2: Current biomechanical rationale concerning composite attachments in aligner orthodontics
Juan Pablo Gomez Arango
Introduction
The orthodontic technique that we now call “aligner orthodontics” has evolved considerably over the last 20 years. Improvements in behavior of aligner plastics, treatment planning software, and three-dimensional (3D) printing have served one basic but fundamental intention: to mitigate the biomechanical limitations inherent to aligner-based tooth movement. Another significant development designed to overcome the aforementioned biomechanical shortcomings of aligner systems has been the continuous improvement of biomechanically complementary composite attachments. Attachments were conceived to produce supplementary force vectors that, when applied to teeth by the aligner material, transform the resultant system, allowing complex tooth movements. The application of one of the initial geometric configurations was initially presented by the clinical team from Align Technology Inc., as basic 1 x 3 mm rectangular structures, bonded to the lower incisor buccal surface, in an attempt at controlling undesired tipping during space closure after incisor extraction (Fig. 2.1A).1 As the incisors adjacent to the extraction space begin to incline mesially, the rigid, fixed structure of the attachment collides with aligner plastic, producing force couples that counteract the initial moment, reducing undesired tipping (see Fig. 2.1B).
Orthodontic tooth movement with conventional bracket techniques can deliver sophisticated force systems due to the manner in which the rigid ligature-archwire-bracket scheme “grasps” the malaligned tooth. This particular arrangement allows broad control of magnitude and direction of applied force vectors, and, consequentially, of tooth movement (Fig. 2.2).
It is important to keep in mind that attachments work, not as active agents that produce forces, but by passively “getting in the way” of plastic as it elastically deforms due to lack of coincidence between tooth position and aligner material (“mismatch”), establishing the force vector that subsequently affects the tooth (Fig. 2.3).
Biomaterials used for attachment fabrication must assure that requirements in adhesion, wear resistance, and esthetics are fulfilled. A recent study2 suggests that contemporary microfilled resin composites provide sufficient wear resistance to deliver a stable attachment shape during treatment, assuring its functionality. Mantovani et al.3 also concluded that the use of bulk-filled resins for attachment fabrication improved dimensional stability when compared to low-viscosity resins, which experience higher polymerization shrinkage. The use of translucent composites generally provides sufficient esthetic acceptance and stain resistance as long as an adequate bonding technique is executed, in which voids (bubbles) in attachment surface and excessive residue (flash) left on tooth surface4 are avoided.
Several considerations come into play when determining the optimal attachment design for a specific clinical objective: geometry, location, and size.
Geometry (active surface orientation)
At the time of aligner insertion, orthodontic forces will be produced in response to the particular complex pattern of mismatches between plastic and tooth structure. This pattern of mismatch–plastic deformation–orthodontic force is critical for attachment design during digital simulation to produce specific areas (active surfaces) that will contact aligner plastic with predetermined force magnitudes, producing the desired force vectors and consequent tooth movements. Not all the surface area of attachments will be in direct contact with the aligner. The active or functional surfaces can and should be determined with thoughtful biomechanical intentionality, in accordance with clinical objectives (Fig. 2.4A). While the magnitude of the force produced is determined by the amount of mismatch (along with the characteristics of aligner material), the direction of the force will depend on the orientation of the active surface. The principles of mechanics state that the direction of the normal component of the contact force (the vector that in this case acts upon the active surface of the attachment) will always be perpendicular to that surface (see Fig. 2.4B). Identifying the direction of these complementary force vectors is essential for treatment planning, especially when more than one force acts simultaneously. In these cases, the resultant forces must be properly recognized to deliver predictable tooth movements (see Fig. 2.4C).
Location
Based on the premise that the magnitude of a moment is proportional to the perpendicular distance between the line of action and the center of resistance, to fully understand the effect of aligner-based orthodontic forces being applied in any particular moment, it is essential to establish this distance in the three planes of space. Once this correlation has been clearly established and quantified, there will be a much clearer picture of the effectiveness of expected rotational moments as well as the possibility of anticipating undesired occurrences such as buccolingual and mesiodistal tipping and intrusion. In a case in which mesiolingual rotation of the tooth is required, localization of attachment A will produce a strong mesial tipping moment and a weak mesiolingual rotational moment (Fig. 2.5A). In this specific clinical situation, a better alternative would be with attachment location B, in which modification in distance from line of action to center of resistance would reduce tipping tendency as well as increase mesiolingual rotational capacity (see Fig. 2.5B).
