Abstract
The goal of orbital reconstruction is to repair trauma defects, to correct the position of the eye anatomically, avoiding enophthalmos, and to restore ocular function. For the reconstruction of (trauma) defects, many surgeons recommend materials that can be bent into an anatomical shape and that possess the properties of radiopacity and long-term stability. However, apart from these desired properties, the ideal material for orbital reconstruction remains controversial. Autologous bone is often mentioned as the ‘gold standard,’ likely because of its mechanical properties, revascularization potential, and its adaptation to the orbital tissue with minimal acute and chronic immune reactivity. However, autologous bone can show unpredictable resorption rates and suboptimal volume correction. In recent years, an increasing interest in the use of alloplasts for orbital reconstruction has become apparent in the literature. Modern technological advantages, such as preoperative planning, navigation, and perioperative imaging, can be beneficial in the decision to choose a certain implant. The aim of this review is to give a comprehensive overview of the advantages and disadvantages of materials used to reconstruct traumatic orbital defects and to provide a practical, evidence-based, complexity-driven set of guidelines.
In the reconstruction of orbital fractures, the purpose of an implant in orbital wall reconstruction is to restore function and aesthetic appearance by repairing the traumatic defect and bringing the globe into its correct position, thereby avoiding enophthalmos. In addition to the timing and methods of reconstruction, as described in previous reviews, a third pivotal factor in orbital fracture surgery is the choice of reconstruction material. Numerous studies describing orbital fracture repair with a variety of materials that offer various advantages and disadvantages have been reported in the literature. For many decades, biological transplants derived from human or animal tissues, polymers, and metals have been used. With the development of biocompatible alloplastic implants, new options (polymers, biological ceramics, and composites) have been added to the surgeon’s armamentarium ( Table 1 ). Within this context, controversy exists regarding the best material features, which can be defined broadly by the following parameters: (1) autogenous versus allogeneic, (2) non-resorbable versus resorbable material, (3) malleable versus preformed anatomical plates, and (4) pre-fabricated versus custom-made implants.
Biological materials |
Autografts/autogenous materials |
Autologous bone |
Calvarium; iliac crest; rib, anterior sinus maxillary wall; mandibular symphysis |
Maxillary sinus wall |
Autologous cartilage |
Nasal septum or concha; auricle; rib |
Autologous fascia |
Tensor fascia lata; temporal fascia |
Autologous periosteum |
Allografts |
Lyophilized dura mater; demineralized human bone; lyophilized cartilage; irradiated fascia lata |
Xenografts and animal-derived materials |
Collagen membrane; porcine sclera; porcine skin gelatin/Gelfilm; bovine bone or sclera |
Biological ceramics (inorganic, non-metallic) |
Porous hydroxyapatite (HA) and other calcium phosphates |
Bioactive glasses (BAG) |
Metals |
Titanium |
Cobalt alloys |
Polymers (plastics) |
Non-porous non-resorbable (permanent) implants |
Silicone; nylon (SupraFOIL; Supramid); polytetrafluoroethylene (PTFE; Teflon, Gore-Tex); hydrogels, PEEK, PEKK |
Non-porous resorbable implants |
Hyaluronate/carboxymethylcellulose (HA/CMC; Seprafilm) |
Porous non-resorbable implants |
Porous polyethylene (PE; Medpor) |
Porous resorbable (absorbable) implants |
Poly(lactic acid) (PLA); poly(glycolic acid) (PGA); PLA/PGA implants |
Polydioxanone (PDS); polyglactin 910/PDS implants (Ethisorb) |
Composites |
HA-reinforced high density composite (HAPEX) |
Titanium/PE composite implant (Medpor Titan) |
HA/PLLA/polycaprolactone (PCL) sheet |
Bone morphogenetic protein-loaded gelatin hydrogel |
PLA-based polymer sheet |
Periosteum/polymer complex |
Gelatin hydrogel (dogs) |
HA nanoparticles/cyclic acetal hydrogels |
Bone marrow-coated PCL scaffolds (pigs) |
The indication for repair of orbital wall fractures is based on a combination of clinical findings and radiological information. However, among 55 studies performed on orbital reconstruction, it was found that the indication for surgery was based on diplopia in only 18.3% of cases and on preoperative enophthalmos in only 29.8% of cases. The other two most frequently reported indications for orbital reconstruction are defect size (<50% of surface) and incarcerated tissue, with both identified on computed tomography (CT) scans. If an indication for surgery is present, the next dilemma is the selection of the correct implant. This choice could be based on an algorithm for the defect size, the anatomical location, or the remaining structural support. Small defects may heal solely by the formation of scar tissue, whereas larger defects, especially those associated with enophthalmos and hypoglobus, need material of a sufficient strength to support the orbital contents and restore the contour of the orbit.
