Graphical abstract
Highlights
- •
MDP can establish a chemical bond with zirconia and is influenced by the pH at the Y-TZP surface.
Abstract
Objectives
Identification of the mechanism of chemical coupling of a phosphate ester monomer to zirconia via a computational modeling approach enables materials scientists to design new coupling agents that can resist hydrolytic degradation of bonds made by methacrylate resins to zirconia. We investigated the possibility of chemical bonding between 10-methacryloyloxydecyldihydrogenphosphate (MDP) and tetragonal zirconia, and the effect of pH reaction conditions on such prospective chemical bonds.
Methods
A tetragonal zirconia crystal model was created. An “Our-own N-layered integrated molecular orbital and molecular mechanics” (ONIOM) method was used to simulate two potential configurations of the MDP–ZrO 2 system: double-coordinate and single-coordinate. Thermodynamic calculations were used to ascertain if the reaction could proceed spontaneously and to compare the stability of the two possible configurations. Short-term testing of shear bond strength (SBS) was done to evaluate bonding improvement of MDP to alumina-sandblasted zirconia surfaces in neutral, acidic or alkaline environments.
Results
Digital models of coordinate bonds between MDP and tetragonal zirconia were constructed. Thermodynamic calculations indicated that the Gibbs free energy for forming double-coordinate and single-coordinate configurations were −461.2 kJ/mol and −450.9 kJ/mol, respectively. Equilibrium constants for the double-coordinate and single-coordinate configurations were 6.4 × 10 80 and 9.8 × 10 78 , respectively. Application of MDP in alkaline conditions showed the highest SBS, whereas acid conditions resulted in lower SBS.
Significance
MDP can establish a “true” chemical bond with zirconia spontaneously. The double-coordinate configuration was identified to be more energetically favorable than the single-coordinate configuration for the coupling of MDP to zirconia. Alkaline conditions may positively affect formation of MDP–ZrO 2 coordination bonds.
1
Introduction
Yttria-stabilized tetragonal zirconia polycrystals (Y-TZP) have attracted appreciable attention in dental restorations and implants because of their fracture resistance and esthetic properties . A strong bond between the dental structure and ceramic is critical for optimal clinical performance of all-ceramic restorations. The clinical protocol most frequently used for luting silica-based ceramic restorations involves etching with hydrofluoric acid followed by silanization . Due to the non-polar nature and inertness of zirconia, neither acid etching nor formation of a siloxane network between silane and the substrate can be achieved in Y-TZP . Thus, alternative strategies for surface treatment to improve the bonding of Y-TZP to resin materials have been developed. These strategies are based predominantly on micromechanical interlocking, and primer conditioning for improving chemical bonding. Examples of these strategies include alumina sandblasting, tribochemical silica coating, selective infiltration etching, laser etching, glazing, and application of acidic adhesive monomers . Among these strategies, use of a 10-methacryloyloxydecyldihydrogenphosphate (MDP)-containing primer for conditioning surface-roughened Y-TZP is rapidly gaining acceptance among clinicians .
The MDP monomer was developed to achieve bifunctional adhesion between metal/metallic oxide and bisphenol-A diglycidyl dimethacrylate (bis-GMA)-containing resin matrices . Chemical conditioning with MDP aims to create a “reactive” Y-TZP surface that facilitates chemical bonding between phosphate groups in the MDP and oxide groups on the Y-TZP surface. Explanation of the exact mechanism of this chemical reaction is extremely beneficial for designing more and better novel phosphate monomers. Hence, scholars have employed different analytical methods to examine what occurs between MDP and zirconia. These methods, such as X-ray photoelectron spectroscopy (XPS), Fourier-transform infrared spectroscopy (FTIR), and Raman spectroscopy, aim to characterize the reaction between MDP and Y-TZP . Unfortunately, they can reflect only the possible changes of the hydroxyl group in the phosphate functional groups during this reaction, rather than identify the exact newly formed chemical bonds. It was not until 2012 that a –P–O–Zr bond on the Y-TZP surface conditioned with a MDP-containing zirconia primer (Z-Prime Plus; Bisco, Schaumburg, IL, USA) was detected by time-of-flight secondary ion mass spectrometry . Detection of the chemical bonds between MDP and zirconia is difficult, so the strength of the evidence provided by only one study is not robust. Does this reaction actually occur, or is the alleged “–P–O–Zr” bond detected only by chance? There are insufficient data about the actual mechanism of reaction of MDP and whether it establishes a “true” chemical bond with zirconia. Furthermore, if the reaction between MDP and zirconia occurs spontaneously then, during this reaction, H + ions dissociate from the phosphate groups of MDP molecules and coordinate with oxygen atoms in zirconia. Therefore, increasing numbers of OH − ions in the reaction environment would promote dissociation of H + ions, which would lead to strengthening of the chemical bonds between MDP and zirconium oxide. Conversely, increasing numbers of H + ions would lead to the opposite effect. These theoretical predictions suggest that conditioning a Y-TZP bonding surface to alkaline pH before application of a MDP-containing primer would promote bonding improvements between MDP and zirconia. If such predictions are correct, it would be useful information for clinical application of MDP.
