Abstract
Introduction
Previous research revealed that during routine abrasive procedures like polishing, shaping or removing of composites, high amounts of respirable dust particles (<5 μm) including nano-sized particles (<100 nm) may be released.
Objective
To determine the cytotoxic potential of composite dust particles on bronchial epithelium cells.
Methods
Composite dust of five commercial composites (one nano-composite, two nano-hybrid and two hybrid composites) was generated following a clinically relevant protocol. Polymerized composite samples were cut with a rough diamond bur (grain size 100 μm, speed 200,000 rpm) and all composite dust was collected in a sterile chamber. Human bronchial epithelial cells (16HBE14o-) were exposed to serially diluted suspensions of composite dust in cell culture medium at concentrations between 1.1 and 3.3 mg/ml. After 24 h-exposure, cell viability and membrane integrity were assessed by the WST-1 and the LDH leakage assay, respectively. The release of IL-1β and IL-6 was evaluated. The composite dust particles were characterized by transmission electron microscopy and by dynamic and electrophoretic light scattering.
Results
Neither membrane damage nor release of IL-1β was detected over the complete concentration range. However, metabolic activity gradually declined for concentrations higher than 660 μg/ml and the release of IL-6 was reduced when cells were exposed to the highest concentrations of dust.
Significance
Composite dust prepared by conventional dental abrasion methods only affected human bronchial epithelial cells in very high concentrations.
1
Introduction
During last decade, the use of engineered nanoparticles (NP), which are particles with a size smaller than 100 nm in all dimensions, has tremendously been rising. They are used in the production of electronics, tires, fuel cells, cosmetics, drugs and numerous other products. However, potential health effects during and after exposure to this particles have to date still not been sufficiently investigated and nanotoxicology has emerged as a new field within toxicology .
Also in dental materials engineered NP are used, in particular as filler particles in restorative composites. The addition of filler particles to composites is important to reduce polymerization shrinkage, to reduce thermal expansion and contraction stresses, to increase the mechanical strength, and to reduce water sorption . Most used filler particles consist of silicon based materials under the form of pyrogenic silica or aluminum silicate glasses. These particles nowadays also contain some extra elements with high atomic masses, such as barium, strontium, zirconium or ytterbium to render composites radiopaque. Although the size of these amorphous glass particles is usually between 0.4 and 1 μm, they are usually combined with nano-particles (typically pyrogenic silica) to obtain optimal packing and filler load. To date, only one manufacturer succeeded in developing a composite material (Filtek Supreme XTE, 3M ESPE, St-Paul, MN, USA) consisting only of nano-sized filler particles while preserving the right viscosity necessary for manual manipulation.
Previous research showed that relatively high amounts of so-called ‘respirable dust’, which is the fraction of inhalable dust that may penetrate deeply into the lungs due to its small dimensions (<5 μm), can be released during routine procedures such as grinding and polishing new composites . More in-depth research using state-of-the-art equipment revealed that most airborne composite dust was even nano-sized, irrespective of the type of composite . Most composite dust consisted of small pieces of composite with the filler particles held together by resin, but single filler particles could also be observed by transmission electron microscopy (TEM) . Considering the still increasing use of composites, not only as restorative material to restore decayed, traumatized or worn teeth, but also as pits and fissure sealant, and bracket adhesive in orthodontic dentistry, dentists and dental assistants may be exposed to this ultrafine (particulate matter with aerodynamic diameter <100 nm) composite dust on a daily basis.
Depending on the chemical composition, reactivity and size, respirable dust may induce adverse pulmonary and extra-pulmonary health effects . Most of the toxicological studies, with regard to composite materials, have focused on the toxicity of released components from bulk samples, while there is a lack of information regarding the toxicity of composite materials in the form of composite dust. There are, however, some reports that indicate that dental personnel is at higher risk for developing pulmonary symptoms, such as asthma .
