Abstract
Objectives
Cure, volumetric changes and mechanical properties were assessed for new dental composites containing chlorhexidine (CHX) and reactive calcium phosphate-containing (CaP) to reduce recurrent caries.
Methods
20 wt.% of light curable urethane dimethacrylate based liquid was mixed with 80 wt.% glass filler containing 10 wt.% CHX and 0–40 wt.% CaP. Conversion versus depth with 20 or 40 s light exposure was assessed by FTIR. Solidification depth and polymerization shrinkage were determined using ISO 4049 and 17304, respectively. Subsequent volume expansion and biaxial flexural strength and modulus change upon water immersion were determined over 4 weeks. Hydroxyapatite precipitation in simulated body fluid was assessed at 1 week.
Results
Conversion decreased linearly with both depth and CaP content. Average solidification depths were 4.5, 3.9, 3.3, 2.9 and 5.0 with 0, 10, 20, and 40% CaP and a commercial composite, Z250, respectively. Conversions at these depths were 53 ± 2% for experimental materials but with Z250 only 32%. With Z250 more than 50% conversion was achieved only below 1.1 mm. Shrinkage was 3% and 2.5% for experimental materials and Z250, respectively. Early water sorption increased linearly, whilst strength and modulus decreased exponentially to final values when plotted versus square root of time. Maximum volumetric expansion increased linearly with CaP rise and balanced shrinkage at 10–20 wt.% CaP. Strength and modulus for Z250 decreased from 191 to 158 MPa and 3.2 to 2.5 GPa. Experimental composites initial strength and modulus decreased linearly from 169 to 139 MPa and 5.8 to 3.8 GPa with increasing CaP. Extrapolated final values decreased from 156 to 84 MPa and 4.1 to 1.7 GPa. All materials containing CaP promoted hydroxyapatite precipitation.
Significance
The lower surface of composite restorations should both be solid and have greater than 50% conversion. The results, therefore, suggest the experimental composite may be placed in much thicker layers than Z250 and have reduced unbounded cytotoxic monomer. Experimental materials with 10–20 wt.% additionally have volumetric expansion to compensate shrinkage, antibacterial and re-mineralizing components and competitive mechanical properties.
1
Introduction
Dental caries is caused by bacteria producing acid that then dissolves the hydroxyapatite mineral in the tooth. In order to minimize disease progression, restore function and allow effective biofilm removal, infected tissue is excavated and replaced with a filling material. Following the recent signing and future multinational ratification of the Minamata agreement on mercury, phasing out of amalgam fillings will become likely. Dental composites will then be the main direct tooth restorative material. Unfortunately composites generally require replacing more often and are technically more difficult to place .
Dental composites consist of filled light curable dimethacrylate monomers. Their failure can be via fracture due to low strength but is more commonly nowadays caused by recurrent caries following composite debonding from the tooth structures. This can result from lack of composite antibacterial action and shrinkage during setting. Resultant micro-gap formation allows bacterial penetration, secondary caries and continuing hydroxyapatite dissolution beneath the composite restoration .
Many studies have included amorphous calcium phosphate or brushite (dicalcium phosphate dihydrate) particles in dental composite formulations. The aim was to promote surrounding dentin remineralization . The low solubility of these calcium phosphates however, could potentially limit this benefit. More recently, mixtures of reactive hydrophilic and hydrophobic calcium phosphates were included in antibacterial chlorhexidine-releasing composites . Hydrophilic, water soluble MCPM enhanced rapid water sorption induced swelling which promotes expansion to compensate polymerization shrinkage and effective antibacterial chlorhexidine release. Additionally, surface MCPM readily dissolved. This could potentially supersaturate dentinal fluid and force hydroxyapatite precipitation. In the composite bulk, however, MCPM reacted with βTCP to form less soluble brushite crystals. This reaction can help bind absorbed water. Unbound water would otherwise reduce long-term strength through hydrolysing glass silane surfaces or plasticizing the polymer phase .
Previous studies using reactive calcium phosphates and chlorhexidine in composites as the only fillers resulted in excessive expansion and decline in strength . Combining more conventional fillers with reactive calcium phosphates partially overcame these issues. Lack of refractive index matching, however, meant formulations had to be cured through mixing of alternative chemical cure initiators with an activator instead of by light exposure . Furthermore, neither of these studies considered cure variation with depth, long term strength reduction or modulus.
High degree of monomer conversion is essential for optimal physical properties and to reduce cytotoxic risks but it does increase polymerization shrinkage . High light penetration depth is also required for placement in deeper fillings. The following new study aim was therefore to compare conversion versus depth, volumetric changes and mechanical properties of new light curable composites with reactive calcium phosphates and chlorhexidine. Volumetric studies included both polymerization shrinkage and subsequent expansion that occurs due to water sorption. Re-mineralizing properties have been assessed using a method more commonly employed to investigate bone cement.