To clarify the issues mentioned above, we designed a series of tests to identify the possibility of a reaction between MDP and zirconia through computational chemistry. Then, a short-term shear bond strength (SBS) test was carried out to address the theoretical prediction that environmental pH can influence the resin bond strength of MDP-conditioned Y-TZP. The null hypothesis was: (i) MDP can establish a true chemical bond with Y-TZP; (ii) improvement in the bonding between MDP and Y-TZP is not influenced by the pH of the Y-TZP surface.
2
Materials and methods
2.1
Modeling of a MDP–ZrO 2 system
A tetragonal zirconia crystal model was created using Materials Studio v4.0 (Accelrys, San Diego, CA, USA) according to the Inorganic Crystal Structure Database (Fachinformationszentrum Karlsruhe Information Services, Eggenstein-Leopoldshafen, Germany). After building the crystal model, faces with low indices were cleaved to evaluate the atomic arrangement, as well as the possible interaction sites, between the MDP molecules with those crystalline faces . To build a viable zirconia cluster for subsequent calculations, a small, neutral repetitive unit in a tetragonal unit cell was cleaved from the tetragonal zirconia crystal model. The “ideal” ZrO 2 cluster possessed the following characteristics: (i) an electrically neutral ZrO 2 cluster; (ii) a cluster size appropriate for high-level ab initio calculation; (iii) the structure of the ZrO 2 cluster did not change considerably after geometric optimization using revised Gaussian 09 software (Gaussian, Wallingford, CT, USA).
Phosphate groups in the MDP molecule can react with one or two zirconium atoms in zirconia crystals. Therefore, theoretically two bonding configurations are possible: “double coordinate” and “single coordinate” ( Fig. 1 A ). These two theoretically possible bonding configurations between the phosphate group of the MDP molecule and zirconium atoms on the zirconia surface were modeled for the MDP–ZrO 2 system.
2.2
Calculations
Interactions between MDP and Zr 4 O 8 clusters were analyzed by thermodynamic calculations to examine the stability of the two MDP–ZrO 2 bonding configurations. Calculations were undertaken using the ONIOM method . All calculations relating to geometric optimization and calculation of thermodynamic functions were undertaken using Gaussian 09 software. All data were calculated at standard conditions (1 atmosphere, 298 K).
2.3
Shear bond testing
A total of 140 Y-TZP blocks (10 × 10 × 2 mm 3 ) were sectioned from a machinable Y-TZP block (KaVo Everest ® , ZS-Ronde; Kaltenbach and Voigt GmbH and Co., Bismarcking, Germany) using a low-speed saw (Isomet 100; Buehler Ltd., Lake Bluff, IL, USA) under copious water cooling. The Y-TZP block was sintered completely according to manufacturer instructions. Y-TZP plates were assigned randomly to seven groups ( N = 20) according to the method of surface conditioning, as detailed below.
For group C (control group), the bonding area of Y-TZP was sandblasted with 110-μm alumina particles from 10 mm for 20 s at 0.3 MPa using a sandblasting device (Lndp-II; Jianian Futong Medical Equipment Co., Ltd., Tianjin, China).
After alumina sandblasting, Z-prime Plus (CZ; Bisco Inc., IL, USA) or Monobond Plus (CM; Ivoclar Vivadent, Schaan, Liechtenstein) were applied to the surface of Y-TZP and dried with oil-free air after 5–10 s of volatilization.