In defining the ideal characteristics of an orbital implant, many surgeons prefer materials that (1) allow bending to an anatomical shape, (2) are radiopaque, and (3) remain stable over time. The key question is what specific characteristics orbital implants should have to be beneficial for the different types of orbital fracture. While an increasing body of evidence is pointing to the importance of differentiated and complexity-based treatment models in general trauma surgery, this approach seems to fail for orbital fractures. For smaller defects (types I and II), the strength of the reconstruction material holds limited relevance for a successful outcome. Rather, the choice of material is more dependent on biocompatibility. In larger fractures (types III and IV), mechanical properties and the contour or form factor needs special consideration, as well as biocompatibility. The orbit remains a controversial entity in the human body with respect to the appropriate material for fracture repair. Today, the search for a material with ideal characteristics is ongoing ( Table 2 ).
1. | Stability and fixation | Strong enough to support the orbital content and related forces |
Ability to be stable and retain its shape once manipulated | ||
No deformation (sagging of material into maxillary sinus) under pressure load | ||
Stable over time | ||
Possibility of being fixed to surrounding structures | ||
2. | Contouring and handling | Restores adequate volume to treat enophthalmos, diplopia, and motility disorders |
Easy to shape to fit the orbital defect and regional anatomy/malleability | ||
Adequate in three-wall fractures | ||
No sharp edges and smooth surface | ||
3. | Biological behaviour | Biocompatibility: no infection/extrusion/migration/foreign body reaction |
Chemically inert, non-allergenic, non-carcinogenic | ||
Durable with minimal resorption | ||
Osteoinductive/osteoconductive | ||
High tissue incorporation but readily dissected in implant removal during secondary reconstruction | ||
4. | Drainage | Spaces within the implant to allow drainage of orbital fluids |
5. | Donor site morbidity | Does not increase surgical complication rate/donor site morbidity (pain, swelling, etc.) |
6. | Radiopacity | Radiopaque to enable radiographic evaluation without artefacts |
7. | Availability and cost-effectiveness | Readily available in sufficient quantities |
Acceptable costs |
The aims of this study were (1) to provide a comprehensive overview of the advantages and disadvantages of both traditional and new materials for the reconstruction of traumatic orbital defects, (2) to define the ideal characteristics of implant materials for future research, and (3) to offer evidence-based clinical recommendations regarding the best suitable material available.
Advantages and disadvantages of currently available reconstruction materials
Biological materials
Biological materials are defined as grafts harvested from the same or another human or animal and include autografts, allografts, and xenografts. Autologous grafts are characterized by cost-effectiveness but limited availability, variable resorption rates leading to unpredictable (orbital) volume, associated donor site morbidity (pain, scarring, infection, haematoma), and an increased surgical time. In the past, viral infections and other diseases (e.g. bovine spongiform encephalopathy and Creutzfeldt–Jakob disease) originating from the donor tissue have been reported for allografts and xenografts.