Another example of the influence of attachment localization is observed during transverse arch expansion, when buccal tipping of posterior segments is detrimental to treatment objectives. A recent unpublished finite element analysis (FEA) study5 of the mechanical effects of the bonding position of rectangular horizontal attachments found that the resultant tipping moment acting on the molars was greater when located on the lingual surface of the first upper molars versus the labial surface (Fig. 2.6).
Size
Attachment size is important because of its mechanical and esthetic implications. Small configurations are desirable because they are less noticeable; however, as size diminishes, so does the ability to produce predictable forces due to reduced active surface area. On the other hand, larger attachment designs are desirable because of their increased biomechanical capabilities, but they result in increased aligner retention (with subsequent patient discomfort) and negative esthetic perception, especially with high-profile configurations in anterior teeth.
Functions
Providing aligner retention
For aligner-based orthodontic forces to affect teeth as conceived in digital simulation, the aligner must be stably seated after insertion and remain so for the duration of treatment. Occasionally, deficient adaptation of the aligner may occur, usually resulting from faulty fabrication, but may also occur due to the many reactive forces produced once properly fitted. For example, as a frequent response to intrusive forces acting on the posterior teeth, the aligner will tend to be dislodged in the anterior segment, and vice versa. The use of intermaxillary elastics, especially when they are engaged directly to the aligner, will also tend to vertically dislodge it in the direction of the elastic force. Bonding retentive attachments on teeth adjacent to those receptors of the elastic force is recommended to maintain proper aligner engagement (Fig. 2.7A). A study by Jones et al.6 suggests that the optimal attachment configuration, when high aligner retention is imperative, is a nongingivally beveled (such as a horizontal rectangular or occlusally beveled) design, as close to the gingival margin as possible (see Fig. 2.7B). As a general rule of attachment design, occlusal beveling will facilitate aligner insertion due to the inclined plane configuration as well as increase force (and discomfort) required for aligner removal.
Avoiding aligner “slipping”
Especially when rotating rounded teeth, the sum of a series of tangential forces is responsible for tooth movement (Fig. 2.8A), causing inconvenient displacement (slipping) of the aligner in relation to the tooth surface, reducing the system’s efficacy and predictability, and resulting in lack of full expression of digitally planned rotation with the tooth lagging behind the corresponding aligner stage. Clinically, incomplete rotation and loss of tracking will be observed, manifesting as a space between tooth and plastic (see Fig. 2.8B). Appropriately designed attachments can help the aligner lock in to the tooth crown, greatly reducing this undesired slipping effect.
Delivering predetermined force vectors
The fundamental purpose of composite attachments in aligner orthodontics is to produce specific, complementary force vectors required for predictable tooth movement, which are not possible with the sole use of aligners thermoformed with existing materials (Fig. 2.9A).
Unfortunately, to harness the full clinical potential of bonded attachments, current polymers have yet to resolve limitations associated with their viscoelastic and hygroscopic nature. Once inserted, the initial force produced by the aligner after it is elastically deformed is not constant and will decline with time. This time-dependent reduction of force under constant deformation is called stress relaxation.7,8 Not infrequently, due to unwarranted localized stress (caused by excessive mismatch), lack of compliance, or shortcomings inherent to the polymer, the aligner is not able to accommodate the attachment. When forces exerted upon the aligner exceed its capability to adjust to the new position, unintended forces will appear, the tooth will lag behind, and control will be lost (see Fig. 2.9B). Fig. 2.9 illustrates how this phenomenon is responsible for the incomplete expression of the expected tooth movement, where only 35 of the 45 degrees of predicted rotation were achieved after completion of the entire sequence of stages. In this case, after the aligner is removed, plastic deformation of the aligner material is evident. This time-dependent plastic deformation under constant force is called creep and is attributed to reorganization of polymer chains.9 It is important to underline that this permanent deformation, so detrimental to clinical performance of plastic aligners, is not caused by a violation of the materials’ elastic limit but is due to a time-dependent, mechanochemical phenomenon of a different nature.
This inherent flaw of aligner plastics is the major cause behind the inconsistent force levels and plastic deformation that result in one of the most dreaded occurrences for orthodontists practicing aligner orthodontics, now commonly referred to as loss of tracking. Fig. 2.10 illustrates an example of the clinical manifestations of this complex reality in which mesiolingual rotation and extrusion of a first upper left bicuspid were incorporated in the digital treatment plan but did not fully occur. The lack of coincidence between the attachment and its corresponding recess in the aligner is unambiguous evidence of loss of tracking, a contingency that in many cases must be resolved by obtaining updated digital dental models from which a new treatment sequence must be designed.