2
Materials and methods
In the following, urethane dimethacrylate (UDMA) was used as the base monomer with triethylene glycol dimethacrylate (TEGDMA) and hydroxyethyl methacrylate (HEMA) as diluents. Relative levels were pre-optimized to allow high powder content and low shrinkage whilst ensuring rheological characteristics comparable with commercial composites. Camphorquinone (CQ) and dimethylparatoluidine (DMPT) were employed as initiator and activator, respectively. The base glass was chosen due to its close refractive index (0.52) match with the monomer (0.48) to enable good depth of cure. It additionally had high radiopacity due to barium (ratio of Al:Si:Ba oxides of 1:9.5:3 by XRF) to enable detection in dental X-rays. Furthermore, the particle size range (1 to 20 micron diameter by SEM) enabled high filler content. Chlorhexidine and glass fibers levels were fixed but reactive calcium phosphate levels were varied.
2.1
Composite paste preparation
Dental pastes were prepared by combining liquid and powder phases at a powder: liquid ratio of 4:1. The light curable liquid phase consisted of UDMA, TEGDMA and HEMA (all from Esstech) with CQ and DMPT (both from Sigma–Aldrich) in the weight ratio of 68:25:5:1:1. The base powder phase was a silane treated barium boro alumino silicate glass (IF 2019 from Sci-pharm). This component contributed 30, 50, 60 or 70 wt.% of the filler. Additional components were borosilicate glass fibers (MO-SCI) and Chlorhexidine diacetate salt hydrate (CHX) (Sigma–Aldrich) fixed at 20 and 10 wt.% of the total filler, respectively. Furthermore, equal masses of β-tricalcium phosphate (Plasma Biotal) and monocalcium phosphate monohydrate (Himed) were included. The combined reactive calcium phosphate (CaP) content was 0, 10, 20 or 40 wt.% of the total filler. All components of the powder phase were weighed and mixed on a mixing pad before adding the required amount of monomer to form a paste.
2.2
Monomer conversion
To quantify monomer conversion, composite pastes were placed in metal rings (10 mm internal diameter and 1 mm thick) on the centre diamond of a golden gate ATR top-plate (Specac Ltd., UK) in a Fourier transform infrared spectrometer (FTIR) (Perkin Elmer Series 2000, UK). Up to 4 rings were stacked on top of each other to gain samples of 1, 2, 3 and 4 mm depth. The temperature was kept at 37 °C using a 3000 Series RS232 high stability temperature controller (Specac Ltd., UK). The top surface of the sample was covered with acetate sheet to prevent oxygen inhibition of the polymerization process . FTIR spectra of the lower surface of the specimen in contact with the diamond were recorded with a resolution of 4 cm −1 for 20 min from 700 to 2000 cm −1 . After 1 min, the top surface was exposed to blue light (Demi Plus, Kerr) for 20 or 40 s. Fractional monomer conversion at 1 to 4 mm depth was calculated from the initial and final peak height at 1320 cm −1 above background at 1347 cm −1 .
2.3
Depth of cure (ISO 4049:2009)
To assess depth of cure using the ISO 4049 method, pastes were condensed into metal moulds (4 mm diameter and 6 mm deep) ( n = 3). After exposure of the top surface to blue light for 20 s or 40 s, the specimen was removed from the mould and any soft material scraped away using a plastic spatula. The depth of the remaining solid specimen, h , was measured. The depth of cure is presented as half of h according to the ISO standard.
2.4
Polymerization shrinkage
2.4.1
Calculated shrinkage
One mole of polymerizing C C bonds typically gives volumetric shrinkage of 23 cm 3 /mol . Total fractional shrinkage, φ , due to the composite polymerization can therefore be estimated from FTIR monomer conversions, using
φ = 23 C ρ ∑ i n i x i W i
where C , monomer conversion (%); ρ , composite density (g/cm 3 ); n i , the number of C C bonds per molecule; w i , molecular weight (g/mol) of each monomer; x i , mass fraction of each monomer.
2.4.2
Experimental shrinkage
Polymerization shrinkage was also determined using Archimedes’ principle and ISO 17304:2013. This method determines the densities of unpolymerized paste and polymerized discs by measuring their mass in air and water. An analytic balance (AG 204 Mettler Toledo) equipped with a density kit was used.
2.5
Mass and volume change
For composite density, mass change and volume change determination, pastes were placed in metal rings (1 mm deep and 10 mm internal diameter), covered top and bottom with acetate sheet and light cured for 40 s top and bottom to ensure maximum polymerization of the whole disc. Before testing, the resultant composite discs were removed from the moulds and their edges polished to remove any loose chips. Discs were stored in separate sterilin tubes each containing 10 mL of distilled water at 37 ̊C. After 0,1, 2, 4, 6 h and 1, 2, 4 days and 1, 2, 4 and 6 weeks, specimens mass, volume and density were determined using a density kit and four-figure digital balance as above.
