Abstract
Objective
To investigate contact angle and surface free energy of experimental dental resin composites containing novel delivery systems of polymeric hollow beads and low-surface tension agents after chewing simulation test.
Methods
A delivery system of novel polymeric hollow beads differently loaded with two low-surface tension agents was used in different amounts to modify commonly formulated experimental dental resin composites. The non-modified resin was used as standard. Surface roughness R a , contact angle Θ , total surface free energy γ S , its apolar <SPAN role=presentation tabIndex=0 id=MathJax-Element-1-Frame class=MathJax style="POSITION: relative" data-mathml='γSLW’>γLWSγSLW
γ S LW
, polar <SPAN role=presentation tabIndex=0 id=MathJax-Element-2-Frame class=MathJax style="POSITION: relative" data-mathml='γSAB’>γABSγSAB
γ S AB
, Lewis acid <SPAN role=presentation tabIndex=0 id=MathJax-Element-3-Frame class=MathJax style="POSITION: relative" data-mathml='γS+’>γ+SγS+
γ S +
and base <SPAN role=presentation tabIndex=0 id=MathJax-Element-4-Frame class=MathJax style="POSITION: relative" data-mathml='γS−’>γ−SγS−
γ S −
terms were determined and the results prior to and after chewing simulation test were compared. Significance was p < 0.05.
Results
After chewing simulation R a increased, Θ decreased, R a increased for two test materials and γ S decreased or remained constant for the standard or the test materials after chewing simulation. R a of one test material was higher than of the standard, Θ and γ S of the test materials remained lower than of the standard and, indicating their highly hydrophobic character ( Θ ≈ 60–75°, γ S ≈ 30 mJ m −2 ). <SPAN role=presentation tabIndex=0 id=MathJax-Element-5-Frame class=MathJax style="POSITION: relative" data-mathml='γSLW’>γLWSγSLW
γ S LW
, and <SPAN role=presentation tabIndex=0 id=MathJax-Element-6-Frame class=MathJax style="POSITION: relative" data-mathml='γS−’>γ−SγS−
γ S −
of the test materials were lower than of the standard. Some of the test materials had lower <SPAN role=presentation tabIndex=0 id=MathJax-Element-7-Frame class=MathJax style="POSITION: relative" data-mathml='γSAB’>γABSγSAB
γ S AB
and <SPAN role=presentation tabIndex=0 id=MathJax-Element-8-Frame class=MathJax style="POSITION: relative" data-mathml='γS+’>γ+SγS+
γ S +
than of the standard.
Significance
Delivery systems based on novel polymeric hollow beads highly loaded with low-surface tension agents were found to significantly increase contact angle and thus to reduce surface free energy of experimental dental resin composites prior to and after chewing simulation test.
1
Introduction
Contact angle Θ and surface free energy (SFE) are discussed to influence plaque formation on modern dental filling composites . Since there is a positive correlation between SFE and early plaque formation , dental resin composites differing in contact angle Θ were broadly investigated and bacterial adherence of materials with high Θ and low SFE was found to be significantly less . But it was also reported that no correlation occurred between streptococcal adhesion and substratum surface roughness or SFE and it was realized that the influence of SFE on bacterial adhesion decreased significantly after saliva coating .
The present work is related to earlier investigations reporting an entirely new approach to obtain resin materials with low SFE but acceptable physical properties which also proved diminished bacterial adherence . Both papers reported about a new delivery system of polymer hollow beads (Poly-Pore, AMCOL Health & Beauty Solutions, Arlington Heights, IL, USA) as carrier material, highly loaded with two different low-surface tension agents (Tego Protect 5000, Evonik Tego Chemie GmbH Essen, Germany and Dimethicone, Dow Corning Corp., Midland, MI, USA) which were added in small quantities of 5–6 wt.% to experimental dental resin composites. It was found that due to abrasion processes, simulated by polishing, the carrier hollow beads were destroyed and released the low-surface tension agents which flushed the surface and thus reduced the resin’s SFE . Now the effect of the exposition of the low-surface tension agents on SFE was re-investigated under more clinically relevant conditions with a chewing simulation device. To simplify the comparison with the previous papers the selected experimental resins were coded accordingly. The null hypothesis was that the SFE of the delivery system containing material did not differ from the standard after performing the chewing simulation test.