After alumina sandblasting, a solution of sulfuric acid (pH = 2–3) was applied to the surface of Y-TZP for 10 min, dried with oil-free air, and conditioned with Z-prime Plus (ZH) or Monobond Plus (MH).
After alumina sandblasting, a solution of sodium hydroxide (pH = 11–12) was applied to the surface of Y-TZP for 10 min, dried with oil-free air, and conditioned with Z-prime Plus (ZOH) or Monobond Plus (MOH).
A polymerized resin composite (Valux Plus; 3 M ESPE, Maplewood, MN, USA) cylinder (inner diameter, 6 mm; height, 3 mm) was cemented to each conditioned Y-TZP block with a resin composite cement (Variolink N; Ivoclar Vivadent). The cement was light-cured for 40 s (Elipar FreeLight 2; 3 M ESPE). Bonded specimens were subjected to SBS testing using a universal testing machine (Model 3365; Instron Corp., Canton, MA, USA) at a crosshead speed of 1 mm/min. Testing was undertaken after 24 h or 30 days of water storage at room temperature
2.4
Statistical analyses
SBS data were subjected to two-way analysis of variance (ANOVA) and least square difference (LSD) multiple comparison tests to compare the effects of the two categorical independent parameters (surface-conditioning methods and water storage time) on SBS. Post hoc analyses were undertaken using the Tukey test to compare mean values of SBS between individual groups with 24 h or 30 days of water storage. Statistical analyses were carried out using SPSS v19.0 (IBM, Armonk, NY, USA). Statistical significance was preset at p = 0.05.
2.5
Characterization of Y-TZP surfaces with or without acid and alkaline pre-treatments
pH values of Y-TZP powders with or without pre-treatments of solutions of sulfuric acid (pH = 2–3) and sodium hydroxide (pH = 11–12) were determined by a precision pH meter (PHS-3C; Shanghai Yice Electronic Equipment Ltd., Shanghai, China). Before examination, pre-treated Y-TZP powders were dried under vacuum, immersed in deionized water, and stirred for 10 min.
Y-TZP powders with or without acid and alkaline pre-treatments were dried at 80 °C under vacuum for 12 h to exclude the interference of water. Then, they were characterized by FTIR (NEXUS 870; Thermo Nicolet™, Waltham, MA, USA) in transmission mode.
Alumina-sandblasted Y-TZP surfaces with or without acid and alkaline pre-treatments were sputter-coated with gold and examined with a field emission scanning electron microscope (FE-SEM; SU8180; Hitachi, Tokyo, Japan) at 20 kV.
2
Materials and methods
2.1
Modeling of a MDP–ZrO 2 system
A tetragonal zirconia crystal model was created using Materials Studio v4.0 (Accelrys, San Diego, CA, USA) according to the Inorganic Crystal Structure Database (Fachinformationszentrum Karlsruhe Information Services, Eggenstein-Leopoldshafen, Germany). After building the crystal model, faces with low indices were cleaved to evaluate the atomic arrangement, as well as the possible interaction sites, between the MDP molecules with those crystalline faces . To build a viable zirconia cluster for subsequent calculations, a small, neutral repetitive unit in a tetragonal unit cell was cleaved from the tetragonal zirconia crystal model. The “ideal” ZrO 2 cluster possessed the following characteristics: (i) an electrically neutral ZrO 2 cluster; (ii) a cluster size appropriate for high-level ab initio calculation; (iii) the structure of the ZrO 2 cluster did not change considerably after geometric optimization using revised Gaussian 09 software (Gaussian, Wallingford, CT, USA).
Phosphate groups in the MDP molecule can react with one or two zirconium atoms in zirconia crystals. Therefore, theoretically two bonding configurations are possible: “double coordinate” and “single coordinate” ( Fig. 1 A ). These two theoretically possible bonding configurations between the phosphate group of the MDP molecule and zirconium atoms on the zirconia surface were modeled for the MDP–ZrO 2 system.
2.2
Calculations
Interactions between MDP and Zr 4 O 8 clusters were analyzed by thermodynamic calculations to examine the stability of the two MDP–ZrO 2 bonding configurations. Calculations were undertaken using the ONIOM method . All calculations relating to geometric optimization and calculation of thermodynamic functions were undertaken using Gaussian 09 software. All data were calculated at standard conditions (1 atmosphere, 298 K).