Since the 18th century, autologous bone has been the ‘gold standard’ biomaterial for the reconstruction of bony defects in the craniofacial area. Autologous bone grafts are used in orbital surgery because of their strength, rigidity, vascularization potential, and incorporation into the orbital tissues with minimal acute and chronic immune reactivity (i.e. infection, extrusion, collagenous capsule formation, and ocular tethering). Calvarial bone appears to be a superior option in orbital reconstruction because of its accessibility, the various graft sizes that can be harvested, the hidden nature of the scar as a result of its location in a hair-bearing area, and the occurrence of little or no postoperative pain. Donor site morbidity remains a general drawback for autologous bone harvesting. In full-thickness calvarial harvesting, care has to be taken not to tear the dura, since this injury carries the risk of iatrogenic subarachnoid haemorrhage or even intracerebral haemorrhage and might require reconstruction itself. Iliac crest grafting carries an associated risk of peritonitis, haemorrhage, pain, anterior spine fracture, lateral femoral cutaneous nerve damage, increased hospitalization time, and possibly thrombo-embolism. Additional disadvantages of autologous bone are the difficulty that can occur in contouring the bone to the perfect shape (e.g. because of fracturing of the graft with bending). In addition, autologous bone grafting is associated with unpredictable resorption, especially rib and iliac bone, which are of endochondral origin. The latter is in contrast to calvarial bone, which is intramembranous in origin and is more stable. Resorption rates of 80% have been observed in iliac crest bone grafting. Resorption may be decreased by fixating of the graft, which promotes revascularization and osteoconduction.
Despite the popularity of autologous bone, cartilage is easier to harvest and much more malleable, and the relative avascularity of this tissue allows survival with minimal oxygen perfusion and less resorption. The nasal septum is particularly advantageous because of the rapid harvest time and the minimal cosmetic and functional morbidity. Bayat et al. performed a randomized clinical trial (RCT) and found a superior effect for nasal cartilage versus conchal cartilage with respect to the occurrence of enophthalmos at the 3–6-month follow-up. A second RCT found no significant differences in the clinical outcomes of orbital fractures repaired with either a cartilage graft or an absorbable polyacid co-polymer. In cartilage grafting, limited donor site morbidity is involved. The major drawback of cartilage use is acquiring sufficient tissue for large orbital defects.
Allografts (syn. homografts) are transplanted tissues (e.g. lyophilized dura mater or banked (demineralized) bone) from another human being. Their advantages include a decreased surgical time, preoperative customizability, absence of donor site morbidity (only in cadavers), and abundant availability of banked (demineralized) bone. Lyophilized dura (Lyodura) was a standard in the past for the reconstruction of smaller orbital defects because of its strength and absence of tissue reactions. However, it became controversial following a case of Creutzfeldt–Jakob prion disease in a patient who received dura originating from a cadaver. Consequent to this report, lyophilized dura sterilization was no longer performed with gamma irradiation but with sodium hydroxide. The disadvantages of allografts include a resorption rate substantially higher than that of autologous tissue, the need for immunosuppressive pharmacotherapy, and the alleged risk of viral transmission, such as hepatitis C virus and HIV. Demineralized bone sheets of 100–300-μm thickness have been shown to be too weak to support orbital prolapse in cases with enophthalmos.
The use of xenografts in bone reconstruction is generally not encouraged because it is associated with disease transmission, immunological transplant rejection, and unpredictable and high resorption rates. In contrast to biological materials, the use of manufactured implants saves operative time and avoids donor site morbidity.
Metals
Titanium has been used extensively in craniofacial surgery and dentistry in the form of implants, plates, and screws. With its high biocompatibility and physico-mechanical properties, it could be an ideal implant for covering large anatomical defects (categories III–V) and globe malposition if implant-stabilizing surrounding bone or a distal landmark (a ‘bony ledge’) is absent.