2
Materials and methods
2.1
Test material preparation (analog )
Four experimental light-curing resin-based restorative materials (A, B, C and E) were prepared ( Tables 1 and 2 ) using a laboratory vacuum planet kneader (Herbst Maschinenfabrik GmbH, Buxtehude, Germany). Material ST, representing a common formulation for dental resin composites, was used as the standard. The preparation process of all test materials and ST was done under vacuum at 50 °C. Preparation of the test materials: Poly-Pore was loaded with two different active agents (Tego Protect 5000, Evonik Tego Chemie GmbH or Dimethicone, Dow Corning Corp.) in varying quantities of the agents resulting in four different delivery systems ( Table 2 ). The delivery systems of materials A and B contained Tego Protect 5000, and materials C and E contained Dimethicone as the active agent. ST was modified by replacing 5% or 6% of the glass filler with the respective delivery system. At first the monomers were mixed, next all other ingredients but the glass filler and the Poly-Pore delivery systems were added and dissolved in the monomer mix. Then the respective Poly-Pore delivery system and finally the glass filler were homogeneously dispersed in the mixture.
Code | Product/properties | Batch | Company |
---|---|---|---|
Photoinitiator | α,α-Dimethoxy-α-phenylacetophenone | 0066162S | Ciba Specialities Chemical Inc., Basel, Switzerland |
Stabilizer | Pentaerythrityl-tetrakis[3-(3,5-di-tert-butyl-4-hydroxyphenyl)-propionate] | 26099IC3 | |
TTEGDMA | Tetraethyleneglycole dimethacrylate, standard monomer, functionality = 2, MW = 330 [g mol −1 ], good chemical and physical properties, very low viscosity (14 Pa s, 25 °C), diluting | J1620 | Cray Valley, Paris, France |
UV-stabilizer | 2-Hydroxy-4-methoxy-bezophenone | 411351/143302 | Fluka, Buchs, Switzerland |
UDMA | 7,7,9-Trimethyl-4,13-dioxo-3,14-dioxa-5,12-diaza-hexadecan-1,16-diol-dimethacrylate, standard monomer, functionality = 2, MW = 471 [g mol −1 ], flexible, tough, very good chemical resistance, medium viscosity (10,000 mPa s, 25 °C) | 330503057 | Rahn AG, Zürich, Switzerland |
Bis-GMA | Bis-GMA, standard monomer, functionality = 2, MW = 513 [g mol −1 ], rigid, very good chemical resistance, very high viscosity (4500 mPa s, 60 °C) | 2008218303 | |
CQ | d , l -Camphorquinone | 0148990002 | |
Amine | Ethyl-4-(dimethylamino)-benzoate | 310170 | |
Glass | Strontiumborosilicate glass (Glass G0 18-093, d 50 = 0.7 μm), silaned (3-methacryloyloxypropyltrimethoxy silane), D = 2.6 [g cm −3 ] | Lab14701 | Schott Electronic Packaging GmbH, Landshut, Germany |
Poly- | Poly-Pore, cross-linked polyallyl methacrylate, adsorber, hollow beads, diameter 20–40 μm | L07070303AB | AMCOL Health & beauty Solutions, Arlington Heights, IL, USA |
Tego | Tego Protect 5000, hydroxyfunctional polydimethylsiloxane, hydro- and oleophobic, D = 1.05 [g cm −3 ], surface tension γ L = 17.8 (0.7) mJ m −2 | ES57608918 | Evonik Tego Chemie GmbH, Essen, Germany |
Dimeth | Dimethicone 200/350 cst, polydimethylsiloxane, D = 0.965 [g cm −3 ], surface tension γ L = 16.6 (0.5) mJ m −2 | 4962250 | Dow Corning Corp., Midland, MI, USA |
Poly-Dimeth | Poly-Pore loaded with 80% and 85.7% Dimethicone, D = 1.0 [g cm −3 ] | Experimental products | University laboratory |
Poly-Tego | Poly-Pore loaded with 80% and 85.7% Tego Protect 5000, D = 1.0 [g cm −3 ] |
ST | A | B | C | E | Raw material |
---|---|---|---|---|---|
73.00 | 68.00 | 67.50 | 68.20 | 67.50 | Glass |
– | 5.00 | – | – | – | Poly-Tego 80% |
– | – | 6.00 | – | – | Poly-Tego 85.7% |
– | – | – | 5.00 | – | Poly-Dimeth 80% |
– | – | – | – | 6.00 | Poly-Dimeth 85.7% |
27.00 | 27.00 | 26.50 | 26.80 | 26.50 | Matrix |
0 | 4.00 | 5.14 | 4.00 | 5.14 | Active agent |
2.2
Preparation of the delivery systems (analog )
To obtain the four delivery systems ( Tables 1 and 2 ) 80 g or 85.7 g, respectively, Dimethicone or Tego Protect 5000 were dissolved in 400 g butanone (Lot 244238, Brenntag GmbH, Mülheim, Germany), then 20 g or 14.3 g, respectively, Poly-Pore hollow beads were added. The mixture was stirred for approximately 10 minutes to optimally wet the sorption particles. The mixture was slightly warmed to evaporate the solvent, and stirring was stopped when it became too stiff. Then it was put in a drying closet at 50 °C until a constant weight was obtained (generally 24 h). After this treatment, the loaded sorption materials, representing the delivery systems, appeared totally dry and powdery.
