Abstract
Objective
Although aluminum oxide and silicon oxide nanoparticles are currently available as dental materials, there is a lack of basic information concerning their biocompatibility. This study evaluates the biological responses of cultured macrophages (RAW264) to aluminum oxide (Al 2 O 3 NPs) and silicon oxide nanoparticles (SiO 2 NPs) by analyzing cytotoxicity and genotoxicity.
Methods
The nanoparticles are amorphous and spherical, with diameters of 13 nm for the Al 2 O 3 NPs and 12 nm for the SiO 2 NPs. The cultured RAW264 are exposed to the nanoparticles (NPs) and examined for cytotoxicity using the WST-8 cell viability and Hoechst/PI apoptosis assay, for genotoxicity by micronucleus analysis, for changes in nuclear shape (deformed nuclei) and for comet assay using confocal microscopy, and micromorphological analysis is done using scanning and transmission electron microscopes.
Results
Nuclei and DNA damage because of exposure to both types of NPs is observed by inmunostaining genotoxicity testing. The cytotoxicity and genotoxicity are well correlated in this study. Numerous NPs are observed as large aggregates in vesicles, but less or nonexistent NP internalization is seen in the nucleus or cytoplasm. These morphological results suggest that a primary cause of cell disruption is the chemical changes of the NPs in the low pH of vesicles (i.e., ionization of Al 2 O 3 or SiO 2 ) for both types of oxide NPs.
Significance
Although further research on the elution of NP concentrations on cell or tissue activity under simulated clinical conditions is required, NP concentrations over 200 μg/mL are large enough to induce cytotoxic and genotoxic effects to cells.
1
Introduction
Nanoscale particles are one of the fastest growing sectors in materials research and are currently being used in a wide range of fields, such as industrial, military, and biomedical applications, owing to their unique size-dependent properties. The main characteristic properties of the nanoparticle, namely quantum size effects and surface-induced effects, result from its small size. A large number of nanoparticle products are currently in development and under investigation, and some are already commercially available. For dental applications, numerous new dental products containing nanoparticles have been developed and are available for implants, pulp capping agents, restorative materials, tooth-paste, and oral hygiene products . Titanium dioxide, silicon dioxide, aluminum oxide, and zinc oxide are commonly available for a variety of applications in dental materials. Nano-sized silicon dioxide is one of the most popular nanomaterials for restorative materials such as resin composites or bonding resins . In addition, nano-sized aluminum oxide is used in orthopedic materials . Recently, these two materials have been used as pulp capping agents and as a surface coating for dental implants that come in direct contact with pulp tissues and periodontium .
Although dental applications require nanoparticles (NPs) with a high degree of biocompatibility to protect human health, there is a serious lack of basic information concerning NP toxicity, and especially genotoxicity. A rapid approach for risk assessment is needed because of the rapid development of dental products using various particles with different chemical and physiological properties. Toxicological studies of the interaction of NPs with biological systems, including living cells and organs, have spurred a growing awareness of the need to predict the possible hazard of NPs via collaborative research in science and industry. Nanoparticle sizes are similar to that of cellular components, such as nuclei, mitochondria, and lysosomes, and they may be able to bypass cellular barriers, thereby leading to unexpected adverse tissue reactions. Therefore, an important topic in NP research is basic morphological information on the location and internalization of NPs; namely, where and how NPs are incorporated into cellular components.
Because aluminum oxide and silicon oxide NPs are commonly used in dental materials, the purpose of this study is to evaluate the effects of aluminum oxide NPs (13 nm diameter) and silicon oxide NPs (12 nm diameter) upon cultured RAW264 using cytotoxicity (WST-8 assay), immunostaining genotoxicity (deformed nuclei, micronuclei, Hoechst/PI apoptosis, and comet assay) and micromorphological analysis of NP internalization using scanning and transmission electron microscopes. The null hypothesis in this work is that there exist no cytotoxic and genotoxic effects from aluminum oxide and silicon oxide NPs upon RAW264 cells.
2
Materials and methods
2.1
Nanoparticles
Two types of Al 2 O 3 NPs (Al 2 O 3 NPs) and SiO 2 NPs (SiO 2 NPs) (Sigma–Aldrich, St. Louis, MO, USA) were used in this study. The transmission electron microscope (TEM) samples were prepared by evaporating a droplet of the aqueous dispersion of NPs onto a carbon-coated copper grid. The crystallinity and surface charge of the NPs were characterized by an X-ray diffractometer (XRD; Rint 2100; Rigaku, Tokyo, Japan) and a zeta potential analyzer (ZetaPALS, Brookhaven Instruments, NY, USA), respectively.