An attractive feature of titanium is its ability to be both incorporated into the surrounding tissues and to osseointegrate. Titanium mesh seems to be particularly suitable for reconstructing large orbital fractures. Computer-assisted designed and manufactured (CAD/CAM) titanium implants have enabled optimal reconstructive surgery, with the protection of vital structures such as the optic nerve. Titanium is strong, rigidly fixable, widely available, and is subject to osseointegration with minimal foreign body reaction. However, titanium is costly and may have irregular edges if not cut properly, which may impinge soft tissue. Furthermore, fibrous tissue will incorporate the mesh-holes, which can make implant replacement technically complex. Late unwanted effects such as infection, corrosion, and toxic metal ion release have been reported with the use of titanium implants. One RCT has evaluated the effects of titanium implants as compared to perforated polydioxanone (PDS) foil for small orbital floor fracture reconstruction, and found no significant differences in the clinical outcomes. A pilot study without controls used a low-profile 0.25-mm titanium plate in large defects (categories II and III) and found successful clinical outcomes without complications in 93% of the cases; at the 6-month follow-up, no functional or aesthetic concerns were observed.
Cobalt-based alloys such as vitallium are used widely in dentistry for their high resistance to corrosion. These alloys produce large artefacts on CT and magnetic resonance imaging (MRI) and have rarely been used in orbital surgery. These materials have been replaced by titanium in general prosthesiology.
Polymers
Polymers (or plastics) are large molecules comprising multiple repeated subunits and can be categorized into absorbable and non-absorbable (permanent) types.
Non-absorbable permanent polymer implants
Porous ultra-high density polyethylene (PE; Medpor) sheets of various sizes and thicknesses (0.4–1.5 mm) have been used widely to cover smaller floor defects since the 1990s. This widespread use is a product of the ability to easily cut the sheets into various shapes and the ability of orbital tissue to move freely over the smooth surface. Connective tissue and vascular components grow into the pores with minimal foreign body reaction. In a prospective cohort study of floor reconstructions, PE sheets showed satisfactory surgical outcomes and infection rates similar to autografts.
Silicone is flexible, easy to handle, chemically inert, and relatively cheap. Silicone implants are substantially less palpable than non-silicone implants (autografts, titanium, or resorbable plates). However, unacceptably high rates of implant extrusion, cyst formation, and infections have been found, especially in the early postoperative period. Indeed, 12% of orbital silicone implants require removal within 1 month after placement.
Polytetrafluoroethylene (PTFE; Teflon) is biologically and chemically inert, non-antigenic with minimal foreign body reaction, sterilizable, and easily mouldable. However, this polymer has not yet been subject to comparative clinical studies.
Relatively new in orbital floor repair is the use of nylon foil, a non-porous polyamide. Nylon foil has provided favourable results in preliminary non-comparative studies.
Hydrogels are a network of hydrophilic polymer chains in a watery gel, and possess flexibility similar to natural tissue. Hydrogels have shown promising results in animal research in delivering bone morphogenetic protein type 2 (BMP-2) locally, significantly stimulating local bone growth.
Absorbable polymer implants
These materials have been used widely for over 30 years in many fields of surgical practice, and are of interest because of their more predictable absorption rates than biological grafts, as well as their high level of customizability and control. Resorbable materials provide temporary support, leaving fibrous granulation tissue during their degradation. These materials do not necessarily require rigid fixation, can be applied in multiple layers in larger orbital volume displacement, and can be radiolucent on postoperative imaging.
In an RCT, the administration of an absorbable copolymer of poly(lactic acid) (PLA) and poly(glycolic acid) (PGA) had functional and aesthetic outcomes and complications similar to auricular cartilage implants in orbital blowout fractures with or without medial wall involvement. In addition, PLA 70/30 plates were studied in a controlled clinical trial and showed similar surgical outcomes and complications as compared to autografts in category II and III floor defects, without MRI evidence of foreign body reaction.
Polydioxanone (PDO, PDS) is used widely in surgery for resorbable sutures, which degrade completely in approximately 6 months. In a multi-centre RCT, perforated PDS foils of 0.15-mm thickness were found to have surgical outcomes similar to 0.3-mm titanium meshes in orbital floor reconstructions, although PDS foil was considered to be more convenient to handle. Another RCT compared the use of a porcine collagen membrane to a 0.15-mm PDS foil and observed that complications and clinical symptoms remained absent for 6 months after orbital floor surgery. In one prospective case series, PDS implants were suggested as inadvisable because of unsatisfactory orbital reconstructions and high complication rates.