2.3
Specimen preparation and chewing simulation
Ten disks (thickness: 1 ± 0.1 mm, diameter: 10 ± 0.1 mm) of each material were made and cured for 40 s on each side (Spectrum 800, Dentsply deTrey GmbH, Constance, Germany). The output of the curing device was routinely checked (bluephase meter, Ivoclar Vivadent AG, Schaan, Liechtenstein). Irradiances of 931 ± 90 mW cm −2 were measured, and no significant decrease in the output was observed. The cured specimens were stored for 14 days in water at 37 °C and then one side was wet-polished (sterile water) with fine and superfine polishing discs (Super-Snap mini, Shofu Inc., Kyoto, Japan) for 1 min, with a grinding pressure of 40–50 g and 10,000 rpm (Endo-Mate TC, Nakanishi Inc., Tochigi, Japan). The degree of polymerization was not measured but the high irradiance of the light curing device ensures an optimal polymerization. Next the specimens were fixed with the polished sides up in the mounts of a chewing simulator (CS-4, SD Mechatronik GmbH, Feldkirchen-Westerham, Germany) with a MMA/PMMA resin (Technovit 4000, Heraeus Kulzer GmbH, Hanau, Germany). They were stressed for 650 cycles in water at 37 °C to simulate a 24 h chewing period according to the literature, which correlates the number of cycles to a realistic period of time . The 24 h were chosen to test the daily availability of the active agents. The test specimens were sliding under contact to a spherical antagonist (aluminum oxide, 5 mm diameter, Quick-Ohm Küpper & Co. GmbH, Wuppertal, Germany) for a linear distance of 8 mm (speed: 40 mm s −1 ) and a vertical load of 50 N resulting in a contact area of 0.6 mm × 8 mm and an average wear of 5.4 μm (±2.5 μm). This was repeated five times resulting in a worn area of 3 mm × 8 mm = 24 mm −2 to obtain sufficient surface to place a drop.
2.4
Contact angle and surface free energy (analog )
Contact angles were determined with the sessile drop method (Phoenix-Alpha contact angle goniometer, Surface Electro Optics – SEO – Corporation, Suwon-City, Korea). Immediately after placing the drop on the specimen’s surface ten pictures of each drop were photographed (magnification: 25-folds) in one second (CCD-camera: FireDragon, Toshiba-Teli Corporation, Tokyo, Japan) and analyzed (Image XP-software, Version 6.0 FW 012108, SEO Corporation). The drops were formed by a standardized dosing tube and, therefore, had always the same volume. Contact angle Θ was measured one time on each of the ten disks. SFE was calculated from the Θ measured after water storage. Apolar Lifshitz-van der Waals <SPAN role=presentation tabIndex=0 id=MathJax-Element-9-Frame class=MathJax style="POSITION: relative" data-mathml='γLLW’>γLWLγLLW
γ L LW
, polar Lewis acid <SPAN role=presentation tabIndex=0 id=MathJax-Element-10-Frame class=MathJax style="POSITION: relative" data-mathml='γL+’>γ+LγL+
γ L +
and Lewis base <SPAN role=presentation tabIndex=0 id=MathJax-Element-11-Frame class=MathJax style="POSITION: relative" data-mathml='γL−’>γ−LγL−
γ L −
terms of the test liquids were taken from the literature and the specimens’ total SFE γ S , their apolar term <SPAN role=presentation tabIndex=0 id=MathJax-Element-12-Frame class=MathJax style="POSITION: relative" data-mathml='γSLW’>γLWSγSLW