The aim of this study was to examine different cell responses of human bronchial epithelial cells after exposure to composite dust particles. Viability, cytotoxicity, as well as the release of IL-1β and IL-6 were evaluated using a bronchial epithelial cell culture model. The null hypothesis of this study was that composite dust is not toxic for human bronchial epithelial cells.
2
Materials and methods
2.1
Particulate matter sampling
Five different commercial composites were used in this study ( Table 1 ). They represent four different composite types according to filler size, including nano-composite, nano-hybrid, micro-filled hybrid and conventional hybrid composite. Samples in the form of composite sticks, with size 17.4 × 5.4 × 1.6 mm, were prepared in a metal mold. After application in the mold, the unpolymerized composite was covered with a glass plate in order to avoid the formation of an oxygen inhibition layer. Both sides of the samples were cured for 40 s according to the manufacturers’ instructions, with a light-curing lamp (Bluephase 20i, Ivoclar-Vivadent, Schaan, Liechtenstein) with an output >1100 mW cm −2 . After quick contact decontamination (5 s in 70% ethanol (Hydral 70, VWR, Haasrode, Belgium)), composite samples were transferred to a sterile vial, which was sealed by an autoclavable sheet (Sterichamps ® S4, Paul Hartman AG, Selestat Cedex, France) ( Fig. 1 ). The vial was beforehand treated for 4 h at 200 °C to remove possible endotoxin contamination and subsequently autoclaved together with a counter-angle handpiece (Kavo Intracompact handpiece, Kavo, Biberach, Germany). Next, the handpiece was connected outside the vial to an electric micro-motor (EWL K9, Kavo) and the composite sample was ground with a rough diamond bur (842314014 Komet, Lemgo, Germany, grain size 100 μm). For each composite, another bur was used, which was cleaned ultrasonically in between. After maximum 5 times, the bur was replaced. All composite dust was collected in the vial. The whole procedure was performed in a laminar flow cabinet and composite dust was checked for bacterial contamination by incubation of the suspensions in bacterial medium (Brain heart infusion, BHI, Becton Dickinson and Company, Franklin Lakes, NJ, USA) for 24 h at 37 °C and 5% CO 2 . For each composite, this procedure was carried out following the same standardized protocol.
Composite | Filtek Supreme XTE | GrandiO | Tetric EvoCeram | Gradia Direct | Z100 MP |
---|---|---|---|---|---|
Manufacturer | 3M ESPE, Seefeld, Germany | Voco, Cuxhaven, Germany | Ivoclar—Vivadent, Schaan, Liechtenstein | GC, Tokyo, Japan | 3M ESPE, St Paul, MN, USA |
Classification | Nano-composite | Nano-hybrid composite | Nano-hybrid composite | Micro-filled hybrid composite | Hybrid composite |
Resin matrix | BisGMA, BisEMA, UDMA, TEGDMA | BisGMA, TEGDMA | BisGMA, UDMA, BisEMA | UDMA | BisGMA, TEGDMA |
Filler |
|
|
|
|
|
Filler loading | 78 wt.% | 73 wt.% | 87 wt.% | 82–83 wt.% | 84.5 wt.% |
59.5 vol.% | 64 vol.% | 71.4 vol.% | 66–67 vol.% | 66 vol.% |
Afterwards, composite dust particles were transferred to endotoxin free glass test tubes, suspended with cell culture medium without phenol red (DMEM/F-12, Gibco Life Technologies, Karlruhe, Germany) supplemented with 0,2% FBS (Fetal Bovine Serum, Gibco Life Technologies, Ghent, Belgium), ultra-sonicated for 16 min , and serially diluted to obtain different particle concentration ranging from (1.1 μg/ml to 3.3 mg/ml).
2.2
Cell and culture conditions
Human immortalized bronchial epithelial cells (16HBE14a-) were obtained as a gift from Dr. Gruenert (University of California, San Francisco, USA). They were cultured in DMEM/F-12 supplemented with 5% FBS (fetal bovine serum), 1% Streptomycin, 1% Fungizone, 1% l -glutamine (all from Gibco, Life Technologies, Gent, Belgium) at 37 °C in a 95% humidified and 5% CO 2 saturated environment. Only passages lower than 10 were used.
