Abstract
Objectives
The contraction stress of a silorane-based material and a new low-shrinkage nanohybrid composite were compared to three conventional dimethacrylate-based resin composites using two different measuring systems. It was hypothesized that the silorane-based material and the low-shrinkage nanohybrid composite would exhibit lower contraction stress than dimethacrylate-based composites irrespective of measuring system.
Methods
The materials tested were Filtek Silorane LS (3M ESPE), Venus Diamond (Heraeus Kulzer), Tetric EvoCeram (Ivoclar Vivadent), Quixfil (Dentsply DeTrey), and Filtek Z250 (3M ESPE). Shrinkage stress was assessed using a stress–strain analyzer consisting of two opposing attachments, one connected to a load sensor and the other fixed to the device, or a system fixed to a universal testing machine with an extensometer as a feedback system. All specimens were light-cured with 20 J/cm 2 ; the contraction force (N) generated during polymerization was continuously recorded for 300 s. Contraction stress (MPa) was calculated at both 40 s and 300 s. Data were statistically analyzed by three-way ANOVA and Tukey’s post hoc test ( α = 0.05).
Results
Venus Diamond exhibited the lowest stress under both experimental conditions. Stress values scored as follows: Venus Diamond < Tetric EvoCeram < Filtek Silorane LS < Quixfil < Filtek Z250 ( p < 0.05). Stress values measured with the stress–strain analyzer were significantly lower than those measured with the universal testing machine with feedback.
Significance
The hypothesis was partially rejected because only Venus Diamond exhibited the lowest stress values among the tested materials. Contraction stress was higher for all composites when measured in a test system with a feedback. This study confirms that simply reducing the shrinkage does not ensure reduced stress development in composites.
1
Introduction
Despite extensive improvements in the mechanical properties of resin-based tooth-colored restorative materials, volumetric shrinkage and subsequent contraction stress arising during the polymerization reaction are still significant drawbacks. Contraction stress transferred to the tooth may lead to cusp deflection or enamel microcracks, whereas stress at the tooth–composite interface has the potential to cause adhesive failure, leading to post-operative sensitivity, microleakage, marginal discoloration, and recurrent caries .
Several studies have attempted to measure the development of polymerization stress ; however, stress values recorded in vitro are influenced by the deformation of the system components subjected to the forces of composite shrinkage. These deformations are commonly referred as “system compliance” . In low-compliance systems, the composite under investigation is placed between two flat surfaces connected to opposing rigid attachments of the stress analyzing equipment. The low-compliance setup is achieved through a feedback system that constantly monitors the material shrinkage and counteracts the displacement of the cross-head in the opposite direction, keeping the specimen height constant . Therefore, the load cell registers the force needed to maintain the initial height of the specimen, and stress is calculated by dividing the force by the cross-sectional area of the bonded surface . The system is otherwise rigid and made from generally high elastic modulus materials, such as steel or glass. Typically in high-compliance systems a feedback apparatus is not included, and shrinkage stress is represented by the force needed to deform the load cell and other components of the testing equipment that may resist the contraction of the composite .
The contraction stress of a resin composite is related to system compliance. Usually, high-compliance systems lead to lower stress values than those obtained with near-zero compliance systems, which may overestimate stresses associated with the clinical situation, where varying levels of compliance exist depending upon the tooth characteristics and geometry of the cavity preparation .
As the composite material is constrained by the bonded surfaces during polymerization, the compliance of the tooth structure may partially relieve the development of stress through tooth deformation in vivo . A variable termed the configuration factor, or C -factor, of the cavity preparation has been used to describe this constraint. The C -factor is expressed as the ratio of the bonded surfaces to the unbonded surfaces of the restoration, and has been considered to be one of the critical factors in the development of contraction stress in resin composite restorations .
Based on the intensive research on shrinkage and stress development, different approaches have been proposed to reduce polymerization shrinkage and minimize the stress of resin-based restorative materials, including incremental placement techniques , the development of soft-start polymerization , the use of low-modulus intermediate layers , and alternative chemical formulations of dimethacrylate-based composites . Additionally, several low-shrinkage materials were proposed as alternatives to dimethacrylate resins , but none of them have proven overall to offer a significant improvement to Bis-GMA-based composites.
