Highlights
- •
We developed controlled-release naringin nanoscaffolds.
- •
The nanoscaffolds were evaluated by an organ culture model.
- •
The nanoscaffolds enhance functions of osteoblasts.
- •
The nanoscaffolds suppress formation of osteoclasts.
Abstract
Objectives
Osteoporosis is one of the most common bone diseases in the world and results from an imbalance of bone cell functions. In the process of guided bone regeneration, osteoporosis weakens the bonding strength between scaffold and bone. Naringin is evidenced to be effective for the treatment of osteoporosis and bone resorption and the aim was to explore methods and benefits of its incorporation.
Methods
In this study, naringin was incorporated in the electrospun nanoscaffold containing poly(ɛ-caprolactone) (PCL) and poly(ethylene glycol)-block-poly(ɛ-caprolactone) (PEG-b-PCL).
Results
The nanoscaffold demonstrated unchanged chemical structure, improved hydrophilicity, thinner and more uniform nanofibers by Fourier-transform infrared spectroscopy, contact angle measurement and scanning electron microscopy. The nanoscaffold also showed faster degradation rate and controlled-release of naringin. Osteoblast-nanoscaffold interactions were studied by the evaluation of adhesion, proliferation, differentiation of MC3T3-E1 osteoblasts and mineralization of ECM on the nanoscaffolds. Meanwhile, the response of osteoclasts to nanoscaffolds was evaluated in a mouse calvarial critical size defect organ culture model. The osteoclasts around the bone defect were shown by tartrate resistant acid phosphatase staining.
Significance
The results demonstrated that controlled-release naringin nanoscaffolds supported greater osteoblast adhesion, proliferation, differentiation, and mineralization and suppressed osteoclast formation.
1
Introduction
Extensive craniomaxillofacial bone defects caused by tumor, infection, trauma and dysplasia are regularly repaired by bone substitution materials. However, the restorative effect depends not only on the implant properties but also on the characteristics of the host bone. Researchers pay increasing attention to the patients’ own diseases. Among them, osteoporosis is the most common systemic skeletal disorder that weakens the bonding strength between the implants and bone, delays bone defect repair, lowers the quality of new bone formation and even causes prosthesis failure . With the aging of populations, osteoporosis is a still increasing prevalence. As a part of the whole body bone, craniomaxillofacial bone is inevitably influenced by osteoporosis, showing reduced bone mass, bone mineral content and bone density, deterioration of the microstructure of the trabecular bone and lower osteogenesis ability. Although osteoporosis is not an absolute contraindication for bone repair, it increases implant failure and the risk of complications. Therefore, the treatment of bone defects in osteoporosis patients remains a challenge in dental medicine. Osteoporosis occurs when bone resorption is greater than bone formation. Thus, osteoclasts and osteoblasts imbalance correction may be a therapeutic modality for bone repair of the patients with osteoporosis.
The most widely used drugs to treat osteoporosis are bisphosphonates. Bisphosphonates maintain bone mass by inhibiting the function of osteoclasts . However, bisphosphates have been reported to cause osteoclast dysfunction and even osteonecrosis . Researchers showed that naringin plays a dual role in regulation of osteoblasts and osteoclasts. Naringin, a polymethoxylated flavonoid, is an active constituent of Rhizoma Drynariae (Traditional Chinese Medicine). Naringin has been reported to stimulate proliferation and differentiation of osteoblastic cell lines and inhibit osteoclast formation . Naringin has been shown to inhibit HMG-CoA reductase, activate the BMP-2 promotor, increase bone formation and induce the expression of osteogenic markers of osteoblasts . Naringin can also dose-dependently suppress the number of osteoclasts formed by the treatment with interleukin-1 (IL-1) . Chen et al. has reported that naringin increased alkaline phosphatase activity, osteocalcin level, bone mineral density and decreased the number of osteoclasts. In addition, naringin has been shown to improve bone quality of rats with osteoporosis induced by retinoic acid and orchidectomized rats .
Guided bone regeneration (GBR) is a method to repair bone defects, which emerged with the development of biology and bioengineering. GBR scaffolds are used as barriers to prevent soft tissue ingrowth and create space for slowly regenerating periodontal and bony tissues. For the past few years, the electrospinning technique has been used widely in fabrication of scaffolds for bone , tendon , vasculature and neural tissue regeneration. Electrospun scaffolds with a large surface area-to-volume ratio, high porosity and morphology similar to the extracellular matrix (ECM) of natural tissue can serve as an ideal GBR scaffold .