2.2
Cell culture and preparation of the conditioned medium
The RAW264 murine macrophage cell line (Riken Biosource Center Cell Bank, Tsukuba, Japan) was cultured at 37 °C in an α-minimal essential medium (Wako Chemical, Tokyo, Japan) containing 10% fetal bovine serum (Biowest, Nuaillé, France) and 1% antibiotic–antimycotic (Anti-Anti, Gibco, NY, USA) in a humidified atmosphere of 5% CO 2 in air. Cells were grown in a cell culture dish and checked using a microscopic control that was free of any contamination. Confluent cells were detached using a cell scraper and aliquots of separated cells were subcultured at 1:4 splits every 3 days.
2.3
Exposure of cells to nanoparticles
Cells were seeded in Falcon 96-well plates (Corning, NY, USA) at a density of 1 × 10 4 cells per well with 200 μL of medium. After 72 h of culturing, the medium was removed and 200 μL of medium containing two different NP concentrations (200 and 400 μg/mL) was added to the 72 h cultured cells. Cells that had not been treated using the NPs were used as a control. The final particle dispersions were prepared fresh before use by serial dilution of the stock suspension (400 μg/mL) in medium. The stock solution was mixed using a vortex, and then sonicated for 5 min to reduce agglomeration of the nanoparticles. The NP-exposed cells were subsequently incubated for 24 h.
2.4
Cell viability
The cell viability was measured using a WST-8 assay (CCK-8, Dojindo, Kumamoto, Japan). The cells were incubated in medium in the 96-well plates (1 × 10 4 cells/200 μL medium/well) for 72 h, and subsequently for 24 h after being exposed to the NPs. The medium was then removed via aspiration and the cells were washed twice using phosphate-buffered saline (PBS). The medium and WST-8 test solution was then added, and the plate was incubated at 37 °C for 1 h, after which the cell supernatant was placed in new 96-well plates and the absorbance at 450 nm was measured using a microplate reader (Model 680, Bio-Rad, Hercules, CA, USA). The test medium was carefully transferred to the new 96-well plate to avoid NP contamination in the medium. Blank and medium controls (medium containing NPs without cells) were treated identically.
2.5
Micronuclei analysis and changes in nuclear shape (deformed nuclei)
Nuclear morphology and distribution were investigated by immunocytochemistry. An established biomarker for genomic instability was carried out to evaluate the susceptibility of the cells to NP-induced chromosomal damage endpoints (micronuclei and deformed nuclei). Following exposure to the NPs, the cells were incubated with Hoechst 33342 (Dojindo) for 30 min. The number of cells exhibiting micronuclei and deformed nuclei was counted, with over 400 nuclei analyzed per treatment time point and all analyses performed in triplicate. The cell numbers were scored under laser scanning confocal microscopy (TS1200-E; Nikon, Tokyo, Japan).
2.6
Hoechst/PI apoptosis assay
After each incubation period under test conditions, the cells were rinsed twice with PBS and then incubated with Hoechst 33342 and propidium iodine (PI; Dojindo) as nuclear probes in PBS for 30 min at 37 °C. Use of Hoechst 33342 and PI staining helped distinguish between apoptosis and necrosis owing to the difference in the permeability of the cell membranes of live and damaged cells. Subsequently, the cells were rinsed three times with PBS and analyzed using laser scanning confocal microscopy (TS1200-E) at 40× magnification via oil. The groups were categorized such that Hoechst (+)/PI (−) were living cells, and Hoechst (+)/PI (+) were late apoptosis cells. The cells stained with PI alone (Hoechst (−)/PI (+)) were represented as necrotic cells that were excluded from the data.
2.7
Alkaline single-cell gel electrophoresis (comet assay)
The DNA damage in RAW264 cells was analyzed using the alkaline comet assay. Treated cells were rinsed with PBS and resuspended in Hank’s balanced salt solution (Sigma–Aldrich) with 10% dimethyl sulfoxide (DMSO) and (ethylenedinitriol)tetraacetic acid (EDTA). The cells were embedded in agarose gel on comet slides (Trevigen, Gaithersburg, MD, USA) and lysed in a prechilled lysis solution (2.5 M NaCl, 0.1 M EDTA, 10 mA Tris base, pH 10) with 1% Triton X for 1 h at 4 °C. The cells were then subjected to denaturation in an alkaline buffer (0.3 M NaCl, pH 7.5) for 20 min. Electrophoresis was performed at 21 V and 300 mA for 30 min in a cooled chamber. After electrophoresis, the slides were washed with water and dehydrated using 70% ethanol. The slides were subsequently stained with SYBR ® green dye (Invitrogen, Carlsbad, CA, USA). Images of the samples were observed and captured using fluorescence microscopy (TS1200-E). For the scoring method, a total of 50 comets on each gel were classified as belonging to one of five categories according to the tail and head intensity. Each category was given a value between 0 and 4, and the total scores of the slide could be between 0 and 400 arbitrary units .
