Introduction
Current craniofacial growth modification devices use static forces, but cyclic forces are believed by some to be more effective. The latter have not been evaluated in large animal models, and it is not known how such forces are transmitted to distant parts of the skull. In this study, we aimed to (1) develop a portable loading system capable of delivering reliable cyclic loads to the porcine nasofrontal suture (NFS), (2) explore strain transmission to distant sutures, and (3) characterize the sutural growth effects in a small pilot study.
Methods
After we validated the device, cyclic (2.5 Hz) tensile loads were applied unilaterally to the NFS of 6 abattoir pig heads, with strain gauges on multiple sutures. Similar loading was applied to 3-month-old live pigs ( Sus scrofa , n = 4 and 1 sham) 30 minutes per day for 5 days. These animals received fluorescent markers of mineralization on loading days 1 and 3. Suture strains were recorded on day 5. Histomorphometric analysis quantified suture width and mineral apposition rate.
Results
A wearable loading system was developed to produce an average of +900 microstrain at the targeted NFS. Substantial strains were seen at the contralateral NFS and midline sutures, but bone strains were low. Strain patterns were similar ex vivo and in vivo, with the latter generally having higher magnitudes. Preliminary evidence demonstrates wider sutures with higher mineral apposition rates in the loaded sutures.
Conclusions
Daily spurts of cyclic load caused sutural strain throughout the skull. This regimen most likely enhances sutural growth and may be therapeutically useful.
Highlights
- •
A wearable device was developed for cyclic loading of the craniofacial skeleton.
- •
Single-suture cyclic loading can transmit strain to distant parts of the skull.
- •
Strain transmission causes rotation of skull bones with sutures as break points.
- •
Direct cyclic tensile loading increases suture width and mineral apposition rate.
- •
Short spurts of cyclic loading may efficiently manage dentofacial deformities.
Underdevelopment of the craniofacial skeleton due to early sutural fusion can lead to severe deformities with impairments in feeding, respiration, and esthetics. Current dentofacial orthopedic growth modification devices use static forces, but studies in small animal models suggest that cyclic forces may be more efficient in enhancing suture growth. However, such studies need to be replicated in large mammals because of size effects on skeletal biology. Moreover, the small size of previous models has prevented an understanding of how sutural loading affects the rest of the skull. If these loads are transmitted through the skull, the growth of neighboring sutures could be altered as well.
In this study, we aimed to develop a large animal model to study cyclic loading and assess the degree to which such loads extend to nontargeted sutures. In this pilot study, we used a pig model to investigate how cyclic loading of the nasofrontal suture (NFS) affects strain distribution and growth at many sutures in the midface and cranium. The pig ( Sus scrofa domesticus ) was chosen because it has an adult size comparable with that of a human, and has similar patterns of embryology and ossification. The NFS was chosen because of its accessibility and major contribution to midfacial length in normal pigs. The NFS is also affected in syndromic craniosynostosis. The aims of this pilot study were (1) to develop a wearable cyclic loading system for large animals, (2) to characterize the strains produced by direct cyclic loading of the NFS on targeted and other craniofacial sutures, (3) to compare such strains in fresh ex-vivo pig heads with those in live pigs, and (4) to conduct a preliminary test of whether cyclic loading can increase sutural widths and mineral apposition rates.
Material and methods
The custom-engineered loading device used a voice coil connected to a power supply and function generator to produce cyclic forces ( Fig 1 ). A load cell on the device measured the magnitude of force output. The device was placed superior to the NFS and parallel to the midsagittal axis. Two vertically extended plates were fitted with holes and screws that engaged loading attachments on the skull. Calibration with known loads allowed conversion of output voltage into kilograms.

Loading attachments were installed on the frontal and nasal bones. To provide sufficient contrast with physiologic strain during mastication (–1600 microstrain [μɛ] 8 ), the imposed cyclic strain was tensile, but the system is capable of compressive loading as well. Frequency was set at 2 to 3 Hz to mimic the rate of a pig’s chewing. To avoid injuring the sutural tissue, the desired NFS strain magnitude was 800 to 1000 μɛ, about the mean for sutures during mastication.