2.6
Biaxial flexural strength and modulus
To assess strength and modulus variation with time, 1 mm thick and 10 mm diameter specimens were prepared as above and stored either dry or for 1 day, 1 week or 1 month in distilled water. Biaxial flexural strength, S , and modulus, E , were determined using a “ball on ring” jig and a universal testing machine (Instron 4502, U.K.) with the following equations:
S = P h 2 1 + μ 0.485 ln a h + 0.52 + 0.48
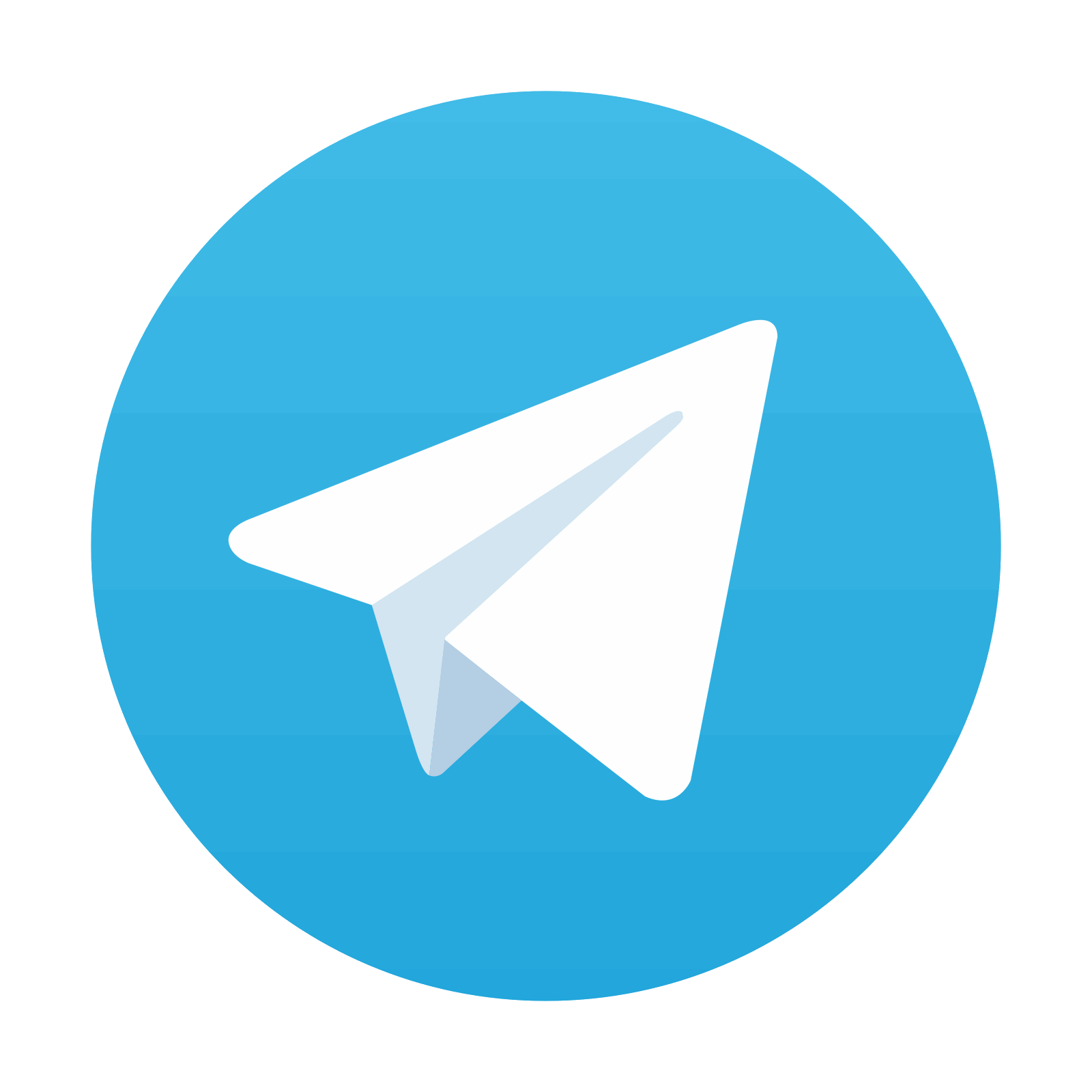
Stay updated, free dental videos. Join our Telegram channel

VIDEdental - Online dental courses
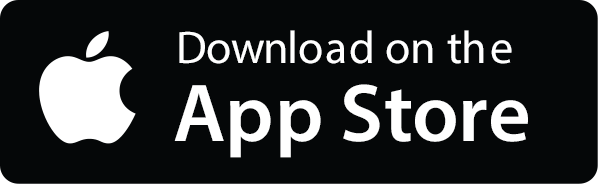
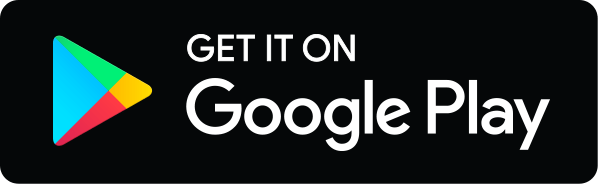