inside the light curing unit. Another spectrum of all the specimens was obtained after 24 h of storage in dry conditions. The peak area corresponding to vinyl stretching (6165 cm −1 ) was used before and after curing to calculate DC as follows: DC = [1 − (area of peak cured/area of peak uncured)] × 100%. After determining the conversion, the specimens were weighed prior to immersion in 8 ml of methylene chloride containing 0.01 wt% BHT (2,6-di- tert -butyl-4-methylphenol, Sigma Aldrich) for 1 week. The use of BHT is intended to avoid further conversion due to the increased molecular mobility produced in solvent-swollen networks. The solvent from the extraction solutions was removed under rotary evaporation to constant mass and 1 H NMR (600 MHz, Varian, Palo Alto, CA) spectra were obtained from the extracts to determine the composition on the sol fraction, according to previously described methods . The remaining discs were dried to a constant mass and re-weighed after extraction to determine mass loss (%).
2.3
Cell culture experiments
Undifferentiated dental pulp cells, OD-21, were maintained in Dulbecco’s modified Eagle’s medium (DMEM from Lonza Walkersville, MD, USA), containing 4.5 g/l glucose, 10% fetal bovine serum (FBS), and supplemented with an antibiotic solution (100 U/ml penicillin-G and 100 μg/ml streptomycin) and 0.594 g/l l -glutamine (Acros Organics, New Jersey, USA) at 37 °C in a humidified atmosphere of 5% CO 2 in air. These cells, graciously supplied by Dr. Tania Botero of the University of Michigan, were chosen due to their relevance to restorative dentistry. Several studies have shown that the use of resins as restorative materials is associated with irritation and necrosis of the pulp tissue. In addition, undifferentiated dental pulp cells might differentiate into odontoblasts to replace the cells that are destroyed by caries or dental restorative procedures .
To study the effect of the extracts from the BAG65 composites (Composite extract group) on cell viability, the OD-21 cells were cultured in 24-well plates, seeded at 5 K cells/well . After 24 h, the cells were treated with serial dilutions of each of the extracts and allowed to incubate in humidified 5% CO 2 and air at 37 °C for up to 7 days, with media exchanges containing the appropriate extract every other day. After 3, 5 and 7 days, a 1 ml solution of 10% Alamar Blue (Biosource, Camarillo, CA, USA) in DMEM was added to each well. Since Alamar Blue is a non-toxic aqueous dye, it is possible to assess cell viability over several time points with the same culture . After 1 h of incubation, 200 μl of each medium was placed into black 96-well plates in triplicate, and fluorescence intensity was measured using a plate reader (Fluoroskan Ascent FL Labsystems) with excitation at 530 nm and emission at 580 nm.
To study the effect of the BAG65 composites when directly exposed to the cells, OD-21 cells were directly cultured with freshly cured composite (fresh composite—direct extract group) as well as composite that had been preincubated in media to extract residual monomers (previously extracted composite—direct extract group) in 24-well plates at a seeding of 10 K cells/well. The composites were introduced after the cells were allowed to grow for 24 h, and then the composite/cell combination was allowed to incubate for 2 days.
To study the effect of total light exposure of BAG61 and BAG 65 composites on cell viability, the OD-21 cells ( n = 5) were cultured in 96-well plates, seeded at 5 K cells/well. After 24 h, the disks from the reduced cure composite group were placed in the wells containing the cells and incubated for 1, 3, 5, and 7 days. The cytotoxicity was evaluated by Alamar blue assay ( n = 3).
2.4
Statistical analysis
Cell viability data were analyzed by one-way analysis of variance (ANOVA) followed by Tukey’s multiple comparison test ( α ≤ 0.05). DC and solubility results were analyzed by two-way analysis of variance followed by Tukey’s test ( α ≤ 0.05).
2
Materials and methods
2.1
Preparation of experimental resin composites
Experimental resins were prepared with a mix of 50 wt% BisGMA (bisphenol A-glycidyl-dimethacrylate) and 50 wt% TEGDMA (triethylene glycol-dimethacrylate), both of which were graciously donated by ESSTECH Technology Inc., (Essington, PA, USA). To this mixture, 0.4 wt% of Camphorquinone and 0.8 wt% of EdMAB (Ethyl 4-dimethyl aminobenzoate) were added as photoinitiator and accelerator, respectively (purchased from Sigma-Aldrich Inc., St. Louis, MO, USA). Chemicals were used as provided by the manufacturers.