Four pig heads of varying ages and provenances (D-1 to D-4; Table I ) were used to establish the load output necessary to achieve the targeted strain at the NFS. Initially, stainless steel screws were used as the loading attachment. However, these screws lacked adequate retention and were changed to stainless steel loading plates with a central loading post ( Fig 2 ). The heads were instrumented with single-element strain gauges (EP-08-125BT-120; Micro-Measurements, Wendell, NC) over the loaded and contralateral NFS. Bilateral NFS were exposed, the periosteum was removed, and the sites prepared using the BAK-200 Basic Application Kit (Micro-Measurements) before the strain gauges were affixed with cyanoacrylate glue. The lead wires from the strain gauges were connected to the strain conditioner/amplifiers (model 2110; Micro-Measurements), which were linked to a computer for data acquisition. Strain information was collected at intervals throughout the loading session and digitized using the MP150 system and Acqknowledge 3.9 software (BIOPAC Systems, Goleta, Calif). The load output from the loading device was also sent through a strain gauge conditioner/amplifier as a separate channel. Strain and load output values were simultaneously collected in Acqknowledge in voltage, transferred into Excel (Microsoft, Redmond, Wash), and converted to microstrains and kilograms, respectively. Magnitudes of the strain and load outputs were obtained by subtracting the baseline values from the peak values.
Number | Source | Loading attachment type | Strain gauges placed | Loaded NFS strain (μɛ) | Contralateral NFS strain (μɛ) |
---|---|---|---|---|---|
Heads used to develop and calibrate loading system | |||||
D-0 | Dry skull, estimated age 3 months, unknown source | Stainless steel screws: 9.5 mm length, 1.4 mm diameter | Single-element: bilateral NFS | 108 ± 3 (10) | 29 ± 2 (10) |
D-1 | Fixative-perfused head, 5.5-month-old male minipig | Stainless steel screws: 9.5 mm length, 1.4 mm diameter | Single-element: bilateral NFS | 2161 ± 4 (10) | 307 ± 1 (10) |
D-2 | Previously frozen head, dentally mature (≥2 years old) female minipig | Stainless steel screws: 9.5 mm length, 1.4 mm diameter | Single-element: bilateral NFS | 187 ± 14 (5) | –9 ± 2 (5) |
D-3 | Previously frozen abattoir head, estimated age 6 months, unknown sex | Stainless steel screws: 9.5 mm length, 1.4 mm diameter | Single-element: bilateral NFS | 241 ± 3 (20) | 162 ± 2 (20) |
D-4a | Previously frozen abattoir head, estimated age 6 months, unknown sex | Stainless steel screws: 9.5 mm length, 1.4 mm diameter | Single-element: bilateral NFS | 1369 ± 19 (5) | 764 ± 14 (5) |
D-4b | Previously frozen abattoir head, estimated age 6 months, unknown sex | Stainless steel plates each stabilized by 2 screws ( Fig 2 ) | Single-element: bilateral NFS | 1856 ± 7 (3) | 836 ± 2 (3) |
Heads used in definitive ex-vivo strain measurements | |||||
H-1 | Fresh abattoir heads, estimated age 6 months, unknown sex | Stainless steel plates each stabilized by 2 screws ( Fig 2 ) | Single-element: bilateral NFS and CS, INS, IFS. Rosette: loaded-side nasal and frontal bones. | 1272 ± 190 (20) | 693 ± 86 (20) |
H-2 | Fresh abattoir heads, estimated age 6 months, unknown sex | Stainless steel plates each stabilized by 2 screws ( Fig 2 ) | Single-element: bilateral NFS and CS, INS, IFS. Rosette: loaded-side nasal and frontal bones. | 684 ± 104 (20) | 490 ± 62 (20) |
H-3 | Fresh abattoir heads, estimated age 6 months, unknown sex | Stainless steel plates each stabilized by 2 screws ( Fig 2 ) | Single-element: bilateral NFS and CS, INS, IFS. Rosette: loaded-side nasal and frontal bones. | 737 ± 137 (20) | 664 ± 111 (20) |
H-4 | Fresh abattoir heads, estimated age 6 months, unknown sex | Stainless steel plates each stabilized by 2 screws ( Fig 2 ) | Single-element: bilateral NFS and CS, INS, IFS. Rosette: loaded-side nasal and frontal bones. | 720 ± 27 (20) | 508 ± 10 (20) |
H-5 | Fresh abattoir heads, estimated age 6 months, unknown sex | Stainless steel plates each stabilized by 2 screws ( Fig 2 ) | Single-element: bilateral NFS and CS, INS, IFS. Rosette: loaded-side nasal and frontal bones. | 1063 ± 202 (20) | 1045 ± 162 (20) |