In this study, the advantages of electrospinning technology and an active constituent of a Traditional Chinese Medicine (Rhizoma Drynariae) were combined for the first time. Naringin was incorporated into the electrospun nanofibers, which served as a controlled-release GBR scaffold. The properties of the controlled-release naringin scaffold (such as chemical, hydrophilic and morphological characteristics, drug release, material degradation, osteoblast-scaffold interactions, and osteoclast-scaffold interactions) were studied to evaluate its potential for the treatment of bone defects in osteoporosis patients.
2
Materials and methods
2.1
Materials
Poly-ɛ-caprolactone (PCL) (Mw = 80,000), poly(ethylene glycol)-block-poly(ɛ-caprolactone) (PEG-b-PCL) (PEG Mw = 5000; PCL Mw = 5000) and naringin were obtained from Sigma–Aldrich (St. Louis, MO). MC3T3-E1 osteoblast line and 4-day-old CD-1 mice were obtained from the life science Institute of Chongqing Medical University. Dulbecco’s Modified Eagle’s Medium (DMEM), fetal bovine serum (FBS), antibiotics and trypsin–EDTA from GIBCO Invitrogen (USA) were used.
2.2
Electrospinning
PCL was dissolved in acetone to obtain a 15% (w/v) solution. PCL and PEG-b-PCL were mixed at a weight ratio of (1:1) to obtain PCL/PEG-b-PCL solution. Naringin (3.33 mg/ml) was added to PCL and PCL/PEG-b-PCL solution to obtain PCL/naringin and PCL/PEG-b-PCL/naringin solution. The solutions were separately loaded in 5 ml syringes, delivered at a constant flow rate ( Q = 3 ml/h, Harvard Apparatus PHD 2000 syringe pump, Holliston, MA). A voltage of 15 kV was applied using a high voltage power supply (High Voltage System, Gamma High Voltage Research, FL, USA). A positively charged jet was formed from the Taylor Cone. Nanofibers were sprayed onto a grounded aluminum plate kept 12 cm away from the needle tip. Nanofibers were collected on the coverslips (10 mm × 10 mm) for cell and organ culture experiments, while those collected on the aluminum foil were used for Fourier-transform infrared spectroscopy (FT-IR), contact angle measurement, scanning electron microscopy (SEM), drug release and in vitro degradation studies. The electrospun nanofibers were stored in desiccators for several days to remove the residual acetone.
2.3
FT-IR
FT-IR (Nicolet 5DXC spectrometer, USA) was used to qualitatively analyze and the materials before and after electrospinning. The naringin and PEG-b-PCL samples were pressed into pellets with KBr. The PCL samples were hot pressed into membranes. The controlled-release naringin nanoscaffolds were observed directly by FT-IR.
2.4
Contact angle measurement
For determination of wettability of nanoscaffolds, the contact angles of electrospun nonwoven materials were measured by a video contact angle system (DSA100, Siber Hegner, China) mounted with a CCD camera at an ambient temperature. Distilled water was used as the test liquid. Each experiment was repeated six times. Means were shown as the results.
2.5
Morphologies of nanoscaffolds
The morphologies of electrospun nanoscaffolds were observed by SEM (JSM-5600LV, JEOL, Japan) at an accelerating voltage of 30 kV after gold coating. The average fiber diameters of the electrospun nanofibers were measured using Image-ProPlus 5.02 (Media Cybernetics, USA).
2.6
Drug release assay in vitro
A specimen of nanoscaffold (PCL/PEG-b-PCL/naringin and PCL/naringin) (20 mm × 20 mm) was first placed in a vial filled with 2 ml phosphate buffer solution (PBS). Naringin release studies were carried out at 37 °C and 100 rotation/min (rpm) in a thermostatic shaking incubator (THZ-92A, GuangTe Instrument Co., Ltd., China). In this case, 0.5 ml samples were taken from the medium after 0, 1, 2, 6, 10, 14, 21, 30, 60, 90 days, and then the same volume of fresh PBS was added as replacement. Naringin in the medium was determined by a UV–vis spectrophotometer (Nanodrop2000, Thermo, USA) at the wavelength of 284 nm. The results were presented in terms of cumulative release as a function of release time:
where Mt was the amount of naringin released from a sample at time t . The total amount of naringin in a sample was calculated and regarded as M ∞ in this study. Three samples were tested for each electrospun nanoscaffold and the results were reported as average values.