Recently, new low shrinking commercial dental composites have been introduced. Filtek Silorane LS (3M ESPE, St. Paul, MN, USA) is claimed by the manufacturer to be a low-shrinkage composite based on a silorane resin consisting of siloxane and oxirane functional moieties. The siloxane determines the highly hydrophobic nature of the siloranes, and the cycloaliphatic oxirane functional groups are claimed to be responsible for the decreased shrinkage of siloranes compared to methacrylate-based composites. Oxiranes, which are cyclic ethers, polymerize via a cationic ring-opening mechanism, whereas typically methacrylates polymerize via a free-radical mechanism . Preliminary studies on the silorane-based material showed reduced volumetric shrinkage and reduced cuspal deflection compared to conventional dimethacrylate-based composites.
Venus Diamond (Heraeus Kulzer, Armonk, NY) is a new nanohybrid universal composite containing a novel monomer (TCD-DI-HEA, (Bis-(acryloyloxymethyl)tricyclo[5.2.1.02,6]decane) that is said to combine low shrinkage with low viscosity.
The purpose of this study was to measure the contraction stress of a silorane-based restorative material and a new low-shrinkage nanohybrid composite compared to three conventional dimethacrylate-based composites during photo-polymerization with a halogen curing light, using a universal testing machine provided with a feedback system and a stress analyzer with no feedback. The hypotheses tested were: (1) the silorane-based and the low-shrinkage nanohybrid composites develop lower contraction stress during photo-polymerization than conventional dimethacrylate-based restorative materials irrespective of the stress testing method and (2) testing system affects the measured stress values.
2
Materials and methods
The materials tested ( Table 1 ) were a silorane-based restorative material Filtek Silorane LS (3M ESPE); a low-shrinkage nanohybrid composite (Venus Diamond, Heraeus Kulzer); a nanohybrid composite (Tetric EvoCeram, Ivoclar Vivadent, Schaan, Liechtenstein); a packable composite (Quixfil, Dentsply DeTrey GmbH, Konstanz, Germany) and a universal microhybrid composite (Filtek Z250, 3M ESPE).
Composite | Shade | Resin composition | Filler composition | Filler volume | Average filler size |
---|---|---|---|---|---|
Filtek Silorane LS silorane-based composite | A2 | Silorane | Quartz and yttrium fluoride | 53% | 0.1–0.2 μm |
Venus Diamond nanohybrid composite | A2 | TCD-DI-HEA UDMA |
Barium aluminum fluoride glass | 64% | 5 nm–20 μm |
Tetric EvoCeram nanohybrid composite | A2 | Bis-GMA, UDMA | Barium glass, ytterbium trifluoride, Ba–Al-fluorosilicate glass, SiO 2 | 55% | 40 nm and 3 μm (mean particle size 550 nm) |
Quixfil hybrid packable composite | A2 | Bis-EMA, TEGDMA, UDMA, TMPTMA | ZrO 2 –SiO 2 | 66% | 1–10 μm |
Filtek Z250 microhybrid composite | A2 | Bis-GMA, UDMA, Bis-EMA | ZrO 2 –SiO 2 | 60% | 0.01–3.5 μm |
2.1
Stress–strain analyzer
Shrinkage stress during polymerization was assessed using a stress–strain analyzer ( Fig. 1 ) . The setup consisted of two identical opposing parallel stainless steel attachments with a preset distance of 2 mm. One was connected to a load sensor (20 N Tekkal, Milano, Italy) and the other was fixed to the aluminum frame of the device. A silicone tray was fixed to the testing device, creating a simulated cavity between the attachments (Ø 2 mm, 2 mm in height; C -factor = 0.5). The attachments were ground by 180 grit sandpaper before each measurement and a layer of hydrophobic unfilled resin (Scotchbond Multipurpose Plus, 3M ESPE) was applied and polymerized for 40 s with a quartz–tungsten halogen curing light using a previously tested output of 500 mW/cm 2 (Elipar 2500, 3M ESPE). For Filtek Silorane LS its adhesive was used (Silorane System Adhesive Bond, 3M ESPE).
A defined quantity of composite paste (10 mg) for each tested material was placed in the cavity in bulk, isolated with a Mylar matrix, and polymerized with the quartz–tungsten halogen curing unit (Elipar 2500, 3M ESPE) for 40 s. The contraction force (N) generated during polymerization was continuously recorded for 300 s after photo-initiation. Preliminary investigations revealed that no stress occurred after that time (i.e. a plateau of the curve was achieved). Each experiment was conducted at room temperature (23–24 °C) and repeated 10 times for each material ( N = 10).