2.8
SEM and TEM analysis of cells with internalized nanoparticles
For SEM and TEM analysis, the cells incubated with and without NPs were washed using PBS, and fixed with 2.5% glutaraldehyde for 24 h. The cells were then post-fixed in 1% osmium tetraoxide for 1 h and subsequently washed and dehydrated in graded concentrations of ethanol (50, 60, 70, 80, 90, and 100%) and hexamethyl-disilazane (Wako). For SEM analysis, the specimens were sputter-coated with gold and observed using SEM (S-3500N, Hitachi, Tokyo, Japan) with an accelerating voltage of 15 kV in a vacuum. For TEM analysis, the cell specimens were infiltrated and embedded in Epoxy resin (EPON 812, TAAB, Berks, UK). Ultrathin sections (90 nm) were subsequently cut using a diamond knife on a microtome (Sorvall MT-5000, Du Pont, Wilmington, DE, USA). The sections were collected on copper grids and stained with saturated aqueous uranyl acetate, counter stained with 4% lead citrate, and observed with the TEM (H-7100; Hitachi, Tokyo, Japan).
2.9
Statistics
Three independent experiments were conducted, and the results were expressed as the mean ± standard deviation. The Mann–Whitney U test was applied to compare the mean values for the control and treatment groups of cell viability (WST-8 assay) and genotoxicity (frequency of deformed nuclei, micronuclei, Hoechst/PI apoptosis and DNA damage). Statistically, the treatment groups were significantly different from the control groups ( p < 0.05 for each).
2
Materials and methods
2.1
Nanoparticles
Two types of Al 2 O 3 NPs (Al 2 O 3 NPs) and SiO 2 NPs (SiO 2 NPs) (Sigma–Aldrich, St. Louis, MO, USA) were used in this study. The transmission electron microscope (TEM) samples were prepared by evaporating a droplet of the aqueous dispersion of NPs onto a carbon-coated copper grid. The crystallinity and surface charge of the NPs were characterized by an X-ray diffractometer (XRD; Rint 2100; Rigaku, Tokyo, Japan) and a zeta potential analyzer (ZetaPALS, Brookhaven Instruments, NY, USA), respectively.
2.2
Cell culture and preparation of the conditioned medium
The RAW264 murine macrophage cell line (Riken Biosource Center Cell Bank, Tsukuba, Japan) was cultured at 37 °C in an α-minimal essential medium (Wako Chemical, Tokyo, Japan) containing 10% fetal bovine serum (Biowest, Nuaillé, France) and 1% antibiotic–antimycotic (Anti-Anti, Gibco, NY, USA) in a humidified atmosphere of 5% CO 2 in air. Cells were grown in a cell culture dish and checked using a microscopic control that was free of any contamination. Confluent cells were detached using a cell scraper and aliquots of separated cells were subcultured at 1:4 splits every 3 days.
2.3
Exposure of cells to nanoparticles
Cells were seeded in Falcon 96-well plates (Corning, NY, USA) at a density of 1 × 10 4 cells per well with 200 μL of medium. After 72 h of culturing, the medium was removed and 200 μL of medium containing two different NP concentrations (200 and 400 μg/mL) was added to the 72 h cultured cells. Cells that had not been treated using the NPs were used as a control. The final particle dispersions were prepared fresh before use by serial dilution of the stock suspension (400 μg/mL) in medium. The stock solution was mixed using a vortex, and then sonicated for 5 min to reduce agglomeration of the nanoparticles. The NP-exposed cells were subsequently incubated for 24 h.
2.4
Cell viability
The cell viability was measured using a WST-8 assay (CCK-8, Dojindo, Kumamoto, Japan). The cells were incubated in medium in the 96-well plates (1 × 10 4 cells/200 μL medium/well) for 72 h, and subsequently for 24 h after being exposed to the NPs. The medium was then removed via aspiration and the cells were washed twice using phosphate-buffered saline (PBS). The medium and WST-8 test solution was then added, and the plate was incubated at 37 °C for 1 h, after which the cell supernatant was placed in new 96-well plates and the absorbance at 450 nm was measured using a microplate reader (Model 680, Bio-Rad, Hercules, CA, USA). The test medium was carefully transferred to the new 96-well plate to avoid NP contamination in the medium. Blank and medium controls (medium containing NPs without cells) were treated identically.