2.3
Shear bond testing
A total of 140 Y-TZP blocks (10 × 10 × 2 mm 3 ) were sectioned from a machinable Y-TZP block (KaVo Everest ® , ZS-Ronde; Kaltenbach and Voigt GmbH and Co., Bismarcking, Germany) using a low-speed saw (Isomet 100; Buehler Ltd., Lake Bluff, IL, USA) under copious water cooling. The Y-TZP block was sintered completely according to manufacturer instructions. Y-TZP plates were assigned randomly to seven groups ( N = 20) according to the method of surface conditioning, as detailed below.
For group C (control group), the bonding area of Y-TZP was sandblasted with 110-μm alumina particles from 10 mm for 20 s at 0.3 MPa using a sandblasting device (Lndp-II; Jianian Futong Medical Equipment Co., Ltd., Tianjin, China).
After alumina sandblasting, Z-prime Plus (CZ; Bisco Inc., IL, USA) or Monobond Plus (CM; Ivoclar Vivadent, Schaan, Liechtenstein) were applied to the surface of Y-TZP and dried with oil-free air after 5–10 s of volatilization.
After alumina sandblasting, a solution of sulfuric acid (pH = 2–3) was applied to the surface of Y-TZP for 10 min, dried with oil-free air, and conditioned with Z-prime Plus (ZH) or Monobond Plus (MH).
After alumina sandblasting, a solution of sodium hydroxide (pH = 11–12) was applied to the surface of Y-TZP for 10 min, dried with oil-free air, and conditioned with Z-prime Plus (ZOH) or Monobond Plus (MOH).
A polymerized resin composite (Valux Plus; 3 M ESPE, Maplewood, MN, USA) cylinder (inner diameter, 6 mm; height, 3 mm) was cemented to each conditioned Y-TZP block with a resin composite cement (Variolink N; Ivoclar Vivadent). The cement was light-cured for 40 s (Elipar FreeLight 2; 3 M ESPE). Bonded specimens were subjected to SBS testing using a universal testing machine (Model 3365; Instron Corp., Canton, MA, USA) at a crosshead speed of 1 mm/min. Testing was undertaken after 24 h or 30 days of water storage at room temperature
2.4
Statistical analyses
SBS data were subjected to two-way analysis of variance (ANOVA) and least square difference (LSD) multiple comparison tests to compare the effects of the two categorical independent parameters (surface-conditioning methods and water storage time) on SBS. Post hoc analyses were undertaken using the Tukey test to compare mean values of SBS between individual groups with 24 h or 30 days of water storage. Statistical analyses were carried out using SPSS v19.0 (IBM, Armonk, NY, USA). Statistical significance was preset at p = 0.05.
2.5
Characterization of Y-TZP surfaces with or without acid and alkaline pre-treatments
pH values of Y-TZP powders with or without pre-treatments of solutions of sulfuric acid (pH = 2–3) and sodium hydroxide (pH = 11–12) were determined by a precision pH meter (PHS-3C; Shanghai Yice Electronic Equipment Ltd., Shanghai, China). Before examination, pre-treated Y-TZP powders were dried under vacuum, immersed in deionized water, and stirred for 10 min.
Y-TZP powders with or without acid and alkaline pre-treatments were dried at 80 °C under vacuum for 12 h to exclude the interference of water. Then, they were characterized by FTIR (NEXUS 870; Thermo Nicolet™, Waltham, MA, USA) in transmission mode.
Alumina-sandblasted Y-TZP surfaces with or without acid and alkaline pre-treatments were sputter-coated with gold and examined with a field emission scanning electron microscope (FE-SEM; SU8180; Hitachi, Tokyo, Japan) at 20 kV.