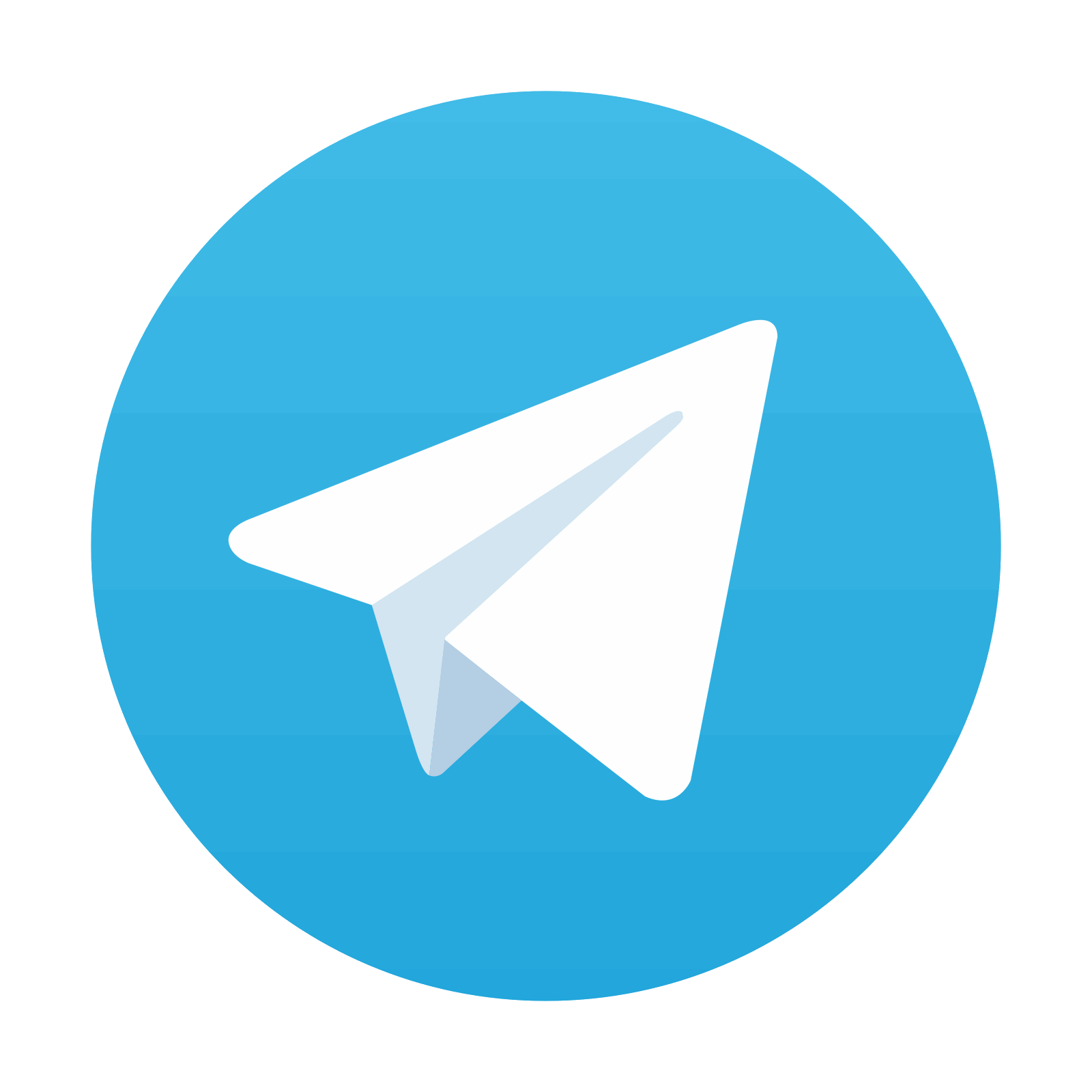
Stay updated, free dental videos. Join our Telegram channel

VIDEdental - Online dental courses
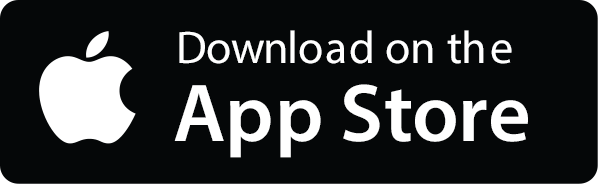
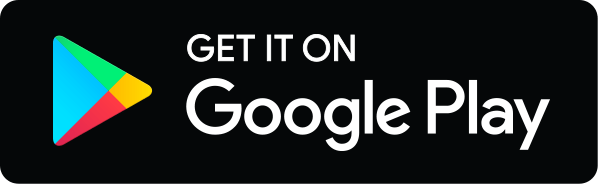