H-6 | Fresh abattoir heads, estimated age 6 months, unknown sex | Stainless steel plates each stabilized by 2 screws ( Fig 2 ) | Single-element: bilateral NFS and CS, INS, IFS. Rosette: loaded-side nasal and frontal bones. | 914 ± 70 (20) | 558 ± 23 (20) |

Six heads (H-1 to H-6) from freshly slaughtered pigs (Kapowsin Meats, Graham, Wash), sex unknown and assumed to be about 6 months old, were used. Loading attachments (2-screw plates) were fastened on both sides of the right NFS. Single-element strain gauges were placed bilaterally on the NFS and coronal sutures, and on the internasal and interfrontal sutures as described previously. Three-element 45° stacked rosette gauges (WA-13-060WR-120; Micro-Measurements) were placed on the right nasal and frontal bones ( Fig 3 ), with the middle element perpendicular to the midsagittal plane. Cyclic loading was carried out for 30 minutes, with mean load output and strains for the beginning, middle, and final minutes of the session determined from averages of 20 waveforms each.

Five mixed-breed pigs ( Sus scrofa ; 4 male, 1 female) approximately 3 months old (Progressive Swine Farms, Woodinville, Wash) were used (P-1 to P-5, Table II ). All experimental procedures followed the National Institutes of Health guidelines for the care and use of laboratory animals and were approved by the University of Washington Institutional Animal Care and Use Committee. The animals were acclimated for approximately 1 week. Pigs P-1 and P-2 received the 2-screw plate attachments but because these became loose, the design was changed for pigs P-3 to P-5. The new design ( Fig 4 ) had a custom titanium plate with 4 flanges, which could be contoured to the natural skull curvature. Each flange was stabilized by 2 stainless steel cortex self-tapping 2-mm screws (DePuy Synthes Vet, West Chester, Pa). These produced comparable load outputs to the 2-screw design in trials using dry skulls.
Number | Sex, age (months), weight (kg) | Treatment | Bone labels | Single-element strain gauges placed | Histologic analysis |
---|---|---|---|---|---|
In vivo subjects | |||||
P-1 | Male, 4.0, 20.5 | Right NFS: stainless steel plates each stabilized by 2 screws ( Fig 2 ). Loaded 5 days but loose after day 1. | Calcein and alizarin injected 2 days apart during loading period | Bilateral NFS and CS, INS, IFS | Bilateral NFS and CS |
P-2 | Male, 4.0, 21.0 | Right NFS: stainless steel plates each stabilized by 2 screws ( Fig 2 ). Sham control (no loading), loose attachments. | Calcein and alizarin injected 2 days apart during loading period | None | Bilateral NFS and CS |
P-3 | Male, 4.0, 20.0 | Right NFS: titanium plate stabilized by 8 screws ( Fig 4 ). Stable attachments, loaded 5 days. | Calcein and alizarin injected 2 days apart during loading period | Bilateral NFS and CS, INS, IFS | Bilateral NFS and CS, INS, IFS |
P-4 | Female, 4.0, 18.6 | Right NFS: titanium plate stabilized by 7 screws ( Fig 4 ). Slightly movable nasal attachment on day 1, loaded 5 days. | Calcein and alizarin injected 2 days apart during loading period | Bilateral NFS and CS, INS, IFS | Bilateral NFS and CS, INS, IFS |
P-5 | Male, 4.0, 20.9 | Right NFS: titanium plate stabilized by 8 screws ( Fig 4 ). Stable attachments, loaded 5 days. | Calcein and alizarin injected 2 days apart during loading period | Bilateral NFS and CS, INS, IFS | Bilateral NFS and CS, INS, IFS |
Histologic controls | |||||
C-1 | Female, 5.4, 34.0 | None | Calcein and alizarin injected 5 days apart | None | Right NFS |
C-2 | Female, 5.4, 30.8 | None | Calcein and alizarin injected 5 days apart | None | Left NFS |
C-3 | Female, 5.4, 32.2 | None | Calcein and alizarin injected 5 days apart | None | Left NFS |
C-4 | Female, 3.6, 22.2 | None | Calcein and alizarin injected 5 days apart | None | Right NFS (only suture width) |