In a retrospective cohort study, reconstructions with polyglactin 910/PDS (Ethisorb) flexible patches showed a similar postoperative orbital geometry as compared to 0.25-mm PDS foil in floor reconstructions. In contrast to reports that polyglactin 910/PDS implants have high infection rates, a retrospective study of 87 patients treated with this material found no postoperative infections during a 3-month follow-up.
Biological ceramics
Hydroxyapatite (HA), which is chemically and crystallographically similar to bone mineral, has been available for craniofacial surgery since the 1990s. However, in orbital surgery, it has been found to be inferior to porous PE sheets with regard to the postoperative outcomes of enophthalmos.
Bioactive glasses (BAGs) are synthetic blocks or granules that bond chemically to bone. The disadvantages of BAGs include their rather brittle nature and the lack of ease in moulding, shaping, and fixing them. Nonetheless, these materials have been demonstrated to be osteoinductive and osteoconductive as implants, and to cause minimal foreign body reaction, infection, extrusion, displacement, and resorption. The benefits of preformed bioglass implants need further research.
Composites
An interesting group of orbital implants is the composites because of the potential to utilize the advantages of a selected material while reducing its disadvantages through hybridization with a second material. A reciprocal process can allow the strengths of both materials to be used. A clinical example is titanium-reinforced PE. Titanium mesh offers the advantages of high strength and stability, easy contouring, and radiopacity in postoperative imaging, while PE implants have a smooth surface allowing the free movement of orbital tissue. In recent years, a composite material (titanium-reinforced porous PE) has become available for maintaining ocular function and facilitating a secondary surgery if necessary. A retrospective chart review found no significant differences in the clinical outcome measures between PE channel implants versus PE-reinforced titanium implants. Kim et al. suggested that reinforced titanium PE implants do not require screw fixation.
A promising addition to polymers may be an extra coating. Heparinized bone marrow-coated polycaprolactone (PCL) scaffolds have shown promising potential in animal research, showing significantly greater bone induction in comparison to non-coated PCL scaffolds.
To conclude, the predominant material investigated in the literature in the 1990s was autologous bone (45% bone versus 32% Medpor or titanium). A global trend towards the use of alloplastic material for orbital reconstruction can be identified. This trend is reflected in more recent publications, in which alloplastic materials have been chosen increasingly for orbital reconstruction (30% bone graft versus 46% alloplastic implants). This increasing preference for alloplastic materials is likely the result of their ease of use, technological advancements, absence of donor site morbidity, and an increasing level of evidence of the safety and efficacy of synthetic materials for this indication.
The ideal implant material
Introduction
In the search for the best material for orbital reconstruction, the most convenient approach may involve searching for the optimal material with reference to the fracture characteristics (e.g. fracture complexity, medical history, experience of the surgeon, costs). This approach could support a decision-making process for selecting an implant type based on typical fracture patterns. Seven material characteristics that would necessarily influence this clinical decision-making process are discussed ( Table 3 ): (1) stability and fixation, (2) contouring abilities, (3) biological behaviour, (4) drainage, (5) donor site morbidity, (6) radiopacity, and (7) availability and cost-effectiveness. Future developments and new technologies are discussed as well.