Control dental composites were prepared by mixing the resin with 72.5 wt% of filler comprised of 5% silane-treated OX50 and 67.5% of a silane-treated strontium glass of approximately 2–3 μm average size (graciously supplied by Bisco, Inc., Schaumburg, IL, USA) in a mechanical mixer (SpeedMixer DAC 150 FVZ, Flacktek, Landrum, SC, USA) at 2300 rpm for 2 min. Experimental composites were made from the same resin and strontium glass filler, but without the OX50 and with the substitution of the strontium glass with 0, 5, 10 and 15 wt% of two sol–gel processed bioactive glasses: BAG65 (65 mole% SiO 2 , 31 mole% CaO, 4 mole% P 2 O 5 ) and BAG61 (3 mole% F added with commensurate reduction of CaO). The BAG was synthesized as described previously . Thus, seven composite formulations were produced, six containing bioactive glass (three of which contained fluoride) and one control without BAG.
2.2
Specimen and extract preparation
2.2.1
Composite extract group—Production of composite extracts
Disk-shaped specimens ( D = 5 mm, 2 mm thick) were made by packing uncured composite into a polyvinylsiloxane mold, sandwiched between two glass slides and clamped with a paper clip for 20 s to extrude the excess material. The specimens were then light cured using a dental curing unit (Demi, Kerr, Danbury, CT, USA) with a 11 mm diameter light tip and measured exitance irradiance of approximately 600 mW/cm 2 (PM1 Laser Power Meter, Molectron, Portland, OR, USA). The materials were light-cured for 20 s (∼12 J/cm 2 ) from the top surface. All samples were stored dry for 24 h and then the top surface was finished with sequential SiC sandpapers (600, 1200, 4000 grit) under water cooling. All the specimens were sterilized by UV radiations for 15 min on each side in a cell culture hood. Extracts of these samples were prepared following ISO 10993-12. Extracts were prepared from 15 samples (each of 70.68 mm 2 surface area) in 5 ml cell culture medium. After a 7 day soaking period, these original extracts were then serially diluted in cell culture medium as follows: 1:1, 1:2, 1:4, 1:8, 1:16, and 1:32 . Five replicate cell cultures were exposed to serial dilutions (at least six dilutions down to 1:32) of the original extracts of the materials.
2.2.2
Fresh composite-direct extract group—Production of fresh-cured composites for direct exposure to cells
Disk-shaped specimens ( D = 9 mm, 2 mm thick, n = 5 per composite/BAG formulation) were made as described in Composite extract group, and exposed directly to cultured cells as described below.
2.2.3
Previously extracted composite-direct extract group—Production of previously extracted composites for direct exposure to cells
Disk-shaped specimens ( D = 9 mm, 2 mm thick, n = 5 per composite/BAG formulation) were made as described in Composite extract group and subjected to the same extraction protocol before the composite disks were then exposed directly to cultured cells as described below.
2.2.4
Reduced cure composite group—Production of composites with reduced radiant exposure for curing
Three different light curing modes were used to produce different levels of degree of conversion (DC). Specimens with and without BAG 65 and BAG 61 were prepared as in Composite extract group. The DC was evaluated by near-FTIR spectrometry (Nicolet 6700 FTIR, Thermo Scientific, Madison, WI, USA). The uncured composites were placed in silicone rubber molds ( n = 5; D = 5 mm; 2 mm thick) sandwiched between glass slides separated by spacers to collect the FTIR spectra (32 scans per spectrum, 4 cm −1 resolution; KBr beam splitter; InGaAs detector). The specimens were then light-cured with three different curing protocols: 360 mW/cm 2 for 3 s (1 J); 360 mW/cm 2 for 5 s (1.8 J) and 600 mW/cm 2 for 20 s (12 J). The lower irradiance (360 mW/cm 2 ) was obtained by placing a 0.2 absorptive neutral density filter (Thorlabs Inc, Newton, NJ) inside the light curing unit. Another spectrum of all the specimens was obtained after 24 h of storage in dry conditions. The peak area corresponding to vinyl stretching (6165 cm −1 ) was used before and after curing to calculate DC as follows: DC = [1 − (area of peak cured/area of peak uncured)] × 100%. After determining the conversion, the specimens were weighed prior to immersion in 8 ml of methylene chloride containing 0.01 wt% BHT (2,6-di- tert -butyl-4-methylphenol, Sigma Aldrich) for 1 week. The use of BHT is intended to avoid further conversion due to the increased molecular mobility produced in solvent-swollen networks. The solvent from the extraction solutions was removed under rotary evaporation to constant mass and 1 H NMR (600 MHz, Varian, Palo Alto, CA) spectra were obtained from the extracts to determine the composition on the sol fraction, according to previously described methods . The remaining discs were dried to a constant mass and re-weighed after extraction to determine mass loss (%).