To affix the attachments, anesthesia was used with a cocktail consisting of midazolam, xylazine, and butorphanol delivered intramuscularly and maintained with isoflurane inhalation. Under aseptic conditions, arcuate incisions were made unilaterally on the right frontal and nasal bones. The NFS was not exposed. Flaps were raised with the periosteum left intact. Pilot holes were made for the screws, and the loading attachments were placed. The incisions were closed with interrupted sutures so that the loading posts projected from the skin. After 1 week of healing, cyclic loading sessions began. Pig P-2 had loose attachments and was considered a sham control. Daily for 5 consecutive days, the pigs were anesthetized as described previously, placed in a prone position, and the loading device was engaged to the loading attachments. On days 1 and 3, mineralization labels were administered (described below). Loading was conducted for approximately 30 minutes per day. In the sham control, the loading device was engaged, but no loads were applied. On the final (5th) day, before loading, under anesthesia, the periosteal flaps were elevated to expose the nasofrontal, internasal, interfrontal, and coronal sutures. Strain gauges were affixed as in the ex-vivo study, except for the unloaded sham control. Because bone strain levels recorded in the ex-vivo experiments had been low, no gauges were placed on the nasal and frontal bones. Load output and strain data were collected during cyclic loading as for the ex-vivo study. Data were analyzed by 1 investigator (S.H.S.).
Fluorochrome labels of mineral apposition were dissolved in sterile saline solution, adjusted to a neutral pH, filtered at 0.22 μm, and administered intravenously on days 1 (calcein, 12.5 mg/kg; Sigma-Aldrich, St Louis, Mo) and 3 (alizarin complexone, 12.5 mg/kg; Sigma-Aldrich) of loading.
After the loading session on day 5, the animals were injected intravenously with heparin and then killed with pentobarbital (Beuthanasia-D Special; Merck, Madison, NJ). This was followed by perfusion through the ascending aorta with approximately 4 L of saline solution and then approximately 4 L of 20% glyoxal fixative (derived from Prefer Concentrate; ANATECH, Battle Creek, Mich, diluted with reagent alcohol and deionized water). Instrumented sutures were removed and separated into 2 blocks, one for assessment of sutural width and mineral apposition, and the other for future immunohistochemistry. In addition, a randomly chosen NFS was removed from each of 4 additional pig specimens of comparable breed mix (C-1 to C-4, histologic controls; Table II ). These animals had been used in an unrelated study involving tooth mobility and periodontal disease and had received bone labels on a slightly different schedule.
The undecalcified samples were dehydrated in an ethanol series and infiltrated with Micro-Bed resin (Electron Microscopy Sciences, Hatfield, Pa) before curing for approximately 21 days in a thermal oven set at 35°C to 36°C. Sections perpendicular to the sutures at 50-μm intervals were obtained using a saw microtome (SP1600; Leica Biosystems, Buffalo Grove, Ill) and mounted on slides. One to 3 sections were selected per suture based on clarity and completeness, and examined under epifluorescent illumination (Eclipse E400; Nikon, Tokyo, Japan). Using a digital camera (SPOT RT3 2Mp Slider; Diagnostic Instruments, Sterling Heights, Mich), the same microscopic field was separately captured for calcein and alizarin. Images were then merged to show images with double fluorescent labels via the MetaVue software (Molecular Devices, Sunnyvale, Calif). Histomorphometric analysis was performed with MetaMorph software (Molecular Devices). Suture width was calculated by dividing the area between both sutural margins over the average length of the 2 sutural margins. Mineral apposition rate was calculated by measuring the area between the calcein and alizarin bone fronts and dividing it by the average suture length and the number of days between labels to give mineral apposition rates in micrometers per day. Mineral apposition rate values from each side of the suture were averaged to give the mean mineral apposition rates ( Fig 5 ). Intraexaminer reliability was determined by remeasuring 5 randomly selected samples. Selection of slides and measurements were all made by 1 investigator blinded to the identity of the samples.