Stability | Contouring | Biological behaviour | Drainage | Donor site morbidity | Radiopacity | Availability | Cost-effectiveness |
---|---|---|---|---|---|---|---|
Titanium meshes (flat) | |||||||
Stability +++ | ++ contouring | ++ allows tissue ingrowth | + permeable | + | + | + | + |
Fixation ++ | − possible sharp edges | − poor dissection of peri-orbita in secondary reconstruction | |||||
Bone graft | |||||||
Stability ++ | + variability in thickness/smooth surface adequate in three-wall fractures | +++ maximal biocompatibility/peri-orbita readily dissects off bone in secondary reconstruction | − | − donor site needed: harvest time/pain/scarring/complications | + | +/− | +/− |
Fixation + | − remodelling/difficult to shape | ||||||
Porous polyethylene sheets | |||||||
Stability+− | + eased by artificial sterile skull/smooth edges | ++ allows tissue ingrowth | − | + | − not visible on postoperative imaging | + | + |
Lack of rigidity when thin | |||||||
Fixation+/− | |||||||
Composite of porous polyethylene and titanium mesh | |||||||
Stability ++ | + eased by artificial sterile skull, adequate in three-wall fractures | ++ allows tissue ingrowth | − | + | + | + | +/− |
Fixation ++ | |||||||
Resorbable materials | |||||||
Stability+/− | + smooth surface and edges/handling (thermoplastics) | +/− sterile infection/inflammatory response | − in case non-perforated: less drainage than uncovered titanium mesh | + | − not visible on postoperative imaging | + | + |
Stable over time? | − non-thermoplastics | ||||||
Fixation+/− | − degradation of material with risk of contour loss | ||||||
Preformed orbital implant | |||||||
Stability +++ | +++ minimal contouring necessary/smooth surface | ++ allows tissue ingrowth | + permeable | + | + | + | +/− |
Fixation ++ |
Stability and fixation