2.3
Cytotoxicity
16HBE14a- cells were seeded on the 96 well plate at a density of 200 000 cells cm −2 . After an incubation period of 24 h and reaching confluency, the medium was removed and the cells were exposed to the composite dust suspensions (1.1 μg/ml – 3.3 mg/ml) for 24 h. As negative and positive control, medium and 1% Triton X (Sigma Chemical CO, P.O. St Louis MO, USA) in medium were added, respectively .
To evaluate cell viability, the WST-1 assay (WST-1, Roche Diagnostic, Mannheim, Germany) was performed. This assay evaluates the viability of cells by spectrophotometric quantification of the production of formazan, which is the cleavage product of the tetrazolium salt WST-1. After 24 h exposure, the supernatant was removed and cells were washed three times with phosphate buffered saline (PBS + , Sigma–Aldrich, Life Sciences, St Louis, MO, USA). WST-1 reagent (5% in medium) was added to the cells and after 2 h in the incubator (humidified 95% air and 5% CO 2 at 37 °C), quantification of formazan was performed spectrophotometrically (Multiscan Ascent, Thermo electron corporation, Vantaa, Finland) at 450 nm (background measurements at 630 nm). In order to prevent potential interaction of deposited particles with the spectrophotometer, the WST-1 reagent was transferred to a new 96 well plate before analysis. Each test was performed in triplicate and repeated for 4 independent times (n = 4). Additional tests to evaluate interference of the particles were performed: WST-1 reagent was mixed with the highest concentration of composite dust suspension (3.3 mg/ml) in medium, supplemented with 0.2% FBS and consequently incubated for 2 h. After centrifugation, and transferring the supernatant to the new 96 well plate, the samples were measured spectrophotometrically at 450 nm. This was compared with spectrophotometrically measured WST-1 reagent alone, after the same incubation time.
The cell membrane integrity was evaluated using the LDH leakage assay assessing the transformation of lactate to pyruvate by the intracytoplasmic enzyme lactate dehydrogenase (LDH). After incubation of the cells with the particles for the 24 h, the supernatant was harvested (LDH supernatant ) and the cells were washed 2 times with PBS (Phosphate Buffered Saline, Sigma–Aldrich, Life Sciences), and subsequently lysed with 0.2% Triton X for 30 min to obtain ‘LDH lystate ’. Subsequently, 50 μl of both ‘LDH supernatant ’ and ‘LDH lystate ’, were transferred into a new 96 well plate and mixed with 100 μl HBSS (Hanks Balanced Salt Solution, Gibco, Life Technologies, Gent, Belgium). This was followed by the addition of 100 μl LDH measurement mixture right before spectrophotometric measurement at 340 nm, in order to observe reduction of pyruvate. LDH measurement mixture was prepared dissolving 0.458 mg/ml of pyruvate (CAS number: 113-24-6, Sigma–Aldrich, Life Sciences), 0.532 mg/ml of β-nicotinamide adenine dinucleotide (NADH + , CAS number: 606-68-8 Sigma–Aldrich, Life Sciences) and 0.794 mg/ml of sodium bicarbonate (CAS number: 144-55-8, Sigma–Aldrich, Life Sciences) in DPBS (Dulbecco Phosphate Buffered Saline without Ca and Mg, Gibco, Life Technologies, Gent, Belgium). Light absorption was measured continuously every 15 s for 3 min. The amount detected in the lysate represented the maximum LDH release from the cells (=100%) and the values from the supernatant were expressed as a percentage of this maximum. Membrane damage was calculated according to following formula:
% membrane damage = LDH ( lystate ) LDH ( lystate ) + LDH ( supernatant ) × 100
Each test was performed in triplicate and repeated for 4 independent times (n = 4).