Mann Whitney U-tests and unpaired t tests were used for statistical analyses, and statistical significance was determined at P <0.05 (GraphPad Prism, La Jolla, Calif).
Results
Ex vivo, the 2-screw plate loading attachments remained intact and stable, but they were unsuccessful in vivo (pigs P-1 and P-2). The 8-screw plate loading attachments in pigs P-3 to P-5 were stable, except for the nasal attachment in pig P-4 that was somewhat moveable, although it still permitted loading and strain recording.
In the ex-vivo study on the transmission of strain in pig heads H-1 to H-6, the loading device produced a mean output of 1.55 ± 0.1 kg. Strains matching the loading frequency of 2.5 Hz were registered at working gauge locations. Load output and strains tended to reduce in magnitude during the session. The highest level of strain was seen in the loaded right NFS, with a mean tensile strain of +898 ± 233 μɛ ( Fig 6 ; Table III ). The contralateral NFS also showed substantial, but lower tensile strain (+660 ± 205 μɛ). Coronal sutures experienced very low compressive strain, averaging –47 ± 30 μɛ on the loaded side and –17 ± 16 μɛ on the contralateral side. The interfrontal suture was usually in tension, with 1 exception (H-6, –85 μɛ). The internasal suture was less consistent, showing tension or compression in an equal number of pig heads, ranging from –210 to +230 μɛ.