Restoring the original orbital volume is essential for recovering ocular function. To accomplish this, a reliable material that can reconstruct proper orbital volume and reposition the supporting tissues without significant resorption is required. Numerous materials have been used to achieve anatomical reconstruction and these differ with respect to their stability. Van Leeuwen et al. have developed a mathematical model to judge preoperatively whether a material is suitable based on four variables that influence deformation: (1) the size of the orbital defect, (2) the mechanical properties of the reconstruction material, (3) the thickness of the reconstruction material, and (4) the pressure load of the orbital content. Based on the properties of various biomaterials, the authors concluded that not all materials were suitable when varying defect sizes were considered. In particular, Jaquiéry category I and II defects can be treated safely with titanium, bone, PLA, and PE (see Table 4 ). For these small defects, a flexible material may generally be sufficient, whereas for the reconstruction of larger defects (Jaquiéry III and IV), a more rigid material (e.g. titanium) is required. Gross soft tissue prolapse, orbital pressure, and the absence of a posterior bony ledge are decisive factors.
Category | Complexity | Description | Note |
---|---|---|---|
Category I | Low | Isolated defect of the orbital floor or the medial wall, 1–2 cm 2 , within zones 1 and 2 | |
Category II | Medium | Defect of the orbital floor and/or of the medial wall, >2 cm 2 , within zones 1 and 2 | Bony ledge preserved at the medial margin of the infraorbital fissure |
Category III | High | Defect of the orbital floor and/or of the medial wall, >2 cm 2 , within zones 1 and 2 | Missing bony ledge medial to the infraorbital fissure |
Category IV | High | Defect of the entire orbital floor and the medial wall, extending into the posterior third (zone 3) | Missing bony ledge medial to the infraorbital fissure |
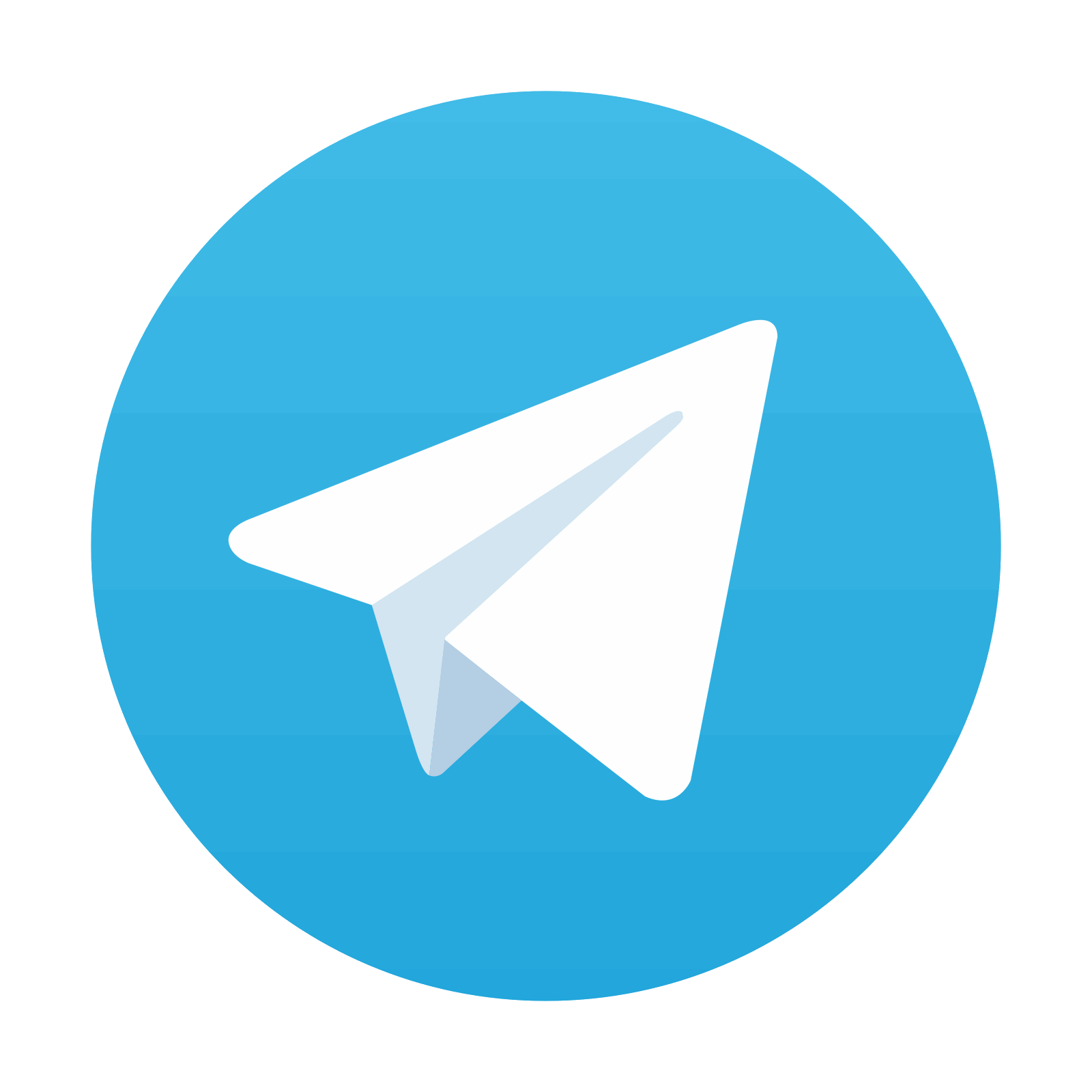
Stay updated, free dental videos. Join our Telegram channel

VIDEdental - Online dental courses
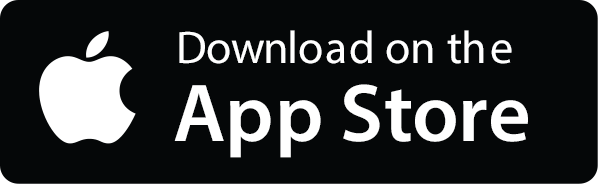
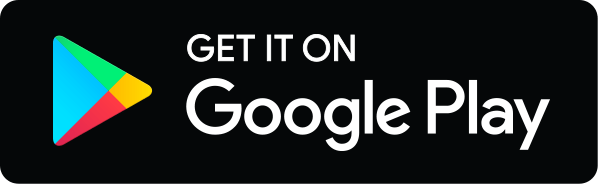
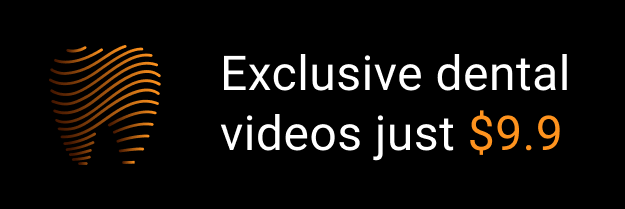