2.4
Analysis of cytokine release
Confluent cells seeded in a 96-well plate were exposed to four different concentrations (3.3 mg/ml, 660 μg/ml, 330 μg/ml, 33 μg/ml) and the supernatant was collected after 24 h and stored until the measurements were performed. The levels of the pro-inflammatory cytokines, IL-1β and IL-6, in the supernatant were evaluated using an Enzyme Linked Immunosorbent assay (ELISA, Novex, Life technologies and BioSource Nivelles, Belgium), for each cytokine following the instructions of the manufacturer. Unexposed cultures were used as negative control, and cultures exposed for 24 h to Poly- l -arginine hydrochloride (3 mM in DMEM/F12 medium, CAS number: 26982-20-7, Sigma–Aldrich) or 24 h to lipopolysaccharide from Escherichia coli , 0111:B4 (70 μg/ml, Sigma–Aldrich), respectively. The results were obtained spectrophotometrically at 450 nm. All measurements were done in triplicate for each concentration of each composite group (n = 3). Evaluation of possible interference with the particles was done according to the manufacturer suggestions: the highest concentration of both IL-1β and IL-6 standards (125 pg/ml) was mixed with 3.3 mg/ml and 660 μg/ml of composite suspensions and evaluated spectrophotometrically.
2.5
TEM Characterization of composite particles
Characterization of composite particles was performed by Transmission Electronic Microscopy (TEM) (JEOL, JEM-1200 EX II Tokyo, Japan) at a voltage of 80 kV. The dust was suspended in the cell medium, ultrasonicated as described before, and one drop was applied on a TEM formvar coated copper grid (FF200H-Cu-50, Electron Microscopy Sciences, Hatfield, PA, USA). After 24 h drying under dust-free conditions, the grids were analyzed under the microscope.
2.6
Characterization of composite dust by dynamic light and electrophoretic light scattering
The intensity weighted hydrodynamic particle diameter and the zeta potential of the composite dust particles were measured directly after suspension preparation ( as described before ) using dynamic light scattering (DLS) and electrophoretic light scattering (ELS) respectively. Both instruments used the Laser Doppler Method with an He-Ne Laser with 633 nm and angle: 2 = 165°.
DLS (Delsa™ Nano C system, Beckman Coulter, Fullerton, CA, USA; Software Version 3.7) was performed to determine the agglomeration level and the average size of the particles. It analyzes the velocity distribution of the particle movements by measuring the dynamic fluctuations of light scattering intensity caused by Brownian motions of the particle. For the calculations of the intensity weighted particle size distribution CONTIN Algorithm was applied. The particle size measurements were conducted in triplicate. The zeta potential, which is a measure for the surface charge of composite particles in the cell culturing medium, was determined by ELS (Delsa™ Nano C system, Beckman Coulter, Fullerton, CA, USA). To avoid multiple scattering, the composite particle suspensions had to be diluted to 3.3 mg/ml and 660 μg/ml (only for GrandiO), respectively.
2.7
Statistics
In order to assess the WST-1 and LDH assay data, a linear mixed effects model was constructed using statistical software (R 3.2.0 and nlme package, R Foundation for Statistical Computing, Vienna, Austria). The well plate where each measurement originated from was taken into account. In this model ‘composite’ and logarithmically transformed ‘concentration’ were considered, along with their interaction. All tests were performed at a significance level of α = 0.05.
The results from the cytokine analysis were analyzed using two-way ANOVA with ‘composite’ and ‘concentration’ as factors.