Number | Right nasal bone rosette gauge | Right frontal bone rosette gauge | Load output (kg) | Suture single-element gauges (μɛ) | |||||||||||
---|---|---|---|---|---|---|---|---|---|---|---|---|---|---|---|
Principal strain (μɛ) | Max angle (°) | Principal strain (μɛ) | Max angle (°) | Right NFS loaded | Left NFS | Right CS loaded-side | Left CS | IFS | INS | ||||||
Max | Min | Shear | Max | Min | Shear | ||||||||||
H-1 | Indeterminate | Indeterminate | 1.55 | 1272 | 693 | −54 | −15 | 15 | 81 | ||||||
H-2 | 15 | −37 | 52 | 64 | 38 | −84 | 121 | 94 | 1.52 | 684 | 490 | 0 | −3 | 86 | 26 |
H-3 | Indeterminate | Indeterminate | 1.60 | 737 | 664 | −28 | −15 | 53 | 190 | ||||||
H-4 | Indeterminate | Indeterminate | 1.64 | 720 | 508 | −47 | −44 | 20 | −100 | ||||||
H-5 | 46 | −34 | 80 | 128 | Indeterminate | 1.64 | 1063 | 1045 | –80 | 0 | 213 | −192 | |||
H-6 | 27 | −46 | 73 | 57 | 26 | −55 | 80 | 111 | 1.37 | 914 | 558 | −75 | −27 | −85 | −17 |
Mean (SD) | 29 (15) | −39 (6) | 68 (14) | 83 (39) | 32 (8) | −69 (21) | 101 (29) | 103 (12) | 1.55 (0.10) | 898 (233) | 660 (205) | −47 (30) | −17 (16) | ∗ | ∗ |
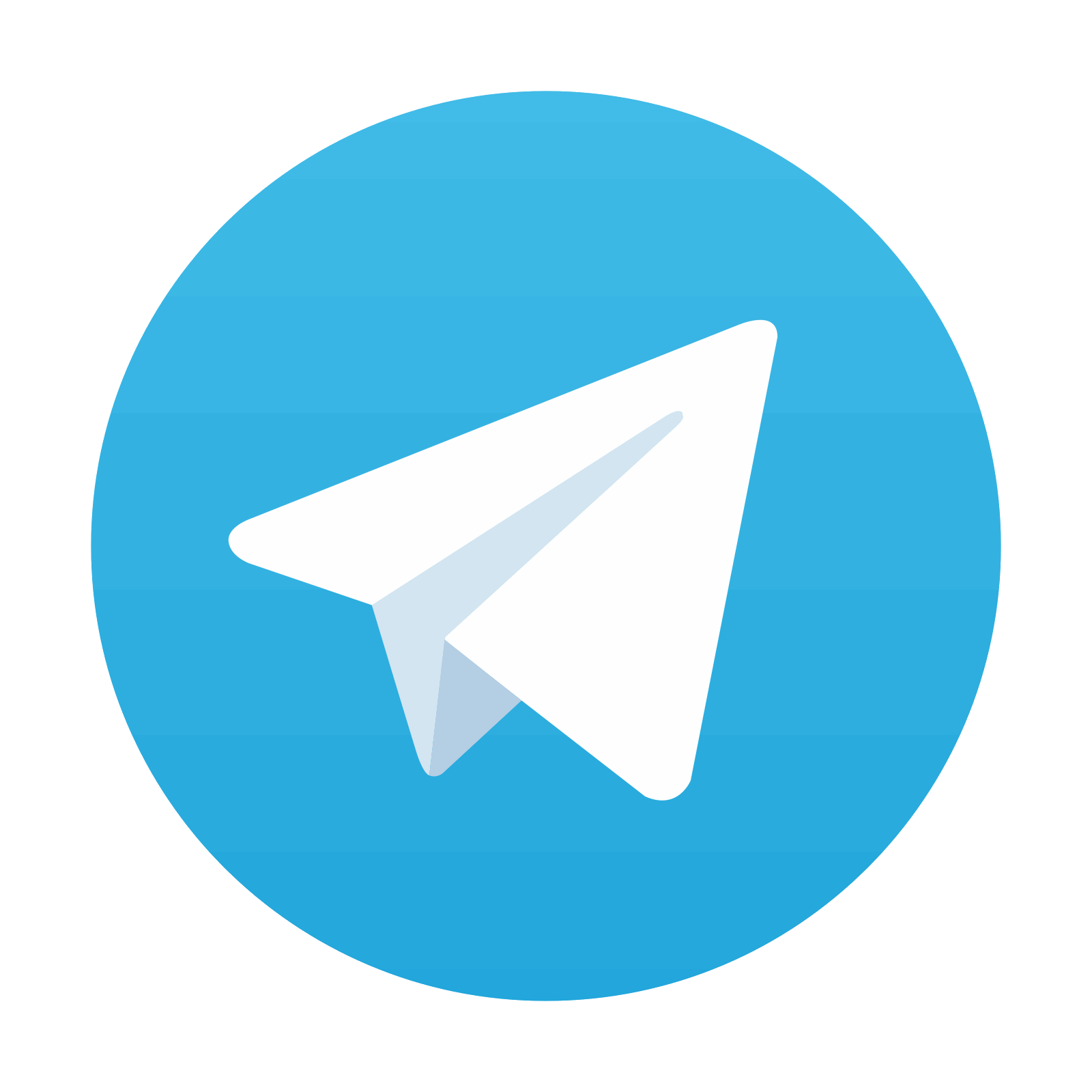
Stay updated, free dental videos. Join our Telegram channel

VIDEdental - Online dental courses
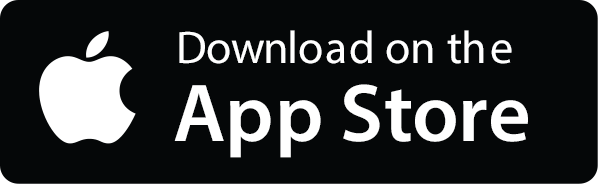
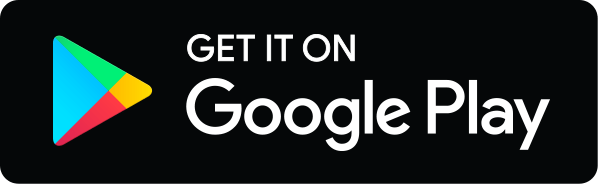
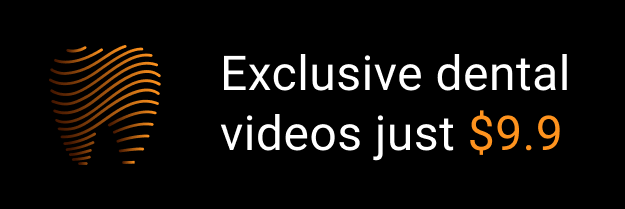