γ S LW
, polar term <SPAN role=presentation tabIndex=0 id=MathJax-Element-13-Frame class=MathJax style="POSITION: relative" data-mathml='γSAB’>γABSγSAB
γ S AB
, acid term <SPAN role=presentation tabIndex=0 id=MathJax-Element-14-Frame class=MathJax style="POSITION: relative" data-mathml='γS+’>γ+SγS+
γ S +
and base term <SPAN role=presentation tabIndex=0 id=MathJax-Element-15-Frame class=MathJax style="POSITION: relative" data-mathml='γS−’>γ−SγS−
γ S −
were calculated according to the equation of van Oss et al. by <SPAN role=presentation tabIndex=0 id=MathJax-Element-16-Frame class=MathJax style="POSITION: relative" data-mathml='(cosΘ+1)×γL=2((γSLWγLLW)+(γS−γL+)+(γS+γL−))’>(cosΘ+1)×γL=2((γLWSγLWL)−−−−−−−−−√+(γ−Sγ+L)−−−−−−√+(γ+Sγ−L)−−−−−−√)(cosΘ+1)×γL=2((γSLWγLLW)+(γS−γL+)+(γS+γL−))
( cos Θ + 1 ) × γ L = 2 ( ( γ S LW γ L LW ) + ( γ S − γ L + ) + ( γ S + γ L − ) )
and with <SPAN role=presentation tabIndex=0 id=MathJax-Element-17-Frame class=MathJax style="POSITION: relative" data-mathml='γSAB=2(γS+γS−)’>γABS=2(γ+Sγ−S)−−−−−−√γSAB=2(γS+γS−)
γ S AB = 2 ( γ S + γ S − )
(Image XP-software).
Prior to the chewing simulation the contact angles of specimens were measured with aqua dest. (laboratory product), glycerol (LOT II1097071, Thermo Scientific, Rockford, IL, USA), ethylene glycol (LOT 3289749, Carl Roth GmbH + Co. KG, Karlsruhe, Germany), and diiodomethane (LOT S82251, Sigma Aldrich GmbH, Taufkirchen, Germany).
Chewing simulation with 650 cycles was done and Θ was measured with aqua dest. After drying with oil-free air Θ was measured again with glycerol, ethylene glycol and diiodomethane. After each organic liquid’s measurement it was wiped off with alcohol and another 650 wear cycles were performed for each liquid to assure a clean surface.
2.5
Surface roughness R a (analog )
Surface roughness data of the materials prior to a chewing simulation test have already been published . After chewing simulation and contact angle measurement the specimens’ surface roughness R a was determined again (Surftest SJ-210 profilometer, diamond pick-up, tip radius 5 μm, load 4 mN, Mitutoyo Corporation, Kawasaki, Japan). Each specimen was measured five times at different distances and in different directions (evaluation length 4 mm, stylus speed 0.5 mm s −1 ) in the wear area. For each measurement the stylus was automatically moved five times forward and backward along the same path. The data were filtered with a cut-off ( Λ c ) of 0.8 mm (Gauss profile filter) following ISO 4288.
2.6
Statistical analysis
Means and standard deviations were calculated. Normal distribution was tested by Kolmogoroff–Smirnoff-test. Multivariate ANOVA and post hoc Scheffé’s test were calculated to find differences between the test materials’ SFEs. Univariate ANOVA and post hoc Scheffé’s test were calculated to find differences between the materials’ surface roughness and a t -test for unpaired samples was accomplished to find differences prior to and after chewing simulation. Statistics were calculated using IBM SPSS Statistics Version 21.0 (IBM Corporation, Somers, NY, USA). The statistical significance for all tests was set at p < 0.05.