2.3
Cell culture experiments
Undifferentiated dental pulp cells, OD-21, were maintained in Dulbecco’s modified Eagle’s medium (DMEM from Lonza Walkersville, MD, USA), containing 4.5 g/l glucose, 10% fetal bovine serum (FBS), and supplemented with an antibiotic solution (100 U/ml penicillin-G and 100 μg/ml streptomycin) and 0.594 g/l l -glutamine (Acros Organics, New Jersey, USA) at 37 °C in a humidified atmosphere of 5% CO 2 in air. These cells, graciously supplied by Dr. Tania Botero of the University of Michigan, were chosen due to their relevance to restorative dentistry. Several studies have shown that the use of resins as restorative materials is associated with irritation and necrosis of the pulp tissue. In addition, undifferentiated dental pulp cells might differentiate into odontoblasts to replace the cells that are destroyed by caries or dental restorative procedures .
To study the effect of the extracts from the BAG65 composites (Composite extract group) on cell viability, the OD-21 cells were cultured in 24-well plates, seeded at 5 K cells/well . After 24 h, the cells were treated with serial dilutions of each of the extracts and allowed to incubate in humidified 5% CO 2 and air at 37 °C for up to 7 days, with media exchanges containing the appropriate extract every other day. After 3, 5 and 7 days, a 1 ml solution of 10% Alamar Blue (Biosource, Camarillo, CA, USA) in DMEM was added to each well. Since Alamar Blue is a non-toxic aqueous dye, it is possible to assess cell viability over several time points with the same culture . After 1 h of incubation, 200 μl of each medium was placed into black 96-well plates in triplicate, and fluorescence intensity was measured using a plate reader (Fluoroskan Ascent FL Labsystems) with excitation at 530 nm and emission at 580 nm.
To study the effect of the BAG65 composites when directly exposed to the cells, OD-21 cells were directly cultured with freshly cured composite (fresh composite—direct extract group) as well as composite that had been preincubated in media to extract residual monomers (previously extracted composite—direct extract group) in 24-well plates at a seeding of 10 K cells/well. The composites were introduced after the cells were allowed to grow for 24 h, and then the composite/cell combination was allowed to incubate for 2 days.
To study the effect of total light exposure of BAG61 and BAG 65 composites on cell viability, the OD-21 cells ( n = 5) were cultured in 96-well plates, seeded at 5 K cells/well. After 24 h, the disks from the reduced cure composite group were placed in the wells containing the cells and incubated for 1, 3, 5, and 7 days. The cytotoxicity was evaluated by Alamar blue assay ( n = 3).
2.4
Statistical analysis
Cell viability data were analyzed by one-way analysis of variance (ANOVA) followed by Tukey’s multiple comparison test ( α ≤ 0.05). DC and solubility results were analyzed by two-way analysis of variance followed by Tukey’s test ( α ≤ 0.05).