2.7
Nanoscaffold degradation assay in vitro
PCL and PCL/PEG-b-PCL/naringin nanoscaffolds (20 mm × 20 mm) were placed in 24-well plates containing 1 ml PBS in each well and were incubated at 37 °C for different periods of time (0, 10, 20, 30, 40, 50, 60 days). After each degradation period, the scaffolds were washed and subsequently dried in a vacuum oven at room temperature for 24 h. The weight loss of the scaffolds was calculated according to the following equations:
W % is the percentage of weight loss, W i is the initial weight of the scaffold and W t is the weight of the scaffold at the time t of the experiment after drying to constant weight.
2.8
Osteoblast-nanoscaffold interactions
2.8.1
Cell seeding and culture
Both sides of the nanoscaffolds were exposed to UV radiation for 2 h, washed three times with PBS for 20 min and incubated with DMEM for 24 h before cell seeding. MC3T3-E1 osteoblasts were cultured in DMEM with 10% FBS and 1% penicillin/streptomycin. After reaching 80–90% confluence, the cells were detached by trypsin–EDTA. Each of the nanoscaffolds on coverslips (10 mm × 10 mm) was placed in a 24-well plate and pressed with cell crowns (Sigma–Aldrich, USA) to avoid possible floating during cell culture. Cells were further seeded on nanoscaffolds at a density of 2 × 10 4 cells/cm 2 and cultured with DMEM at 37 °C, 5% CO 2 and 95% humidity. The cell-seeded nanoscaffolds were replenished with fresh medium every 3 days.
2.8.2
Cell adhesion and proliferation by MTS
In order to observe the cell adhesion and proliferation at different times, viable cells were determined by colorimetric MTS assay (CellTiter 96 ® AQueous Assay). After 1, 2, 4 h and 2, 6, 10, 14 days of cell seeding in the 24-well plates, nanoscaffolds with MC3T3-E1 osteoblasts (constructs) were washed with PBS and incubated with 20% of MTS reagent containing serum free media. After 4 h of incubation at 37 °C in 5% CO 2 and 95% humidity, aliquots were pipetted into a 96-well plate and the absorbance was read at 490 nm using an ELISA microplate reader (Bio-Tek Instruments Inc., Winooski, VT, USA).
2.8.3
Cell differentiation by ALP
The differentiation ability of MC3T3-E1 seeded on different nanoscaffolds was analyzed by the expression of alkaline phosphatase (ALP) activity of the cells. In this study, cells were cultured on the nanoscaffolds for 2, 6, 10 and 14 days, and then assessed for ALP activity. The cell-scaffold constructs were washed twice with PBS prior to analysis. To determine ALP activity, the adhered cells were lysed with 1% Triton-X100 (Huamei Biological Engineering Co., China) overnight. A commercially available ALP Colorimetric Assay Kit (Jiancheng Biological Engineering Institute, Nanjing, China) was used to quantify ALP concentration in the lysate. The absorbance was measured at 405 nm using an ELISA microplate reader (Bio-Tek Instruments Inc., Winooski, VT, USA). And converted to the units of ALP per liter.
2.8.4
SEM observations
In order to observe cell morphologies on different nanoscaffolds, cells were fixed after 7 days of culture. A cellular construct was rinsed twice with PBS and subsequently fixed in 2% glutaraldehyde for 1.5 h at room temperature. Then each cellular construct was rinsed with distilled water, dehydrated with graded concentrations of ethanol solution and finally dried in hexamethyldisilazane overnight. Dried cellular constructs were sputter coated with gold and observed under SEM at an accelerating voltage of 20 kV.
2.8.5
Alizarin Red-S (ARS) staining
Cellular constructs were washed five times in PBS and fixed in ice cold 70% ethanol for 1 h. Then these constructs were washed three times with distilled water and stained with 40 mM ARS for 30 min at room temperature. Microscopic images of the stain showing calcium deposition were taken.
2.9
Osteoclast-scaffold interactions
2.9.1
Mouse calvarial organ culture
The heads of 4-day-old CD-1 mice were provided by the life science Institute of Chongqing Medical University. The calvarial of mouse heads were exposed. The parietal bones were dissected out aseptically. During dissection, periosteum and dura mater were left intact on the bone surfaces. Full-thickness, 1.5 mm in diameter, circular defects were created through the parietal bones. The paired bones were divided into two groups. The critical size defect (CSD) model of mouse calvarial bone was based on our previous research . In the present study, the CSD models were used for the evaluation of the responses of osteoclasts to PCL/PEG-b-PCL/naringin and PCL nanoscaffolds. These two types of scaffolds were cut into small disks (1.5 mm diameter) by a hollow steel tube, and then grafted into the bone defect. The bones with scaffolds were cultured concave side down on the tissue culture plate in DMEM with 10% FBS and 1% penicillin/streptomycin. Incubations were carried out at 37 °C, 5% CO 2 and 95% humidity. Media were changed every 3 days.