2.2
Universal testing machine
The setup consisted of two sandblasted stainless steel cylinders as bonding substrates, Ø 2 mm and 25 mm in height. The two cylinders were fixed to the upper and lower clamps of a universal testing machine (Sun 500, Galdabini, Cardano al Campo, VA, Italy) ( Fig. 2 ). The lateral surface of the stainless steel cylinder was threaded to improve the retention of the testing machine clamps. The attachments were ground by 180 grit sandpaper before each measurement and a layer of hydrophobic unfilled resin (Scotchbond Multipurpose Plus, 3M ESPE) was applied and polymerized for 40 s with a quartz–tungsten halogen curing light using a previously tested output of 500 mW/cm 2 (Elipar 2500, 3M ESPE). For Filtek Silorane LS its adhesive was used (Silorane System Adhesive Bond, 3M ESPE).
A Mylar film was placed around the lower rod and filled up with the composite. After placing the composite, the upper cylinder was lowered and inserted into the upper hole of the mold, and the distance between the two cylinders was set to 2 mm (Ø 2 mm, 2 mm in height; C -factor = 0.5). An extensometer (model 2630-101, Instron, Norwood, MA, USA) was attached to the cylinders and provided an electronic feedback loop in the system to keep the specimen height constant (within 0.1 μm) during the test.
Specimens were polymerized as described above. The force (N) necessary to keep specimen height constant was recorded by the load cell for 300 s after photo-initiation.
Each experiment was conducted at room temperature (23–24 °C) and repeated 10 times for each material ( N = 10).
Contraction stress (MPa) was calculated at 40 s (at the end of light exposure) and 300 s as the force value (N) per area unit (force value/bonded surface area).