2.5
Micronuclei analysis and changes in nuclear shape (deformed nuclei)
Nuclear morphology and distribution were investigated by immunocytochemistry. An established biomarker for genomic instability was carried out to evaluate the susceptibility of the cells to NP-induced chromosomal damage endpoints (micronuclei and deformed nuclei). Following exposure to the NPs, the cells were incubated with Hoechst 33342 (Dojindo) for 30 min. The number of cells exhibiting micronuclei and deformed nuclei was counted, with over 400 nuclei analyzed per treatment time point and all analyses performed in triplicate. The cell numbers were scored under laser scanning confocal microscopy (TS1200-E; Nikon, Tokyo, Japan).
2.6
Hoechst/PI apoptosis assay
After each incubation period under test conditions, the cells were rinsed twice with PBS and then incubated with Hoechst 33342 and propidium iodine (PI; Dojindo) as nuclear probes in PBS for 30 min at 37 °C. Use of Hoechst 33342 and PI staining helped distinguish between apoptosis and necrosis owing to the difference in the permeability of the cell membranes of live and damaged cells. Subsequently, the cells were rinsed three times with PBS and analyzed using laser scanning confocal microscopy (TS1200-E) at 40× magnification via oil. The groups were categorized such that Hoechst (+)/PI (−) were living cells, and Hoechst (+)/PI (+) were late apoptosis cells. The cells stained with PI alone (Hoechst (−)/PI (+)) were represented as necrotic cells that were excluded from the data.
2.7
Alkaline single-cell gel electrophoresis (comet assay)
The DNA damage in RAW264 cells was analyzed using the alkaline comet assay. Treated cells were rinsed with PBS and resuspended in Hank’s balanced salt solution (Sigma–Aldrich) with 10% dimethyl sulfoxide (DMSO) and (ethylenedinitriol)tetraacetic acid (EDTA). The cells were embedded in agarose gel on comet slides (Trevigen, Gaithersburg, MD, USA) and lysed in a prechilled lysis solution (2.5 M NaCl, 0.1 M EDTA, 10 mA Tris base, pH 10) with 1% Triton X for 1 h at 4 °C. The cells were then subjected to denaturation in an alkaline buffer (0.3 M NaCl, pH 7.5) for 20 min. Electrophoresis was performed at 21 V and 300 mA for 30 min in a cooled chamber. After electrophoresis, the slides were washed with water and dehydrated using 70% ethanol. The slides were subsequently stained with SYBR ® green dye (Invitrogen, Carlsbad, CA, USA). Images of the samples were observed and captured using fluorescence microscopy (TS1200-E). For the scoring method, a total of 50 comets on each gel were classified as belonging to one of five categories according to the tail and head intensity. Each category was given a value between 0 and 4, and the total scores of the slide could be between 0 and 400 arbitrary units .
2.8
SEM and TEM analysis of cells with internalized nanoparticles
For SEM and TEM analysis, the cells incubated with and without NPs were washed using PBS, and fixed with 2.5% glutaraldehyde for 24 h. The cells were then post-fixed in 1% osmium tetraoxide for 1 h and subsequently washed and dehydrated in graded concentrations of ethanol (50, 60, 70, 80, 90, and 100%) and hexamethyl-disilazane (Wako). For SEM analysis, the specimens were sputter-coated with gold and observed using SEM (S-3500N, Hitachi, Tokyo, Japan) with an accelerating voltage of 15 kV in a vacuum. For TEM analysis, the cell specimens were infiltrated and embedded in Epoxy resin (EPON 812, TAAB, Berks, UK). Ultrathin sections (90 nm) were subsequently cut using a diamond knife on a microtome (Sorvall MT-5000, Du Pont, Wilmington, DE, USA). The sections were collected on copper grids and stained with saturated aqueous uranyl acetate, counter stained with 4% lead citrate, and observed with the TEM (H-7100; Hitachi, Tokyo, Japan).
2.9
Statistics
Three independent experiments were conducted, and the results were expressed as the mean ± standard deviation. The Mann–Whitney U test was applied to compare the mean values for the control and treatment groups of cell viability (WST-8 assay) and genotoxicity (frequency of deformed nuclei, micronuclei, Hoechst/PI apoptosis and DNA damage). Statistically, the treatment groups were significantly different from the control groups ( p < 0.05 for each).