2.9.2
Osteoclast observing by tartrate resistant acid phosphatase (TRAP) staining
After 14 days’ culture, the bones with scaffolds were stained for TRAP (Acid Phosphatase Kit 387-A; Sigma–Aldrich, St. Louis, MO, USA) according to the manufacturer’s instructions. TRAP-positive cells appeared red or purple. Images were taken by transmitted light microscopy (Olympus IX71, Japan) with a digital camera (Canon 500D, Japan).
2.10
Statistical analysis
All data presented are expressed as mean ± standard deviation (SD). Statistical analysis was performed using one-way analysis of variance (ANOVA) and significance was considered at p < 0.05.
2
Materials and methods
2.1
Materials
Poly-ɛ-caprolactone (PCL) (Mw = 80,000), poly(ethylene glycol)-block-poly(ɛ-caprolactone) (PEG-b-PCL) (PEG Mw = 5000; PCL Mw = 5000) and naringin were obtained from Sigma–Aldrich (St. Louis, MO). MC3T3-E1 osteoblast line and 4-day-old CD-1 mice were obtained from the life science Institute of Chongqing Medical University. Dulbecco’s Modified Eagle’s Medium (DMEM), fetal bovine serum (FBS), antibiotics and trypsin–EDTA from GIBCO Invitrogen (USA) were used.
2.2
Electrospinning
PCL was dissolved in acetone to obtain a 15% (w/v) solution. PCL and PEG-b-PCL were mixed at a weight ratio of (1:1) to obtain PCL/PEG-b-PCL solution. Naringin (3.33 mg/ml) was added to PCL and PCL/PEG-b-PCL solution to obtain PCL/naringin and PCL/PEG-b-PCL/naringin solution. The solutions were separately loaded in 5 ml syringes, delivered at a constant flow rate ( Q = 3 ml/h, Harvard Apparatus PHD 2000 syringe pump, Holliston, MA). A voltage of 15 kV was applied using a high voltage power supply (High Voltage System, Gamma High Voltage Research, FL, USA). A positively charged jet was formed from the Taylor Cone. Nanofibers were sprayed onto a grounded aluminum plate kept 12 cm away from the needle tip. Nanofibers were collected on the coverslips (10 mm × 10 mm) for cell and organ culture experiments, while those collected on the aluminum foil were used for Fourier-transform infrared spectroscopy (FT-IR), contact angle measurement, scanning electron microscopy (SEM), drug release and in vitro degradation studies. The electrospun nanofibers were stored in desiccators for several days to remove the residual acetone.
2.3
FT-IR
FT-IR (Nicolet 5DXC spectrometer, USA) was used to qualitatively analyze and the materials before and after electrospinning. The naringin and PEG-b-PCL samples were pressed into pellets with KBr. The PCL samples were hot pressed into membranes. The controlled-release naringin nanoscaffolds were observed directly by FT-IR.
2.4
Contact angle measurement
For determination of wettability of nanoscaffolds, the contact angles of electrospun nonwoven materials were measured by a video contact angle system (DSA100, Siber Hegner, China) mounted with a CCD camera at an ambient temperature. Distilled water was used as the test liquid. Each experiment was repeated six times. Means were shown as the results.
2.5
Morphologies of nanoscaffolds
The morphologies of electrospun nanoscaffolds were observed by SEM (JSM-5600LV, JEOL, Japan) at an accelerating voltage of 30 kV after gold coating. The average fiber diameters of the electrospun nanofibers were measured using Image-ProPlus 5.02 (Media Cybernetics, USA).