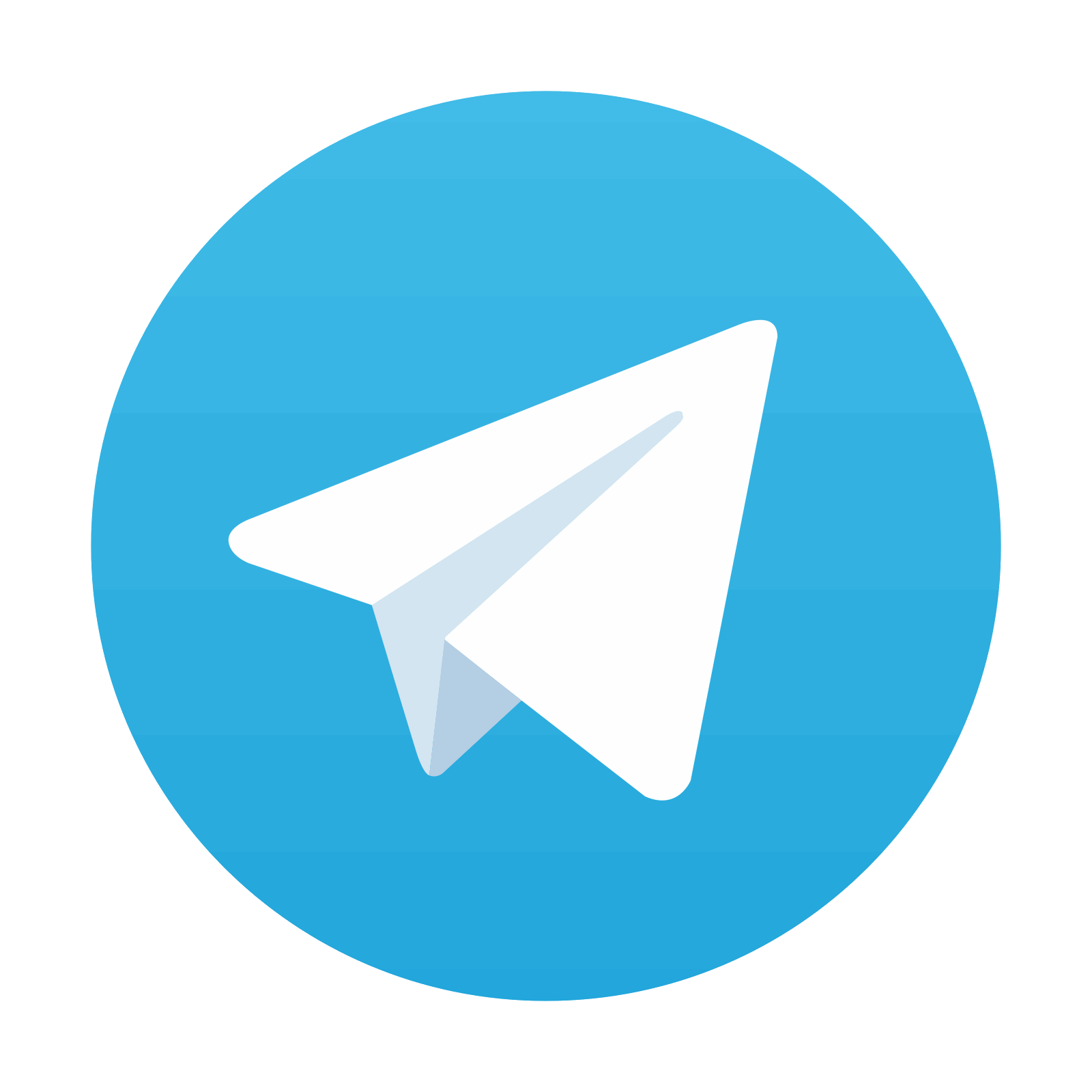
Stay updated, free dental videos. Join our Telegram channel

VIDEdental - Online dental courses
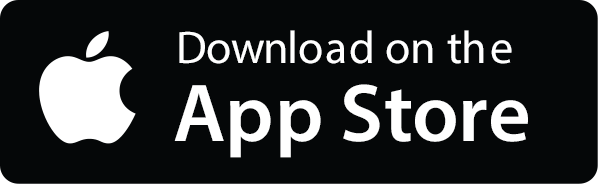
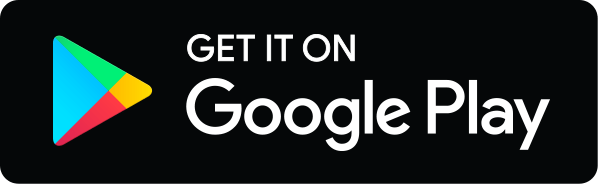