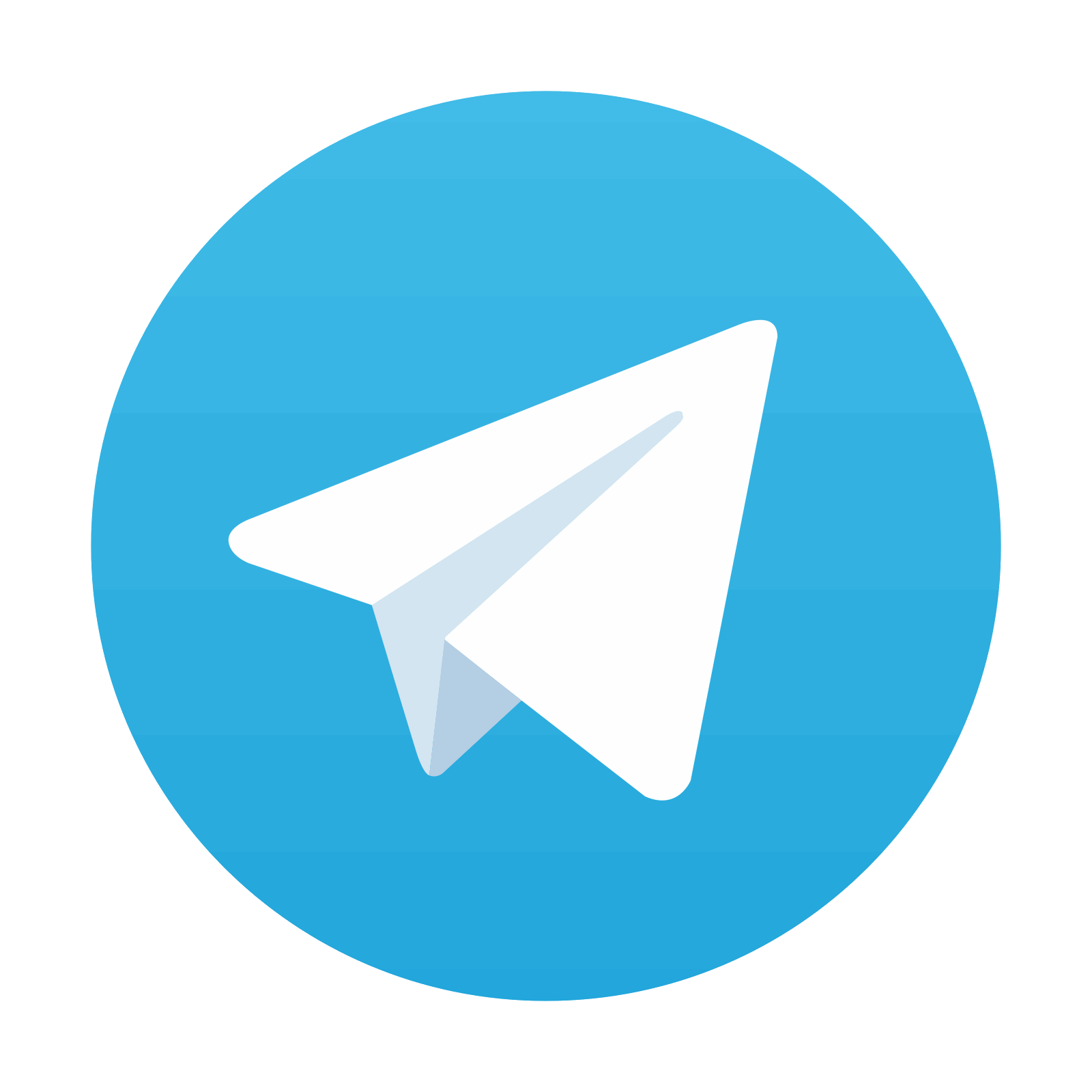
Stay updated, free dental videos. Join our Telegram channel

VIDEdental - Online dental courses
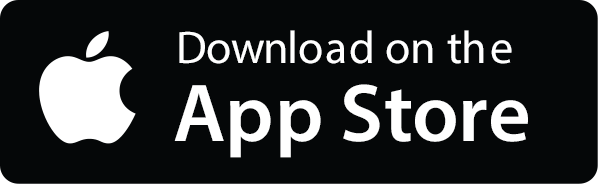
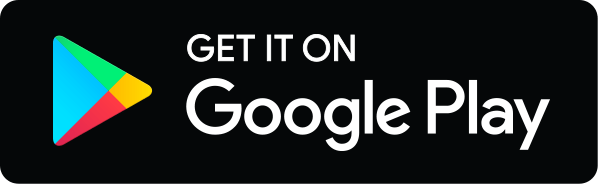