2.6
Drug release assay in vitro
A specimen of nanoscaffold (PCL/PEG-b-PCL/naringin and PCL/naringin) (20 mm × 20 mm) was first placed in a vial filled with 2 ml phosphate buffer solution (PBS). Naringin release studies were carried out at 37 °C and 100 rotation/min (rpm) in a thermostatic shaking incubator (THZ-92A, GuangTe Instrument Co., Ltd., China). In this case, 0.5 ml samples were taken from the medium after 0, 1, 2, 6, 10, 14, 21, 30, 60, 90 days, and then the same volume of fresh PBS was added as replacement. Naringin in the medium was determined by a UV–vis spectrophotometer (Nanodrop2000, Thermo, USA) at the wavelength of 284 nm. The results were presented in terms of cumulative release as a function of release time:
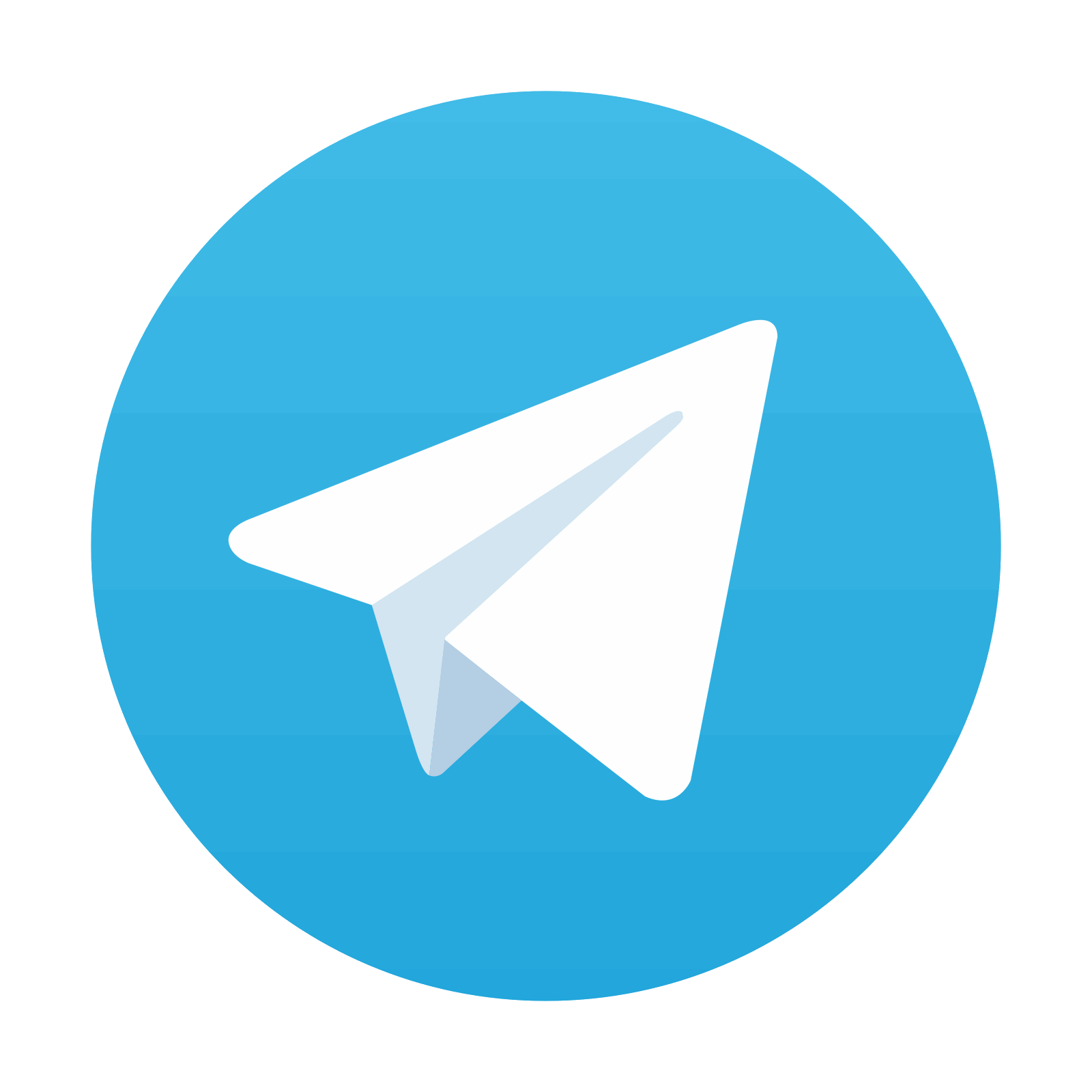
Stay updated, free dental videos. Join our Telegram channel

VIDEdental - Online dental courses
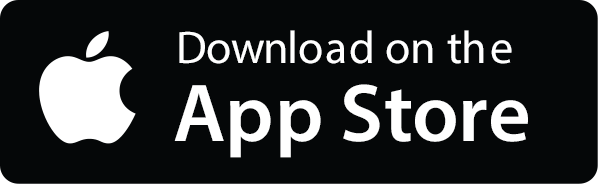
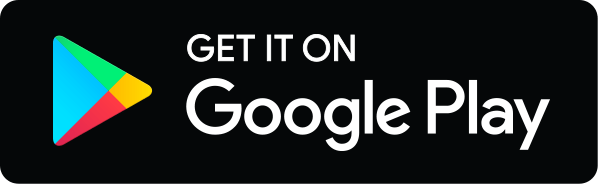