The rate of stress generation was obtained by calculating the derivative of the smoothed stress vs. time curves using data-analysis software (Logger Pro 3.5, Vernier Software & Technology, Beaverton, OR). The maximum stress rate (expressed as MPa/s) was obtained from the stress vs. time curves during the 40 s light exposure.
2.3
Statistical analysis
Data were statistically analyzed by three-way ANOVA. The tested variables were composite, analysis system and time. Differences between groups were identified using Tukey’s multiple comparison test at α = 0.05.
2
Materials and methods
The materials tested ( Table 1 ) were a silorane-based restorative material Filtek Silorane LS (3M ESPE); a low-shrinkage nanohybrid composite (Venus Diamond, Heraeus Kulzer); a nanohybrid composite (Tetric EvoCeram, Ivoclar Vivadent, Schaan, Liechtenstein); a packable composite (Quixfil, Dentsply DeTrey GmbH, Konstanz, Germany) and a universal microhybrid composite (Filtek Z250, 3M ESPE).
Composite | Shade | Resin composition | Filler composition | Filler volume | Average filler size |
---|---|---|---|---|---|
Filtek Silorane LS silorane-based composite | A2 | Silorane | Quartz and yttrium fluoride | 53% | 0.1–0.2 μm |
Venus Diamond nanohybrid composite | A2 | TCD-DI-HEA UDMA |
Barium aluminum fluoride glass | 64% | 5 nm–20 μm |
Tetric EvoCeram nanohybrid composite | A2 | Bis-GMA, UDMA | Barium glass, ytterbium trifluoride, Ba–Al-fluorosilicate glass, SiO 2 | 55% | 40 nm and 3 μm (mean particle size 550 nm) |
Quixfil hybrid packable composite | A2 | Bis-EMA, TEGDMA, UDMA, TMPTMA | ZrO 2 –SiO 2 | 66% | 1–10 μm |
Filtek Z250 microhybrid composite | A2 | Bis-GMA, UDMA, Bis-EMA | ZrO 2 –SiO 2 | 60% | 0.01–3.5 μm |
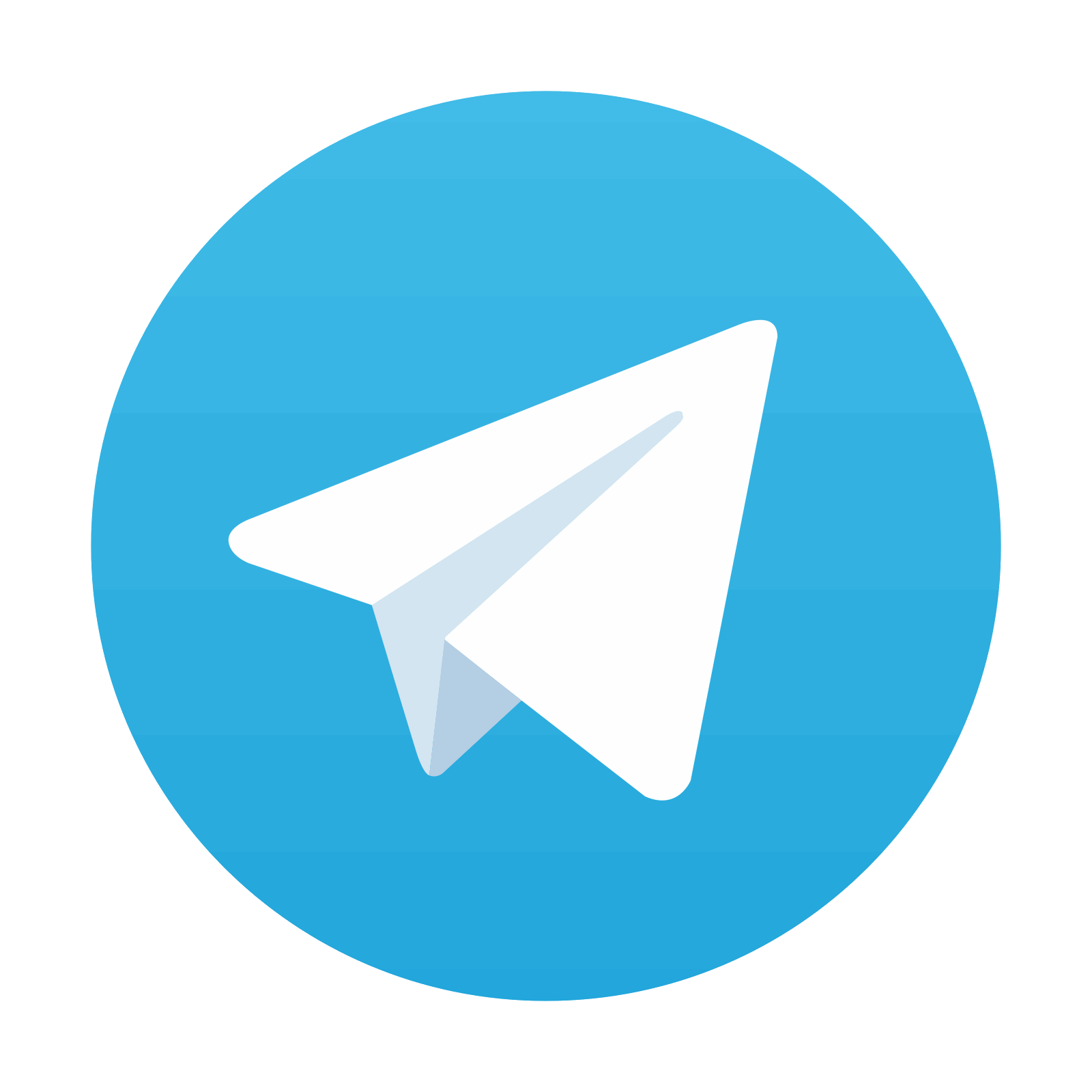
Stay updated, free dental videos. Join our Telegram channel

VIDEdental - Online dental courses
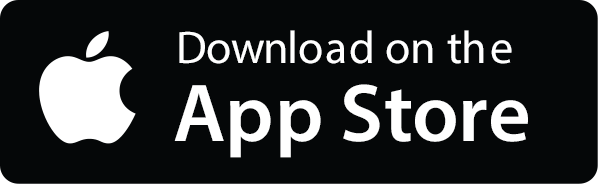
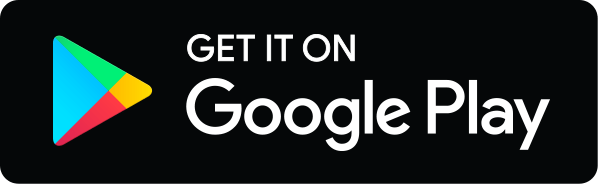
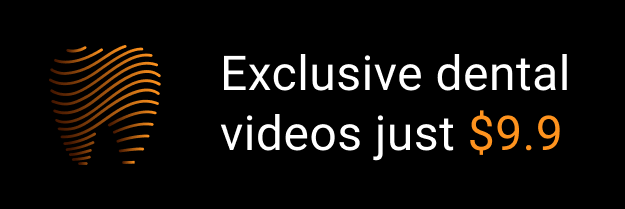