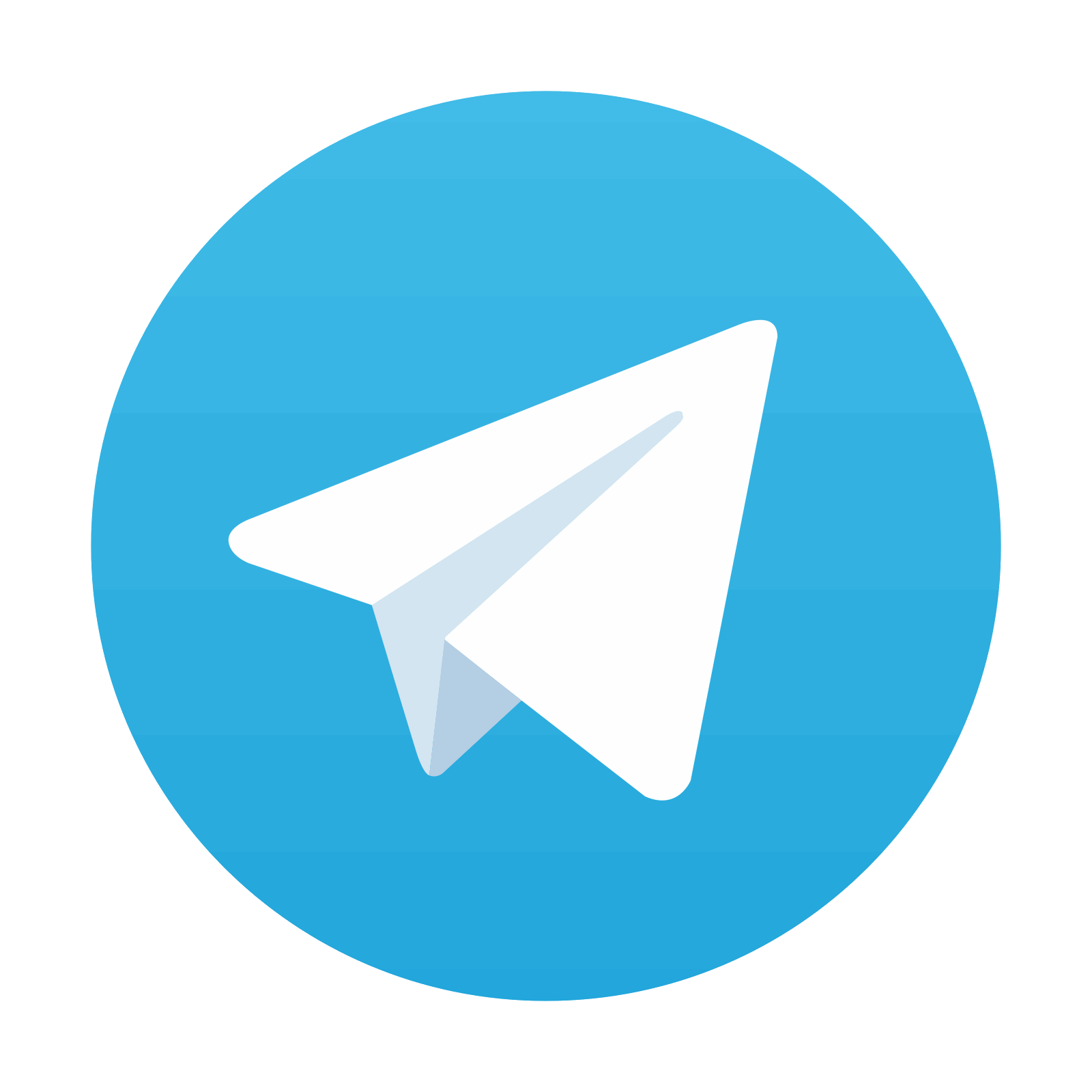
Stay updated, free dental videos. Join our Telegram channel

VIDEdental - Online dental courses
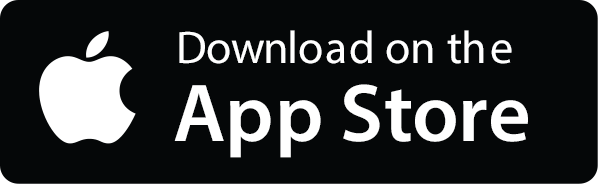
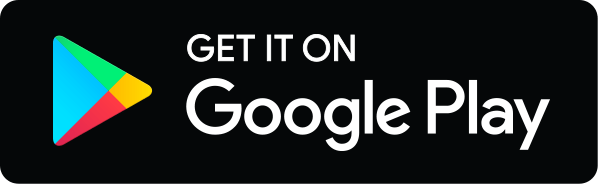