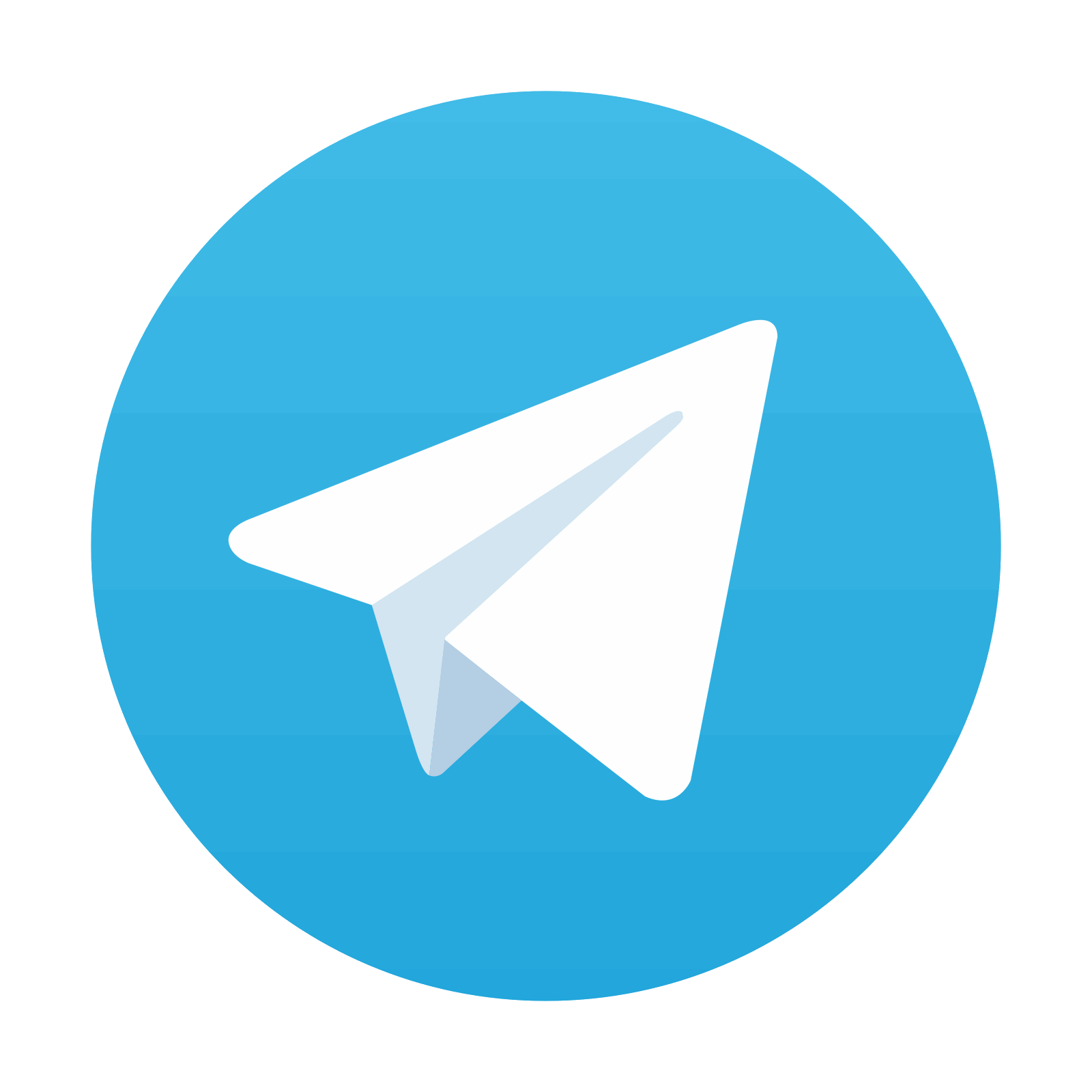
Stay updated, free dental videos. Join our Telegram channel

VIDEdental - Online dental courses
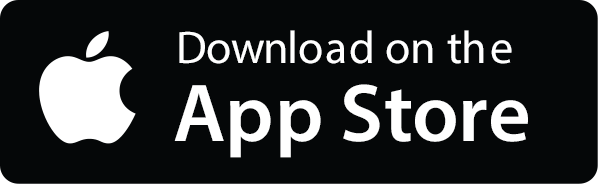
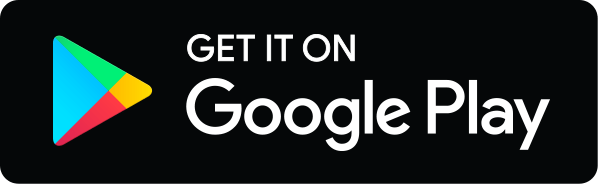