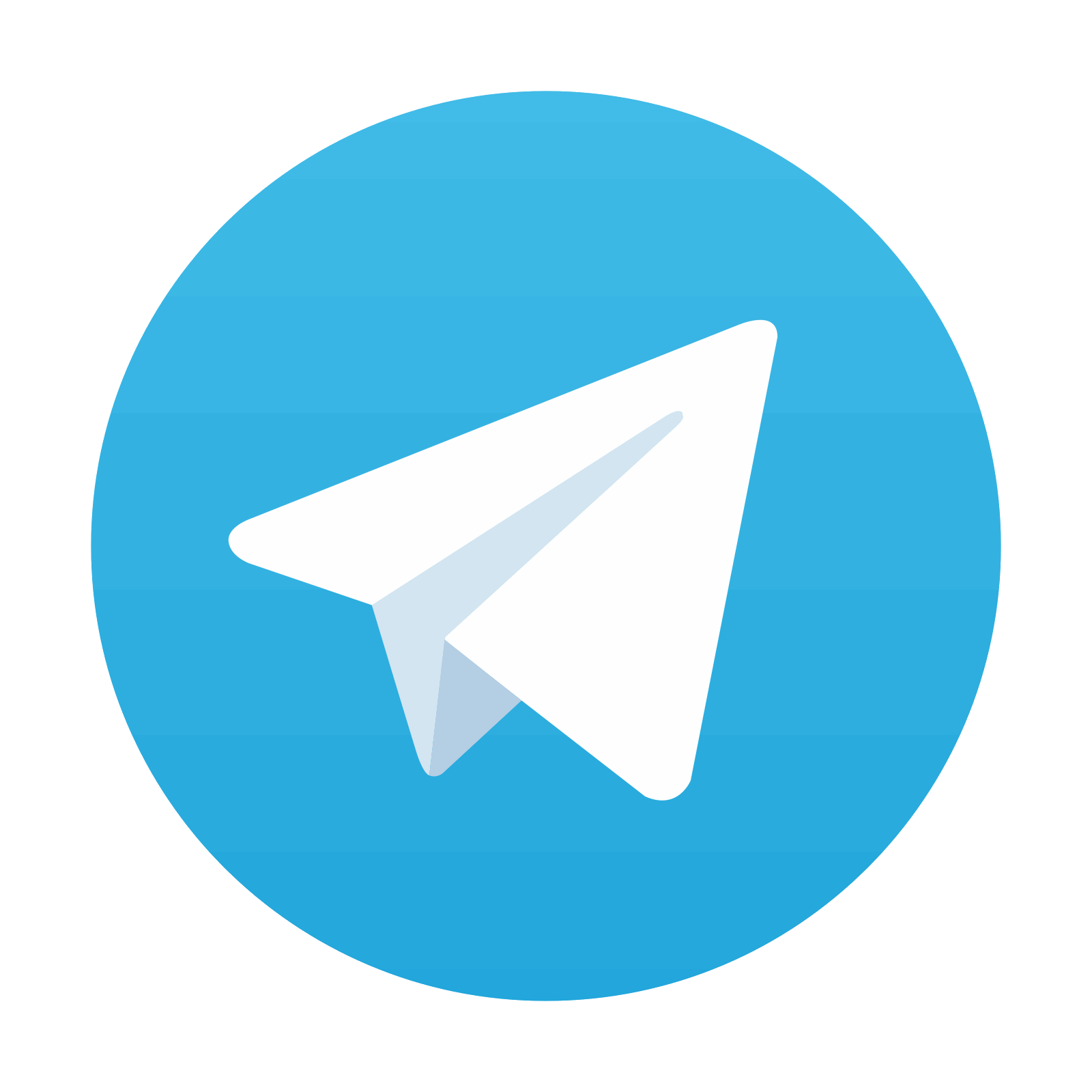
Stay updated, free dental videos. Join our Telegram channel

VIDEdental - Online dental courses
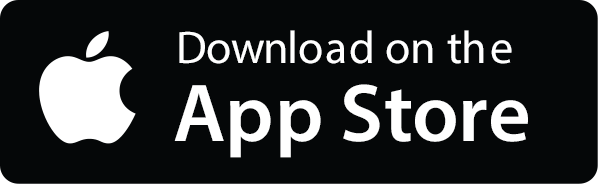
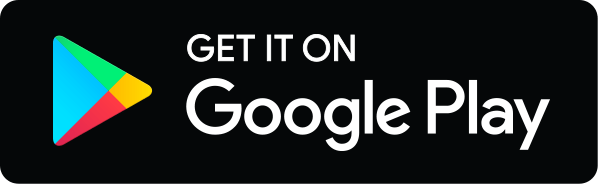